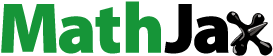
Abstract
Background
Iguratimod has been shown to promote bone formation and inhibit bone resorption in rheumatoid arthritis patients. We aimed to explore its effect on bone metabolism and vascular calcification (VC) in kidney transplant recipients (KTRs).
Methods
A post hoc analysis was conducted among the subjects in our previous randomized clinical trial (NCT02839941). Forty-three KTRs completing bone metabolism 52 weeks after enrollment were selected for this analysis, among whom 27 patients received VC examinations. In the iguratimod group, iguratimod (25 mg twice daily) was added adjuvant to the traditional triple regimen. At the 52-week follow-up, the following parameters were assessed: serum calcium, phosphorus, 25-hydroxyvitamin D, intact parathyroid hormone (iPTH), bone alkaline phosphatase (BALP), osteocalcin, type I collagen N-terminal peptide (NTx), type I collagen C-terminal peptide (CTx), bone mineral density (BMD) of the femoral neck and lumbar spine, coronary artery calcification (CAC) and thoracic aortic calcification (TAC). Bone metabolic and VC indices were compared between the two groups using the independent samples t test and Wilcoxon nonparametric test.
Results
At 52 weeks after enrollment, the iguratimod group had lower osteocalcin (p = 0.010), BALP (p = 0.015), NTx (p = 0.007), CTx (p = 0.012), CAC (p = 0.080) and TAC scores (p = 0.036) than the control group. There was no significant difference in serum calcium, phosphorus, 25-hydroxyvitamin D, iPTH and BMD between the groups. Iguratimod could reduce bone turnover markers (BTMs) at both high and low iPTH levels. The adverse effect of iguratimod was mild and tolerable.
Conclusion
Iguratimod is safe, can reduce BTMs and may could attenuate VC in the first year after KT.
Introduction
The kidneys play a critical role in maintaining the homeostasis of serum levels of calcium and phosphorus through interactions with hormones, including parathyroid hormone (PTH), 1,25(OH)2 vitamin D, and fibroblast growth factor 23. Derangement of calcium and phosphorus and disorders of bones, which are common in chronic kidney disease (CKD), can lead to chronic kidney disease-mineral and bone disorders (CKD-MBD), the triad of biochemical abnormalities, bone disease, and extraskeletal calcification. After successful kidney transplant (KT), although the serum calcium and phosphorus levels are gradually normalized as graft function continuously improves, bone formation and mineralization are still inhibited due to mild CKD status, persistent hyperparathyroidism, and the interference of bone cells by immunosuppressive agents, which can result in decreased bone density and a high risk of fracture [Citation1]. Sakaguchi et al. noted that up to 100% of the recipients showed histological evidence of bone dystrophy and osteopenia after KT [Citation2]. In a cohort of 36 kidney transplant recipients (KTRs), Evenpoel et al. found a relatively low rate of bone turnover at the time of biopsy and a further decline in subsequent years [Citation3]. Additionally, vascular calcification (VC) is one of the manifestations of CKD-MBD, which can lead to cardiovascular disease and increased mortality. Seyahi et al.reported the progression of coronary arterial calcification (CAC) in recipients 3 years after KT [Citation4]. Cianciolo et al. demonstrated that KT could not preclude the progress of VC but only delayed the process [Citation5].
There is no consensus on the optimal treatment of anti-bone resorption in KTRs. Bisphosphonates seem to have a beneficial effect on lumbar bone mass density (BMD), but they cannot significantly reduce fracture events and may increase the risk of low-turnover bone disease [Citation6]. A Cochrane review indicated that bisphosphonate therapy may reduce fractures and bone pain after kidney transplantation, but the low certainty of the evidence suggests that therapy may have little or no effect [Citation7]. Research regarding denosumab, which is a monoclonal antibody that inhibits receptor activators of nuclear factor κB ligand (RANKL) and osteoclast activity, is minimal in KTRs. A meta-analysis regarding denosumab suggested that it can increase bone density but is combined with subsequent hypocalcemia, which requires close monitoring [Citation8]. Further studies are needed to justify its utility in preventing fractures in KTRs.
Iguratimod is a novel small-molecule anti-rheumatic drug with significant efficacy and safety for active rheumatoid arthritis (RA), mainly through its action on T and B lymphocytes [Citation9]. Our team conducted a small clinical trial to investigate the clinical efficacy and safety of iguratimod in highly HLA-mismatched KTRs [Citation10]. In addition to the added effect on immunosuppression, we also found improved indices of bone turnover in KTRs receiving iguratimod therapy. Since iguratimod has been shown to inhibit osteoclast cells through the RANKL pathway [Citation11–13], it could be a valuable drug to reverse high bone resorption independent of parathyroid hormone and thus facilitate the rebalancing of bone modeling after KT. Here, we reported preliminary results regarding the effect of iguratimod on bone metabolism in a small random clinical trial setting.
Materials and methods
Patients and study design
Patients in this study were selected among the study population in a previous randomized, open-label clinical trial from the Kidney Transplantation Center of the First Affiliated Hospital of Nanjing Medical University. The clinical trial was approved by the Ethics Committee of the First Affiliated Hospital of Nanjing Medical University (2016-SR-029) and was registered at www.clinicaltrials.gov (NCT02839941). Written informed consent was obtained from all KTRs and recorded in the case report form files.
The population of the trial was described in detail in our previous paper. The inclusion criteria for patients were as follows [Citation1]: aged 18-65 years [Citation2], had received a primary kidney transplant 2 weeks before admission [Citation3], human leukocyte antigen (HLA) mismatch ≥4 [Citation4], serum creatinine (Scr) level < 1.5 times the upper limit of normal for 3 consecutive times and creatinine variation coefficient < 10% [Citation5], preoperative panel reactive antibody (PRA) < 10% [Citation6], immunosuppressant levels within the target concentration range before enrollment, and [Citation7] voluntary participation. The exclusion criteria were as follows: patients with delayed graft function, primary nonfunction, persistent acute rejection, preoperatively existing donor-specific antibodies, multiorgan transplantation, second kidney transplantation, or any major organ dysfunction, and pregnant or lactating women.
In the clinical trial, we screened 126 patients who received kidney transplants at our center from February 2018 to October 2019 and selected 60 patients based on the inclusion criteria. The patients were randomized into the iguratimod versus non-iguratimod groups at a 1:1 ratio. Three patients (1 in the iguratimod and 2 in the control group) withdrew voluntarily, one patient in the iguratimod group was excluded due to residential relocation, one patient in the control group withdrew on account of a severe surgical complication, and one patient in the control group died from pulmonary infection. Finally, 27 patients who received at least one dose of iguratimod were considered the modified intention-to-treat (mITT) population in the study arm, and 27 patients were present in the control arm. During the follow-up period, one patient in the iguratimod group withdrew in the first week due to intolerable gastrointestinal adverse events (AEs), which left 26 patients in the per-protocol (PP) population of the iguratimod group. In this study, patients were selected from the PP population with complete mineral and bone metabolic biochemical indicators, BMD, and VC examination at 52 weeks of follow-up (54 weeks after KT), including 20 patients in the iguratimod group and 23 patients in the control group.
Treatment
Patients in the iguratimod group received 25 mg oral iguratimod twice daily along with the conventional triple immunosuppressive protocol for 52 weeks, and the control group was only administered routine treatment. The initial immunosuppression protocol for each patient was prednisone, tacrolimus (0.05 to 0.1 mg/kg/day, twice daily reaching a target trough level of 10 ng/mL), and mycophenolate mofetil (MMF, 0.75 to 1.0 g twice daily). Methylprednisolone was intravenously administered at a dose of 500 mg/day on the day of surgery and until two days after KT. The dosage was reduced thereafter to 400 mg, 300 mg, 200 mg and then 80 mg over each subsequent day. This was followed by oral administration of 30 mg/day prednisone as maintenance therapy. Furthermore, 20 mg basiliximab (Simulect, Novartis, Switzerland) was intravenously administered 30 min before the surgery and on the fourth day after KT. Once the renal allograft function was stabilized, tacrolimus (target trough level of 6 to 10 ng/mL), MMF (0.5 to 1.0 g twice daily), and prednisone (25 mg daily initially and then tapering to 5 mg daily within three months) were administered as maintenance therapy. The patients intolerant to tacrolimus at the target dose were additionally given low-dose sirolimus.
Clinical data and laboratory assessments
The clinical and laboratory data of the recipients were collected at enrollment, and then general routine follow-up was conducted every four weeks. Fifty-two weeks after enrollment, clinical, laboratory and imaging data were collected, including mineral and bone metabolism indices and VC indices. Furthermore, bone metabolic indicators of the subjects within three months before KT were retrospectively collected.
Clinical data included sex, age, height, weight, body mass index (BMI), menstrual status, dialysis method and dialysis time, primary kidney disease, comorbidities (such as preoperative hypertension, or diabetes), smoking history (continuous or cumulative smoking for 6 months or longer), drinking history (daily alcohol intake of 3 units or more, the unit referring to the WHO fracture risk assessment system), history of total parathyroidectomy with forearm autotransplantation (TPTX + AT), use of immunosuppressants, use of calcium, calcitriol and cinacalcet, and glucocorticoid accumulation (calculated based on the dose of methylprednisolone).
Two transplant physicians followed up with all the patients and registered the clinical data into a database. Adverse events (AEs) were classified according to the National Cancer Institute (National Cancer Institute, NCI) Common Terminology Standard for Adverse Events (Common Terminology Criteria for Adverse Events, CTCAE), version 4.0. The estimated glomerular filtration rate (eGFR) was calculated using the MDRD formula: eGFR = 170 × Scr −0.999×age−0.176×BUN −0.170×Alb 0.318×(female × 0.762).
Bone metabolism biochemical markers included serum calcium, phosphorus, intact PTH (iPTH), 25-hydroxyvitamin D [25(OH)vitD], and bone turnover markers (BTMs), including bone alkaline phosphatase (BALP), osteocalcin, type I collagen N-terminal peptide (NTx), and type I collagen C-terminal peptide (CTx). Measurements were performed using Cobas e602 and e170 automatic electrochemical luminescence analyzers (Roche, Basel, Switzerland). Calcium levels were corrected using the following formula: Serum calcium = Raw serum calcium + (40-serum albumin)×0.02.
Evaluation of parathyroid hyperplasia, BMD, CAC, and thoracic aortic calcification (TAC)
One senior ultrasound physician with over five years of neck ultrasound experience performed parathyroid screen using a GE Logiq E9 ultrasound diagnostic device (GE Healthcare, Milwaukee, WI). Dual-energy X-ray absorptiometry (DEXA) (Discovery W S/N 85065; Hologic, 35 CrosbyDrive Bedford, USA) was used to determine the BMD of the femoral neck and lumbar spine. The bone density value was expressed in terms of area bone density in g/cm2. All patients were scanned using a 192-layer third-generation dual-source CT (SOMATOM Force, Siemens Healthineers, Forchheim, Germany). The scanning was controlled by prospective ECG gating, the tube voltage was 100 kV, the tube current was automatically regulated by the machine, and the frame rotation time was 250 ms. Patients were held up after inhalation during the scanning. The reconstruction layer thickness was 0.75 mm, the reconstruction convolution kernel was a partial soft algorithm (Bv36), and the visual field (FOV) was 25 × 25 cm. CAC and TAC were calculated by Agatston scoring of the Siemens SyngoVia workstation (SyngoVia VA30A, Siemens Healthcare, Forchheim, Germany). Each calcified coronary plaque was assessed by density and size integration. The coronary arteries, including the left main artery, left anterior descending branch, left spiral branch, and right coronary artery, were evaluated separately. The total calcification score was calculated. Imaging tests were performed using the same instruments.
Statistical analysis
The continuous data were presented as means ± standard deviation (SD). Independent sample t test was used for comparing normally distributed continuous variables and Wilcoxon nonparametric test was used for comparing non-normally distributed continuous variables between two groups. Categorical variables were expressed as the rate or composition ratio, and the chi-square test or Fisher’s exact probability method was applied for comparison. Two-sided p < 0.05 was defined as a significant difference. SPSS 25.0 software was used for data processing.
Result
Patient selection and demographic data
This study was a post hoc analysis of our previous clinical trial. The study cohort of the clinical trial is shown in , and the study cohort of this study is shown in . Forty-three patients (20 patients in the iguratimod group and 23 in the control group) with a complete panel of serum iPTH, calcium, phosphorus, 25(OH)vitD, BTMs, and BMD tests at 52 weeks after enrollment were identified as the current study cohort from all PP populations. All patients in the cohort had pretransplant records of calcium and phosphorus levels, and 32 patients (15 in the iguratimod group and 17 in the control group) had pretransplant data of iPTH, 25(OH)vitD, and BTMs. Pretransplant BMD, CAC and TAC were excluded here because they were available in only 5 patients in this cohort. The graft function of all patients remained stable during the study. The baseline characteristics were similar between the two groups ().
Table 1. Baseline characteristics of the study cohort.
Serum calcium, phosphorus, iPTH, BTMs, BMD, and vascular calcification at 52 weeks
After 52 weeks of enrollment, the patients’ serum iPTH, 25(OH)vitD, calcium, phosphorus, BTMs, and BMD were checked. VC evaluation was performed in 27 patients (14 in the iguratimod group and 13 in the control group). The two groups still showed similar serum iPTH, 25(OH)vitD, calcium, and phosphorus levels. BTMs were significantly decreased in the iguratimod group (p < 0.05). However, femoral neck and vertebral BMDs were even between the two groups after 52 weeks. For patients who received a vascular evaluation, the CAC (p = 0.080) and TAC scores(p = 0.036) were lower in the iguratimod group than the control group ().
Table 2. Serum iPTH,25(OH)vitD, Ca, Pi, BTMs, BMD, and vascular calcification score at 52 weeks.
Effects of iguratimod on mineral and bone metabolism indices across low and high iPTH levels
To investigate whether serum iPTH levels could influence the effect of iguratimod, we conducted a stratified analysis using the iPTH upper bound (88 pg/ml) as the threshold. Under this criterion, 18 patients (9 in the iguratimod group and 9 in the control group) stayed in the ‘iPTH low’ cluster, and the remaining 25 patients (11 in the iguratimod group and 14 in the control group) were in the ‘iPTH high’ cluster. We found that iguratimod could reduce BTMs independent of iPTH level. Femoral neck and vertebral BMDs were similar between the iguratimod and control groups in both clusters ().
Table 3. Mineral and bone metabolism indexes among iPTH low and high clusters.
Safety of iguratimod in KTRs
As reported in our previous clinical trial, no significant difference in liver function, renal function, or hemoglobin levels was observed between the two groups at any time point (). Twelve patients in the iguratimod group reported 18 AEs, and ten patients in the control group reported 14 AEs; this difference was not statistically significant. Gastrointestinal discomfort was the most frequent AE in the iguratimod group (8 in the iguratimod group vs. 0 in the control group), albeit transient and tolerable. No serious AE or AE-related death occurred during this trial.
Table 4. Patient reported adverse events during the clinical trial.
Discussion
Mineral and bone disorders (MBDs) are common after KT and may lead to bone fracture and vascular complications that are detrimental to the long-term quality of life (QoL) and survival of KTRs. Our results provided initial evidence showing that using iguratimod after KT could reduce the levels of BTMs and VC, and the effect on BTMs seemed irrelevant to the serum level of iPTH.
Several reasons may contribute to the risk of MBD after successful KT. In most cases, CKD after KT is only mild, yet it still imposes a high risk for MBD. In addition, preoperative hyperparathyroidism may persist in some patients, resulting in hypercalcemia and hypophosphatemia [Citation14,Citation15]. It has been shown that approximately 25-80% of recipients retain hyperparathyroidism one year after KT [Citation16]. As shown in our results, the iPTH level was above the normal range in over half of the patients at approximately one year after KT, albeit with normal graft function. Furthermore, bone formation and mineralization are suppressed by immunosuppressive agents, especially glucocorticoids, while osteoclast cells remain highly active, which contributes to a negative balance of bone remodeling [Citation17].
Iguratimod is a novel disease-modifying antirheumatic drug (DMARD) used as a first-line treatment for active rheumatoid arthritis (RA) in China and Japan. Ishikawa and colleagues found that more than half of RA patients treated with iguratimod could achieve radiologic stability or remission [Citation18]. The precise mechanisms of iguratimod were unclear. Some studies have shown that it modulates multiple immune cells and nonimmune cells through the NF-κB pathway. In a recent study, Li Changhong et al. showed that iguratimod inhibits osteoclastogenesis via RANKL and the tumor necrosis factor (TNF-
) pathway [Citation19]. Miyama A and colleagues have also demonstrated the bone protective effect of iguratimod on glucocorticoid-induced osteoporosis [Citation13]. Localized bone loss, as occurring in RA, and systemic osteoporosis, as seen in KT patients, share common pathophysiologic pathways. Unbalanced bone resorption and formation result in the net loss of bone density. After KT, osteoblast cells are inhibited by multiple factors, including immunosuppressive drugs, high levels of serum PTH, and deficiency of calcitriol, while osteoclastogenesis is active for similar reasons. For example, glucocorticoids can directly act on osteoblasts to upregulate the expression of RANKL, which binds to RANK on the surface of osteoclasts to facilitate their differentiation and activation [Citation20]. Our results showed a significant decline in bone resorption indices in the iguratimod group, consistent with the mechanism of iguratimod on osteoclast cells.
Iguratimod may also activate osteoblasts directly. Kuriyama et al. found that iguratimod can promote the differentiation of pro-osteoblast (MC3T3-E1) and stromal cell lines in vitro and facilitate the osteogenesis induced by bone morphogenetic protein-2 (BMP2) in vivo [Citation21]. BMP2 modulates bone formation by activating the expression of sterol (Osx) through the fusion flow transcription factor Dlx5. The p38-MAPK pathway can interact with the BMP2-Smad pathway to phosphorylate Osx [Citation22]. Iguratimod can promote osteoblast differentiation by increasing the expression of both Osx and Dlx5 and activating the p38-MAPK pathway. In addition, NF-κB mediates osteoblast destruction by downregulating the expression and phosphorylation of the BMP-Smad1 signaling pathway, while iguratimod prevents osteoblast destruction by inhibiting the NF-κB pathway [Citation23]. Clinical studies have also found that the combination of methotrexate and iguratimod could more effectively stimulate bone formation [Citation24]. However, our results showed that bone formation was also decreased under iguratimod treatment, and there was no net bone density increase after 52 weeks of iguratimod treatment. In the setting of KTRs, the reasons for osteoporosis even after renal function recovery are multiple, and there are unfavorable factors for bone metabolism normalization, including continuous steroid use, immunosuppressants, and dysfunction of the parathyroid axis. In addition, the relatively short observation time and small population may also result in such negative outcomes. However, considering the concurrent decline in bone resorption, we postulate that bone turnover might be rebalanced at a new level during iguratimod treatment. Therefore, further studies should focus on the feedback loop that regulates the homeostasis of bone formation, and a larger cohort and longer follow-up are imperative. In addition, we found that in both low and high iPTH clusters, BTMs showed a similar trend, indicating that the effect of iguratimod on bone mineral turnover was independent of serum iPTH level.
Vascular calcification is the major risk factor for cardiovascular complications in CKD patients as well as in KTRs. Although the complicated mechanisms underlying VC are still not fully understood, this event is strongly related to CKD-MBD. Many studies have demonstrated that KT cannot preclude the progress of VC but only delays the process. The avoidance of cardiovascular complications is positively correlated with the long-term survival of KTRs. Our results indicated the potential vascular protective role of iguratimod, which deserves further attention. The calcification of vascular lesions is partly regulated by the osteoprotegerin-RANKL pathway, the exact target of iguratimod [Citation25]. Iguratimod may also act on vascular endothelial cells and vascular smooth muscle cells to muffle the inflammation caused by multiple factors via the NF-κB pathway. Moreover, our unpublished data revealed that iguratimod could act on macrophages to prevent the inflammatory cascade in vitro. Above all, the effect of iguratimod on bone and VC combined with its immune modulating effect renders it a very promising agent to improve long-term survival and QoL in the KT population.
To our knowledge, this study is the only clinical observation focusing on the effect of iguratimod on MBD in the setting of KT. These results may reveal new ideas for the treatment of CKD-MBD in the KT population. However, there are some limitations in our study. First, the sample size was relatively small. Moreover, our trial was not initially designed to show the effects of iguratimod on bones. Therefore, nearly one-third of our patients lacked preoperative bone metabolism-related data. Second, the follow-up time was short. Vascular calcification and bone density changes might not be present in such a short interval. Third, bone biopsy was not applied in the current study. All these limitations warrant caution in interpreting our results.
In conclusion, the current analysis showed that iguratimod could reduce BTMs independent of serum iPTH levels and may could attenuate VC in the first year after KT. Further studies should be performed to verify our results.
Statement of Ethics
This study was approved by the Ethics Committee of the First Affiliated Hospital of Nanjing Medical University (2016-SR-029) and has been registered at www.clinicaltrials.gov (NCT02839941). Written informed consent was obtained from all KTRs.
Disclosure statement
The authors report no conflict of interest.
Data availability
Reasonable requests for data will be accommodated by contacting the corresponding author.
Additional information
Funding
References
- Naylor KL, Li AH, Lam NN, et al. Fracture risk in kidney transplant recipients: a systematic review. Transplantation. 2013;95(12):1–8. doi: 10.1097/TP.0b013e31828eead8.
- Sakaguchi Y, Hamano T, Wada A, et al. Magnesium and risk of hip fracture among patients undergoing hemodialysis. J Am Soc Nephrol. 2018;29(3):991–999. doi: 10.1681/ASN.2017080849.
- Evenepoel P, Claes K, Meijers B, et al. Natural history of mineral metabolism, bone turnover and bone mineral density in de novo renal transplant recipients treated with a steroid minimization immunosuppressive protocol. Nephrol Dial Transplant. 2020;35(4):697–705. doi: 10.1093/ndt/gfy306.
- Seyahi N, Cebi D, Altiparmak MR, et al. Progression of coronary artery calcification in renal transplant recipients. Nephrol Dial Transplant. 2012;27(5):2101–2107. doi: 10.1093/ndt/gfr558.
- Cianciolo G, Capelli I, Angelini ML, et al. Importance of vascular calcification in kidney transplant recipients. Am J Nephrol. 2014;39(5):418–426. doi: 10.1159/000362492.
- Kan SL, Ning GZ, Chen LX, et al. Efficacy and safety of bisphosphonates for low bone mineral density after kidney transplantation: a meta-analysis. Medicine (Baltimore). 2016;95(5):e2679. doi: 10.1097/MD.0000000000002679.
- Palmer SC, Chung EY, McGregor DO, et al. Interventions for preventing bone disease in kidney transplant recipients. Cochrane Database Syst Rev. 2019;10(10):CD005015.
- Thongprayoon C, Acharya P, Aeddula NR, et al. Effects of denosumab on bone metabolism and bone mineral density in kidney transplant patients: a systematic review and meta-analysis. Arch Osteoporos. 2019;14(1):35. doi: 10.1007/s11657-019-0587-0.
- Jiang H, Gao H, Wang Q, et al. Molecular mechanisms and clinical application of iguratimod: a review. Biomed Pharmacother. 2020;122:109704. doi: 10.1016/j.biopha.2019.109704.
- Tao J, Sun L, Wang Z, et al. Efficacy and safety of iguratimod supplement to the standard immunosuppressive regimen in highly mismatched renal transplant recipients: a pilot study. Front Immunol. 2021;12:738392. doi: 10.3389/fimmu.2021.738392.
- Wang X, Ma C, Li P, et al. Effects of iguratimod on the levels of circulating regulators of bone remodeling and bone remodeling markers in patients with rheumatoid arthritis. Clin Rheumatol. 2017;36(6):1369–1377. doi: 10.1007/s10067-017-3668-8.
- Gan K, Yang L, Xu L, et al. Iguratimod (T-614) suppresses RANKL-induced osteoclast differentiation and migration in RAW264.7 cells via NF-kappaB and MAPK pathways. Int Immunopharmacol. 2016;35:294–300. doi: 10.1016/j.intimp.2016.03.038.
- Miyama A, Ebina K, Hirao M, et al. Effects of iguratimod on glucocorticoid-induced disorder of bone metabolism in vitro. J Bone Miner Metab. 2021;39(4):639–648. doi: 10.1007/s00774-021-01206-5.
- Evenepoel P, Meijers BK, de Jonge H, et al. Recovery of hyperphosphatoninism and renal phosphorus wasting one year after successful renal transplantation. Clin J Am Soc Nephrol. 2008;3(6):1829–1836. doi: 10.2215/CJN.01310308.
- Evenepoel P. Recovery versus persistence of disordered mineral metabolism in kidney transplant recipients. Semin Nephrol. 2013;33(2):191–203. doi: 10.1016/j.semnephrol.2012.12.019.
- Wolf M, Weir MR, Kopyt N, et al. A prospective cohort study of mineral metabolism after kidney transplantation. Transplantation. 2016;100(1):184–193. doi: 10.1097/TP.0000000000000823.
- Farmer CK, Hampson G, Abbs IC, et al. Late low-dose steroid withdrawal in renal transplant recipients increases bone formation and bone mineral density. Am J Transplant. 2006;6(12):2929–2936. doi: 10.1111/j.1600-6143.2006.01557.x.
- Ishikawa K, Ishikawa J. Iguratimod, a synthetic disease modifying anti-rheumatic drug inhibiting the activation of NF-kappaB and production of RANKL: its efficacy, radiographic changes, safety and predictors over two years’ treatment for Japanese rheumatoid arthritis patients. Mod Rheumatol. 2019;29(3):418–429. doi: 10.1080/14397595.2018.1481565.
- Li CH, Ma ZZ, Jian LL, et al. Iguratimod inhibits osteoclastogenesis by modulating the RANKL and TNF-alpha signaling pathways. Int Immunopharmacol. 2021;90:107219. doi: 10.1016/j.intimp.2020.107219.
- Canalis E, Mazziotti G, Giustina A, et al. Glucocorticoid-induced osteoporosis: pathophysiology and therapy. Osteoporos Int. 2007;18(10):1319–1328. doi: 10.1007/s00198-007-0394-0.
- Kuriyama K, Higuchi C, Tanaka K, et al. A novel anti-rheumatic drug, T-614, stimulates osteoblastic differentiation in vitro and bone morphogenetic protein-2-induced bone formation in vivo. Biochem Biophys Res Commun. 2002;299(5):903–909. doi: 10.1016/s0006-291x(02)02754-7.
- Ulsamer A, Ortuno MJ, Ruiz S, et al. BMP-2 induces osterix expression through up-regulation of Dlx5 and its phosphorylation by p38. J Biol Chem. 2008;283(7):3816–3826. doi: 10.1074/jbc.M704724200.
- Song J, Liu H, Zhu Q, et al. T-614 promotes osteoblastic cell differentiation by increasing Dlx5 expression and regulating the activation of p38 and NF-kappaB. Biomed Res Int. 2018;2018:4901591–4901598. doi: 10.1155/2018/4901591.
- Ishiguro N, Yamamoto K, Katayama K, et al. Concomitant iguratimod therapy in patients with active rheumatoid arthritis despite stable doses of methotrexate: a randomized, double-blind, placebo-controlled trial. Mod Rheumatol. 2013;23(3):430–439. doi: 10.3109/s10165-012-0724-8.
- Panizo S, Cardus A, Encinas M, et al. RANKL increases vascular smooth muscle cell calcification through a RANK-BMP4-dependent pathway. Circ Res. 2009;104(9):1041–1048. doi: 10.1161/CIRCRESAHA.108.189001.