Abstract
Aim
This study explores the possible therapeutic role of rats and mice bone marrow-derived mesenchymal stem cells (BM-MSCs) on renal damage and toxicity brought on by carbon tetrachloride (CCl4) in Wistar rats.
Methods
Following an intraperitoneal injection of CCl4 (0.5 mL/kg b.w. twice weekly) for eight weeks, male Wistar rats were intravenously treated with rats and mice BM-MSCs (1 × 106 cells in 0.2 mL Dulbecco’s Modified Eagle Medium (DMEM)/rat/week) a week for four weeks. Kidney functions were evaluated and kidney samples were examined using hematoxylin and eosin (H&E), Masson’s trichrome (MT) staining techniques, and electron microscopy analysis. Kidney cyclooxygenase-2 (COX-2), protein 53 (p53), and tumor necrosis factor-α (TNF-α) were detected by immunohistochemical staining techniques. Additionally, bioindicators of oxidative stress and antioxidant defense systems were identified in kidney tissue.
Results
In CCl4-injected rats, serum creatinine, urea, and uric acid levels significantly increased, as did renal lipid peroxidation (LPO), while superoxide dismutase, glutathione peroxidase (GPx), glutathione (GSH) transferase, and GSH levels significantly dropped in the kidneys. Histologically, the kidneys displayed a wide range of structural abnormalities, such as glomerular shrinkage, tubular dilations, inflammatory leukocytic infiltration, fibroblast proliferation, and elevated collagen content. Inflammatory cytokines like COX-2 and TNF-α as well as the pro-apoptotic mediator p53 were considerably upregulated. Treatment of BM-MSCs from mice and rats with CCl4-injected rats considerably reduced the previously noted abnormalities.
Conclusions
By boosting antioxidant defense and reducing apoptosis and inflammation, BM-MSCs from mice and rats were able to enhance kidney function and histological integrity in rats that had received CCl4 injections.
Introduction
CCl4, also known as tetrachloromethane, Halon-104, and Refrigerant-10 [Citation1,Citation2], is a clear, colorless liquid that is volatile, heavy, and nonflammable. It is a potent environmental hepatotoxin and nephrotoxin that causes steatosis, necrosis, and cirrhosis [Citation3]. Although the exact mechanism of CCl4-induced renal damage is unknown, it is thought to be similar to that of liver damage [Citation4,Citation5]. CCl4 toxicity is due to its reactive and unstable metabolites [Citation6]. The kidney’s proximal tubule cells’ endoplasmic reticulum is where the CYP2E1 enzymes transform CCl4 into the extremely reactive trichloro methyl (CCl3) and trichloro methyl peroxide radicals (CCl3O2) [Citation7–9]. These radicals can attach to macromolecules (proteins and lipids) and extract hydrogen from unsaturated fatty acids, causing a lipid peroxidation (LPO) chain reaction that damages cell membranes and organelles in terms of both morphology and functionality [Citation10,Citation11] CCl4 produces oxidative stress by inducing reactive free radicals, such as hydrogen peroxides, hydroxyl radicals, superoxides, peroxynitrite, and a range of other radicals [Citation12]. The nephrotoxic effects of CCl4 are linked to free radical production, which causes LPO and membrane breakdown [Citation13]. Furthermore, CCl4 exposure causes nephritis, nephrosis, and renal failure, all of which are common kidney illnesses. One of the principal causes of death in CCl4-exposed patients is kidney failure [Citation14]. Oxidative damage has substantial consequences, which are often manifested by the increased activity of oxygen detoxification enzymes. Notably, antioxidants may counteract the detrimental effects of free radicals and oxidative stress [Citation15]. CCl4 induces increased LPO products and decreased activity of enzymes that protect the LPO in the kidney [Citation3,Citation16,Citation17].
CCl4 increases the activity of essential apoptotic enzymes and the generation of well-known inflammatory cytokines, such as TNF-α [Citation18,Citation19]. It causes reactive oxygen species (ROS) and p53 to be released, destroying renal tubular cells. The fibrotic process is then aided, directly or indirectly, by ROS-induced additional inflammation. The reemergence of fibrosis and inflammation may further boost ROS production [Citation20].
MSCs, a type of multipotent non-hematopoietic cell, may be formed from different human body parts, including BM, umbilical cord blood, and adipose tissue [Citation1,Citation21]. MSCs are a type of adult stem cell that can self-replicate and differentiate in different directions, allowing them to specialize in tissue-specific cells while preserving tissue self-renewal and physiological activities [Citation22,Citation23]. Numerous animal model studies have yielded positive results [Citation24–27]. MSCs have anti-inflammatory, antioxidant, angiogenic, and anti-fibrotic properties, and they are mostly used to replace damaged cells or tissues; however, trophic factors, such as chemokines, cytokines, growth factors, and exosomes, dictate their main roles [Citation28,Citation29]. The fibrocyte appears to be a modest contributing cell type, at least in the kidney and liver, although reports suggest that a subpopulation of BM-derived cells known as fibrocytes directly lays down a fibrous matrix [Citation30–33].
As an ‘off-the-shelf’ product, an allogeneic source enables large-scale MSC therapy batch production, donor variability elimination (e.g., age and disease pathophysiology), and rapid accessibility for emergency care [Citation34,Citation35]. Although allogeneic MSC therapies have the potential to be a new class of curative medications, practical issues, such as immune rejection, donor cell availability, and efficient delivery, remain to be resolved [Citation36]. Heterogenic MSCs from different sources share morphological, regenerative, and immunomodulatory characteristics [Citation37,Citation38]. Heterogonous MSCs produced from monkey BM can cure liver fibrosis in a mouse model, and the paracrine actions of MSC may be crucial in the treatment of hepatic fibrosis [Citation39].
So, this research was done to evaluate and contrast the effects of rats and mice BM-MSCs on CCl4-induced kidney injury, fibrosis, oxidative stress, inflammation, and apoptosis in Wistar male rats.
Materials and methods
BM-MSCs culture protocol
Materials
Fetal bovine serum (FBS), Dulbecco’s Modified Eagle Medium (DMEM), and 0.25% Trypsin/EDTA solution were bought from Lonza in Belgium. We purchased sodium selenite, sodium phosphate dibasic (Na2HPO4), and sodium phosphate monobasic (NaH2PO4) from LOBA, Chemie, India. Greiner Bio-one provided culture flasks and culture supplies (Frickenhausen, Germany).
Isolation and culture of rats and mice BM-MSCs
For isolation of BM-MSCs, eight male Wistar rats (Rattus norvegicus) (4-week old) and eight male albino mice (Mus musculus) (4 weeks old) were used. Animals were decapitated by cervical dislocation under short-term ethyl ether inhalation anesthesia and the fur was sprayed with 70% (vol/vol) ethyl alcohol to disinfect the body surface. For harvesting bone marrow, the tibiae and femurs were rapidly dissected out. The muscles connected to the tibiae and femurs were separated during dissection. The muscles, ligaments, and tendons in the tibias, femurs, and humeri were carefully dissected out using medical scissors and a knife after the entire skin was taken from the limbs. Bones were transferred to sterile gauze, and any remaining soft tissue around the bones was scrubbed away. Once the bones had been moved to a sterile culture dish, bone marrow cells were extracted by flushing the dish with DEMEM and then being collected in a 15 mL Falcon tube. The cells were moved and separated during a 5-min period of centrifugation at 3000 rpm. After decanting the supernatant DEMEM supplemented with 15% FBS, 0.36% sodium hydrogen carbonate, and 1% penicillin-streptomycin solution, the cell pellet was immediately washed with phosphate buffer saline (PBS) and suspended in full DEMEM. Using a hemocytometer and a 100X magnification, live and dead cells were counted to determine cell viability (the proportion of viable cells to all other cells) after staining with trypan blue solution (0.2%) [Citation40,Citation41]. 2.5 × 106 cells were seeded at a cell density of 1 × 106 cells/cm2 area in T-25 cm2 sterile Greiner cell culture flasks with canted neck, and then placed in a 5 % CO2-humidified incubator (Biobase, Model: BJPX-C50; South Gongye Road, Jinan, Shandong Province, China) at 37 °C. After 4 d of incubation, floating and nonadherent cells, as well as dead cells, were removed. The isolated MSC meets the criteria according to the Mesenchymal and Tissue Stem Cell Committee of the International Society for Cellular Therapy.
Animals
Adult male Wister rats weighing 130 ± 10 g were kept under conventional laboratory conditions (Egyptian Organization for Biological Products and Vaccines (VACSERA), Helwan, Egypt) at 22 °C, 55% humidity, and a 12/12 (light/dark) cycle. The animals were given free access to tap water and a conventional pellet diet. Every animal’s procedures followed and guidelines set forth by the Scientific Animal Ethics Committee of the Faculty of Science at Beni-Suef University in Egypt for the Use and Care of Animals (Ethical Approval Number BSU/FS/ 2019-73). Every attempt was made to lessen animal suffering and the number of animals involved.
Experimental design
Four groups, each of six animals, were included in the experiment. The animals in the first group received olive oil administered intraperitoneally (i.p.) twice weekly for eight weeks as a normal control, while those in the second group received CCl4 administered i.p. at a dose of 0.5 mL/kg [Citation42] twice/week for eight weeks. The third and fourth groups received CCl4 like the second group and then treated with rat BM-MSCs and mouse BM-MSCs (1 × 106 cell/rat/week) by intravenous injection into lateral tail vein [Citation43] for four weeks. For the duration of the four-week course of treatment, an equivalent volume of DMEM (0.2 mL) was injected weekly into a lateral tail vein of rats of the first and second groups during the last 4 weeks of the experiment (Scheme 1). Blood samples were collected to assess biochemical parameters and were stored at −70 °C until needed. The animals’ body weights for each group were measured. Kidney tissue samples were collected and processed for histological examination.
Biochemical tests of kidney functions
According to Schultz [Citation44], serum creatinine and uric acid levels were measured using reagent kits purchased from Spinreact Company (S.A/S.A.U. Ctra. Santa Coloma, 7 E-17176 Sant ESTEVE DE BAS (GI), Spain). Following Kaplan’s method [Citation45], urea was determined in serum samples using kits obtained from the Diamond Company (23 EL-Montazh St., Cairo, Egypt).
Oxidant and anti-oxidant enzymes of kidney
Kidney LPO was determined following Yagi’s method [Citation46]. Malondialdehyde (MDA), which is produced during the breakdown of polyunsaturated fatty acids, can be used to accurately determine the extent of LPO. The reaction of LPO products with thiobarbituric acid is used to determine their concentration.
Glutathione peroxidase (GPx) activity was assessed using the technique described by Matkovics et al. [Citation47]. This activity was measured in nanomoles of glutathione (GSH) oxidized per minute per milligram of protein. According to Beutler et al. [Citation48], GSH was tested using a spectrophotometric technique utilizing 5,5-dithiobis-2-nitrobenzoic acid (DTNB). According to Mannervik and Guthenberg [Citation49], glutathione transferase (GST) activity was measured photometrically in the presence of reduced GSH and hazardous toxin 1-chloro-2,4 dinitrobenzene (CDNB). Superoxidase (SOD) activity in the kidney tissue was measured following the technique described by Marklund and Marklund [Citation50].
Histopathological observations
The kidney tissue specimens were preserved in a buffered formaldehyde solution (10 % w/v) for histological analysis. The preserved tissues were then dried with ethanol solutions of varying concentrations (50%–100% v/v), embedded in paraffin, cut into 4–5 m thick slices, stained with H&E, and examined under a light microscope using MT. The amount of CCl4-induced kidney injury was assessed using morphological alterations in the H&E and MT-stained sections. For histological investigations, a scoring system was applied [Citation51,Citation52].
Kidney sections were examined to determine the histological lesions. In three randomly selected fields of each section (×100), lesions were graded as absent (0), mild (I), moderate (II), and severe (III) for changes, 0%, less than 30%, 30–50%, and more than 50%, respectively [Citation53]. The graded histopathological lesions represent the different changes such as dilated congested glomeruli, collapsed glomeruli, dilated tubules, pyknotic nuclei, monocellular leukocytic inflammation, and interstitial hemorrhages.
Immunohistochemistry analysis of kidney toxicity
Sections (5–6 µm thick) were deparaffinized with xylene before being rehydrated in a descending series of ethanol. COX-2, p53, and TNF-α were detected by immunohistochemistry in all rats’ kidney tissue using an avidin-biotin immunoperoxidase method [Citation54], according to the manufacturer’s instructions. The primary antibodies for COX-2, p53, and TNF-α were obtained from Bio Genex (Santa Cruz, CA). The sections were incubated with streptavidin horseradish peroxidase (Dako-K0690; Dako Universal LSAB Kit, Bangkok, Thailand), biotinylated secondary antibody (Dako-K0690), and 3, 3′-diaminobenzidine tetrahydrochloride (Sigma-D5905; Sigma-Aldrich Company Ltd., Gillingham, UK) substrate for immunolabelling for 30 min on each. Finally, the nuclei underwent I staining with Harris hematoxylin stain, (ii) dehydration in graded alcohol, (iii) clearing in xylene, and (iv) mounting in DPX. The binding of antibodies was examined using high-powered light microscopy.
Image J analysis staining intensity evaluation
Using the ‘Leica Quin500C’ image analyzer computer system, the percentage of positive Masson’s trichrome stain (MT), TNF-α, p53, and COX-2 immunoreactive cells in the kidney was estimated (Leica Imaging System Ltd., Cambridge, England). Five non-overlapping H&E and immune-stained fields from five distinct sections were examined for each animal. Data are represented as mean ± standard error.
Ultrastructural examining
For transmission electron microscope (TEM) analysis, tiny renal cortex, and medulla specimens (1 mm3) were fixed in 3% glutaraldehyde-formaldehyde at 4 °C for 18–24 h, followed by a post-fixation in 1% osmium tetroxide for 1 h at 4 °C, drying in an evaluated arrangement of ethanol, and embedding in Araldite. Utilizing an LEICA Ultramicrotome, semithin sections of one micrometer were cut, mounted on glass slides, and toluidine blue stained. At that time, ultra-thin sections were cut, viewed with a light microscope to identify the region of interest, and then stained with uranyl acetate and lead citrate and analyzed under a Joel CX 100 TEM with a 60 kV accelerating voltage [Citation55].
Data statistical analysis
The collected data were shown as mean standard error. One-way analysis of variance and Duncan’s multiple range test were performed for the statistical analysis using the Statistical Package of Social Sciences (SPSS) programme version 21 (SPSS, Cary, NC) [Citation56,Citation57]. At p < 0.05, a difference between groups was deemed significant.
Results
Body weight
The rats’ mean body weight before receiving a CCl4 injection varied in groups between 129.18 and 131.17 g. At the end of the experiment, there was a significant decrease (p < 0.05) in body weight and body weight gain in CCl4-njected group when compared to normal group. The treatment of CCl4-njected rats with rats and mice BM-MSCs produced a noticeable increase in the body weight at the end of the experiment. Body weight gain significantly (p < 0.05) increased as a result of treatment with rats and mice BM-MSCs; rats’ BM-MSCs was more effective ().
Table 1. Body weight and body weight gain in the studied groups.
Biochemical effects
The biological effects on blood markers measuring kidney function are listed in . Comparing the groups given treatment with rats and mice BM-MSCs to the CCl4-injected control group, the levels of creatinine, urea, and uric acid considerably dropped.
Table 2. Serum creatinine level, urea, and uric acid levels in different experimental groups.
Effect on antioxidant defense system and oxidative stress
CCl4 injection significantly raised LPO in comparison to the control group. The CCl4-induced elevation in LPO levels was dramatically decreased when rats that had received CCl4 injections were given treatment with both mice and rats BM-MSCs ().
Table 3. SOD activity and MDA in kidney of different experimental groups.
Comparing the CCl4-injected rats to the control group, the GSH content and GST activity levels drastically decreased. In contrast to the control group, treatment with rats BM-MSCs considerably averted a decline in GSH and GST levels, whereas treatment with mice BM-MSCs did not significantly change this impact. In contrast to the control group, the rats given CCl4 injections had considerably decreased SOD activity. Furthermore, treatment with rats BM-MSCs and treatment with mice BM-MSCs dramatically halted the decline in SOD activity relative to the control group. However, GPx activity was significantly lower after CCl4 administration compared to the control group. Treatment with rats BM-MSCs and mice BM-MSCs stopped the decline in these enzymes’ activity considerably ( and ).
Table 4. GSH content and GST& GPx activities in kidney of different experimental groups.
Histopathological examination of kidneys
Light microscopic examinations revealed that the proximal, distal, and collecting tubules (CTs) in the kidneys of the control groups were all normal (), as well as tubule cells of the renal medulla (). CCl4 administration resulted in significant degenerative changes in kidney tissues, including completely destroyed glomeruli (), dilated CTs, fibroblastic proliferation, congested glomeruli, congested blood vessels (), monocellular leukocyte inflammation in tubules (), intertubular fibrosis, and interstitial hemorrhages (). In addition, MT reaction revealed an increase in collagen fibers in the interstitium around the renal tubules, Bowman’s capsule, and blood vessels () compared to the normal control group (). Treatment with rats BM-MSCs and mice BM-MSCs protected the kidneys from CCl4-induced damage, resulting in improved histological structure of glomeruli and tubules, compared to the CCl4 group (). Treatment with rats BM-MSCs and mice BM-MSCs also prevented the decrease in MT stain (, respectively), which showed scant collagen fibers, compared to the CCl4 group.
Figure 1. Photomicrographs of kidney section of control group showing (a,b): normal architecture of glomerulus (G) proximal tubule (P), distal tubule (D), and collecting tubule (CT) and tubular cells of renal medulla (Scale bar of a = 50 µm, Scale bar of b = 200 µm). Kidney section of CCl4-injected group showing (c,d): completely demolished glomeruli (G), dilated collecting tubules, collapsed glomeruli (blue arrow), congested glomeruli (green arrow), and congested blood vessels (arrow) (Scale bar of c = 200 µm and Scale bar of d = 100 µm). Kidney section of CCl4 group showing (e,f) monocellular leukocyte inflammation (IF), pyknotic nuclei (arrow), karyomegaly (curved arrow), intertubular fibrosis, and interstitial hemorrhages (H) (Scale bar of e and f = 50 µm).
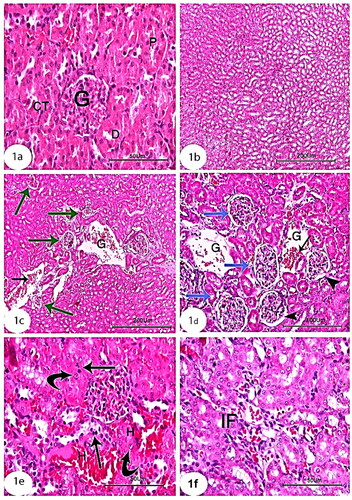
Figure 2. Photomicrographs of kidney section of treated groups (a,b): CCl4 + rats BM-MSCs and (c,d): CCl4 + mice BM-MSCs showing improvement of histological structure of glomeruli and renal tubules. (H&E stain; Scale bar of a and b = 100 µm, Scale bar of c and d = 200 µm).
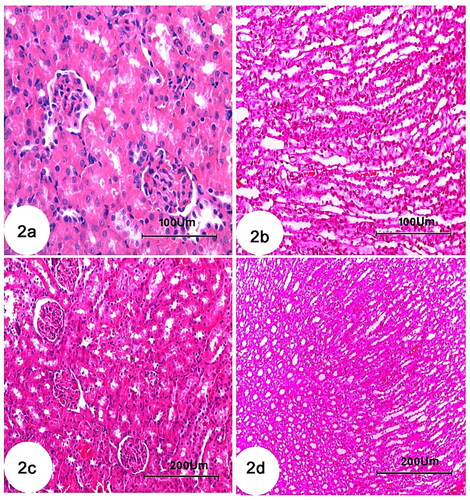
Figure 3. Photomicrographs of kidney section of rats showing (a): Control group with minimal amount of collagen fibers. (b): Group CCl4 showing marked increase of collagen fibers in the interstitium around the renal tubules, Bowman’s capsule and blood vessels. (c): CCl4 + rats BM-MSC and (d): CCl4 + mice BM-MSCs, respectively, showing scantly collagen fibers compared with group CCl4. (Masson’s trichrome stain; Scale bar of a–d = 100 µm).
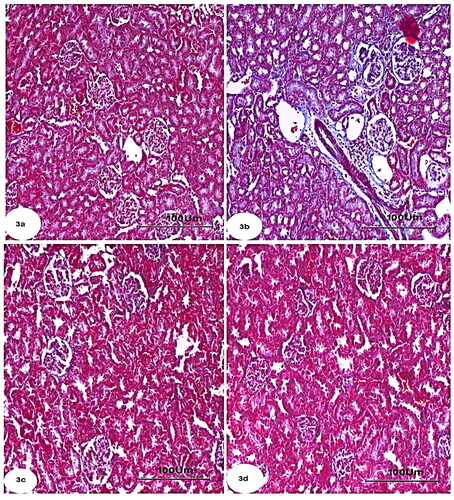
Different groups revealed histopathological scores lesions as shown in . No histological lesions observed in normal control kidney section as represented by zero score. CCl4-treated rats demonstrated different grades of histopathological alterations scores of lung ranging from grade III to grade 0. The treatments of CCl4-injected group with rats’ BM-MSCs and mice BM-MSCs revealed marked improvements including dilated congested glomeruli, collapsed glomeruli, dilated tubules, pyknotic nuclei, monocellular leukocytic inflammation, and interstitial hemorrhages compared to CCl4-injected group.
Table 5. Histopathological scores of kidney lesions in the different groups including, normal control, CCl4, CCl4/mice BM-MSCs, and CCl4/mice BM-MSCs group.
Immunohistochemical examination of kidney
Immunohistochemistry of the control kidney tissues stained with COX-2 showed a very weak immunoreaction for COX-2 in the kidney cortex (). Renal tissues stained with COX-2 in the CCl4 group of rats revealed elevated COX-2 in the renal tubule cells of the kidney cortex collecting duct (). However, immunohistochemistry of kidney tissues treated with rats and mice BM-MSCs stained with COX-2 revealed a lower level of immunoreaction for COX-2 in kidney tubular cells (, respectively).
Figure 4. Photomicrographs from immunohistochemistry of kidney section of rats showing (a): negative immunoreaction for COX-2 in kidney cortex. (b): Group CCl4 showing strong immune expression for COX-2 in renal tubule cells, (c): CCl4 + rats BM-MSCs of kidney cortex collecting and (d): CCl4 + mice BM-MSCs showing weak immunoreaction for COX-2 in collecting tubule cells of kidney cortex. (Immunostaning for COX-2; Scale bar of a–d = 50 µm).
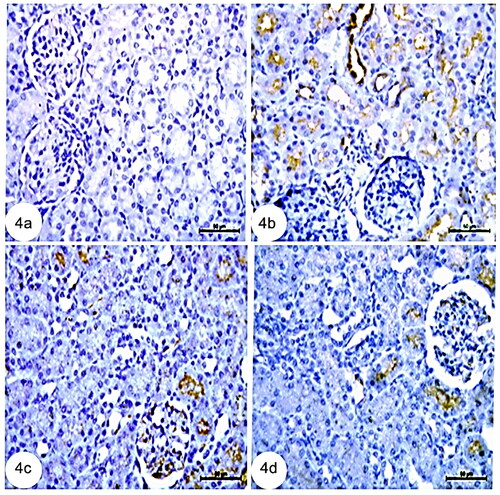
In addition, p53 immunoreactivity in the kidney tubular cells of the CCl4 group significantly surpassed that of the control group (). However, the control rats’ renal tubule structures displayed a very weak immunoreaction to p53 (). Meanwhile, immunohistochemistry of kidney tissues treated with rats BM-MSCs and mice BM-MSCs stained with p53 revealed a poor immunoreaction to p53 in kidney tubular cells (, respectively).
Figure 5. Photomicrographs from immunohistochemistry of kidney section of rats showing (a): control group with negative immunoreaction for p53 in renal tubules. (b): Group CCl4 showing strong positive immunoreaction for p53 in tubular cells of kidney. (c): CCl4 + rats BM-MSCs and (d): CCl4 + mice BM-MSCs showing poor immunoreaction for p53 in tubular cells of the kidney. (Immunostaning for p53; Scale bar of a, c, and d = 50 µm, Scale bar of b = 100 µm).
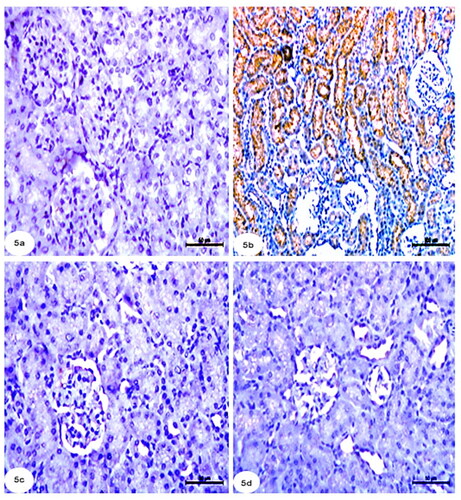
Compared with the control group, immunohistochemistry of the kidney revealed that the CCl4 group had a strong positive immunoreaction to TNF-α in renal tubules cells (). However, in control rats, a very weak immunoreaction to TNF-α was observed in the kidney’s tubular structures (). Moreover, immunohistochemistry of the kidney tissues treated with rats BM-MSCs and mice BM-MSCs stained with TNF-α showed a poor immunoreaction to TNF-α in the kidney’s tubular cells (, respectively).
Figure 6. Photomicrographs from immunohistochemistry of kidney section of rats showing (a): control group with negative immunoreaction for TNF-α in renal tubules. (b): Group CCl4 showing strong positive immunoreaction for TNF-α in tubular cells of kidney. (c): CCl4 + rats BM-MSCs and (d): CCl4 + mice BM-MSCs showing poor immunoreaction for TNF-α in tubular cells of the kidney. (Immunostaning for TNF-α; Scale bar of a–d = 50 µm).
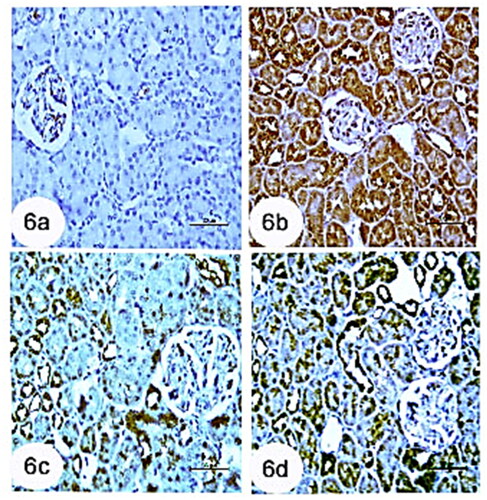
Changes in the average area % of the TNF-α, p53, and COX-2 immunoreactions in the control group, CCl4-injected, and groups that received rats and mice BM-MSCs (). CCl4 group renal tissue had considerably higher COX-2 and TNF-α levels than the normal group ().
Figure 7. Figure showing area % of Masson’s trichrome, COX-2, p53, and TNF-α immunohistochemistry in all studied groups. Means, which have different superscript letters, are significantly different at p < 0.05. a,b,c,dIndicated the difference or similarity between groups.
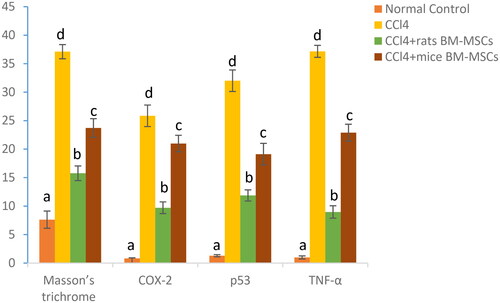
Table 6. Showing the changes in the area percentage of COX-2, p53, and TNF-α immunopositivity in all studied groups.
Electron microscopic examination of kidney
The renal glomerulus control group’s transmission electron microscopic analysis revealed a podocyte and regularly spaced normal-looking secondary foot processes and thin basal lamina, with wide longitudinal glomerular capillary (). The glomerular basement membrane (BM) was significantly thickened, secondary pedicle foot processes fused, and the nucleus of the primary thick foot process shrank in the CCl4 group’s renal glomerulus compared to the control group ()). Furthermore, kidney tissues treated with rats BM-MSCs displayed nearly normal glomerular BM and numerous, regularly spaced secondary foot processes ()), whereas kidney tissues treated with mice BM-MSCs displayed pronounced recovery of the euchromatic nucleus, primary thick foot process, and secondary foot processes on the glomerular BM ().
Figure 8. Electron micrograph of the renal glomerulus (a,b): control group showing a podocyte (P) with primary foot process (P1) and regularly spaced normal-looking secondary foot processes (P2) and thin basal lamina (BL), with wide longitudinal glomerular capillary (Ca) (Scale bar of a = 500 nm and Scale bar of b = 2 µm). (c,d): CCl4 group showing marked thickening of basal lamina (BL), fusion of secondary pedicles foot processes (P2), degenerated podocytes with pyknotic nucleus (P) and fusion of primary foot process (P1) (Scale bar = 2 µm). (e,f) CCl4 + rats’ BM-MScs showing almost normal thin basal lamina (BL), euchromatic nucleus (N), podocyte (P), and regularly spaced normal-looking secondary foot processes (P2) (Scale bar of e = 500 nm and Scale bar of f = 2 µm). (g,h) CCl4 + mice BM-MSCs showing marked recovery of the podocyte (P), euchromatic nucleus (N), with secondary foot processes (P2) and basal lamina (BL) (Scale bar of g = 500 nm and Scale bar of h = 2 µm).
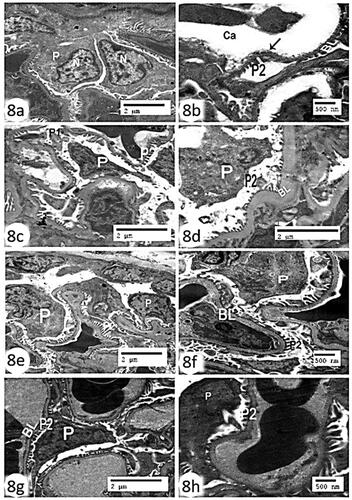
The kidneys of the control group in this study had proximal convoluted tubules, a regular nucleus, mitochondria, apical microvilli, a thin BM, and basal unfolding (), whereas those of the CCl4 group had obvious partial loss of microvilli, a thickening of the BM, significantly degenerated basal in folding, noticeable phagosomes, some vacuoles, swollen (). Contrarily, organelles such the nucleus, mitochondria, microvilli, thin BM, and basal unfolding were almost completely normal in kidney tissues treated with rats and mice BM-MSCs (, respectively).
Figure 9. Electron micrographs of kidney sections of proximal convoluted tubules (a): control group showing regular nucleus (N), mitochondria (M), apical microvilli (MV), thin basement membrane (BM), and basal infoldings (BI) (Scale bar = 2 µm). (b–d); CCl4 group showing obvious partial loss of microvilli (MV), thickening basement membrane (BM), displaying marked degeneration of basal infoldings (BI). Notice the presence of phagosomes (arrow), some vacuoles (V), some swollen electron dense mitochondria (curve arrow) and other vacuolated ones (arrow head) (Scale bar =2 µm). (e): CCl4 + rats BM-MSCs and (f): CCl4 + mice BM-MSCs showing almost normal organelles including nucleus (N), mitochondria (M), microvilli (MV), thin basement membrane (BM), and basal infoldings (BI) (Scale bar =2 µm).
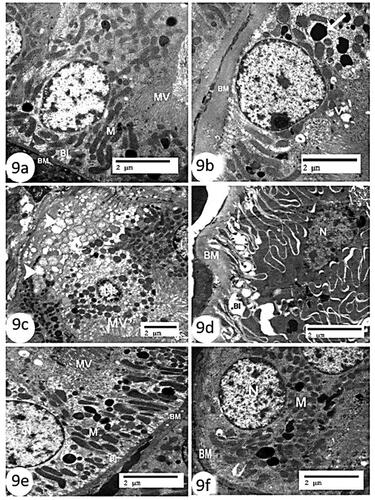
Furthermore, the control group’s distal convoluted tubules were distinguished by lined cells with spherical to oval nuclei that extended deeply to basal cell infoldings, with numerous elongated mitochondria in between and a somewhat thin basal lamina (). In contrast, while the CCl4 group’s distal convoluted tubules had a region of cytoplasm rarefaction, significantly degenerated lining cells, and a clear loss of basal unfolding (), the mice and rats BM-MSC-treated groups had preserved tubular cell ultrastructure compared to the control group ().
Figure 10. Electron micrographs of kidney sections of distal convoluted tubules (a): control group showing lining cells with spherical to oval nuclei (N), extending deeply basal cell infoldings with numerous elongated mitochondria in between (M), and somewhat thin basal lamina (arrow) (Scale bar = 2 µm). (b): CCl4 group showing region of cytoplasm rarefaction (astric), marked degenerated lining cells (arrow), swollen degenerated basal infolded mitochondria (M) and obvious loss of basal infoldings (arrow head) (Scale bar = 2 µm). (c) CCl4 + rats’ BM-MSCs and (d): CCl4 + mice BM-MSCs showing marked recovery of the nucleus (N), elongated mitochondria (M), and well-defined basal infoldings (arrow) (Scale bar = 2 µm).
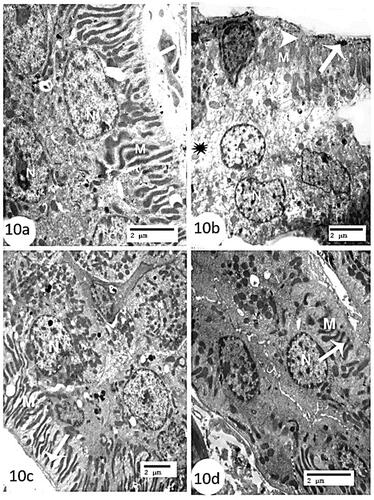
Considering the control group’s kidney, electron microscopy revealed CTs of two cell types in the cortical collecting ducts, which were lined by principal (light) cells and intercalated (dark) cells (). The dark cells had electron-dense cytoplasm, whereas the light cells had more electron-lucent cytoplasm (). The CCl4 group demonstrated that the light cells had pale cytoplasm with electron-dense mitochondria and that the basal cell membrane folded frequently, whereas the dark cells had a few oval mitochondria and some vacuoles (). Concurrently, significant recovery of light cells and dark cells with numerous mitochondria was observed in the group treated with rats BM-MSCs (), but light cells with numerous mitochondria and dark cells with elongated mitochondria and basal cell membrane were observed in the group treated with mice BM-MSCs ().
Figure 11. Electron micrograph of collecting tubules (a): control group showing two cell types are seen in the collecting ducts of the cortex are lined by principal (light) (L) cells and intercalated (dark) (D) cells. The dark cells are characterized by having more electron-dense cytoplasm (arrow head). The light cells have more electron-lucent cytoplasm (arrow). Relatively long tight junctions (J) (Scale bar of a = 2 µm). (b): CCl4 group showing the principal (light) (L) cell has pale cytoplasm with electron-dense mitochondria (arrow), and frequent infolding’s of the basal cell membrane (curve arrow). The intercalated (dark) (D) cells appeared a few mitochondria (arrow) and some vacuoles (V) (Scale bar of b = 2 µm). (C): CCl4 + rats BM-MSCs showing marked recovery of the principal (light) (L) cells and intercalated (dark) (D) cells have numerous mitochondria (arrow) (Scale bar of c = 2 µm). (d): CCl4 + mice BM-MSCs showing almost normal of the principal (light) (L) cells with numerous mitochondria (arrow) and intercalated (dark) (D) cells have elongated mitochondria (arrow) (Scale bar of c = 2 µm).
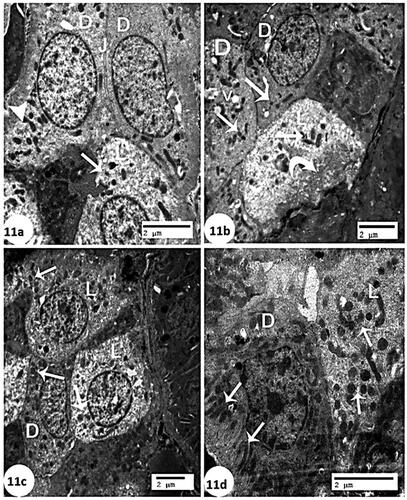
Discussion
To evaluate kidney function, the serum concentrations of urea, uric acid, and creatinine were evaluated. These three measures (or muscle atrophy in the case of creatinine) are affected by severe renal nephron injury [Citation58]. In our investigation, CCl4 injection caused a considerable rise in these three renal function markers because of reduced kidney function. Many kidney disorders start and progress as a result of oxidative stress [Citation59].
According to earlier studies, CCl4 exposure harms kidneys. The mechanism of CCl4 action in tissues comprises reductive dehalogenation brought on by CCl4 biotransformation, in which electrophiles and free radicals start the process of LPO; under these circumstances, if the LPO defeats the antioxidant defense mechanism, kidney damage results [Citation60]. As kidney damage is considered to be a significant public health concern, in this study on rats, we looked at the potential role of BM-MSCs in protecting renal tissues from the dangers of CCl4 by assessing kidney function and structural integrity through biochemical, microscopic, and immunohistochemical investigations.
Creatinine, urea, and uric acid levels significantly rose after CCl4 injection in comparison to the control group. Treatments with mice and rats BM-MSCs caused a significant decrease in creatinine, urea, and uric acid levels, which is consistent with Zaahkouk et al.’s [Citation61] finding, which revealed that administering MSCs to rats resulted in decreased urea and creatinine levels and improved histological structure in the kidney tissue. According to Elbaghdady et al. [Citation62], BM-MSC treatment resulted in lower serum uric acid and creatinine levels.
In this study, injection of CCl4 led to an increase in renal LPO levels, which indicated increased LPO and demonstrated considerable damage (), as revealed by the severe injuries discovered in the kidney’s histology. Interestingly, injection of rats and mice BM-MSCs significantly reduced the extent of LPO by decreasing MDA levels, confirming the nephroprotective effect of BM-MSCs against CCl4-induced renal LPO (). Similarly, the CCl4-treated group significantly reduced MDA content in the kidney [Citation63–65].
Figure 12. A schematic representation of how BM-MSCs reduce oxidative stress, inflammation, and apoptosis to stop CCl4-induced kidney damage.
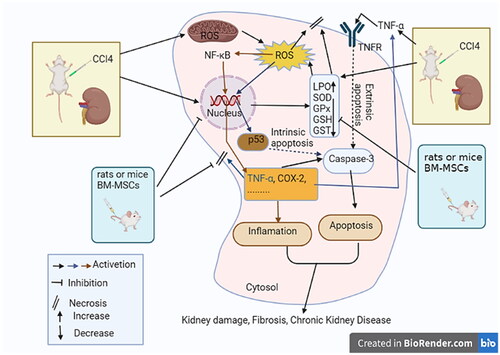
Our findings supported earlier studies that found that CCl4 administration caused significantly decreased GSH and GPx levels in rat kidney. However, our findings also demonstrated that GSH and GPx levels in the kidney were significantly increased in the CCl4 group treated with mice and rats BM-MSCs, which is consistent with previous studies that showed the same thing [Citation63,Citation65,Citation66]. In contrast, Yilmaz-Ozden et al. [Citation64] and Purucker et al. [Citation67] found that CCl4 enhanced GSH and GPx levels in rat kidneys. As an adaptive response to GSH-depleting agents and oxidative stress, higher GSH levels have been proposed [Citation64]. The variations in CCl4 dosages and kidney toxicity levels could be the cause of these contradictory results.
The antioxidant enzymes SOD and GST-related activities were significantly lower in the kidneys of the CCl4 group than in the healthy control group in this research. However, the treatment of CCl4-injected rats with rats and mice BM-MSCs significantly elevated SOD and GST levels, which is consistent with Ramarajan et al.’s [Citation68] results that revealed that pretreatment elevated SOD and GST activity in the kidneys of CCl4 rats by 49% and 99%, respectively. Notably, varying findings from research on the antioxidant status of kidneys in diverse toxin-induced nephrotoxicity have been seen due to toxin dosage and exposure [Citation64,Citation67]. Similarly, antioxidant enzyme activities in rat kidneys increased but decreased after treatment [Citation69,Citation70].
According to histopathological results, therapy with rats and mice BM-MSCs had a preventative impact on rats’ kidney injury after CCl4 exposure. In contrast, adult male rats with renal corpuscles from the CCl4-induced group displayed structural and ultrastructural alterations linked to disturbed glomerular filtration barrier integrity. We detected alterations in the ultrastructure of podocytes, shrunk hyper cellular glomeruli, and thickened glomerular BM. Animals treated with mice and rats BM-MSCs were able to halt the majority of these alterations.
The renal cortex of the rats in the CCl4 group had a number of structural deviations, including apparent completely destroyed glomeruli, dilated CTs, intertubular fibrosis, and inflammatory cell infiltration of the renal glomerulus, which were all discovered through histopathological analysis. El Wakeel and Mohamed [Citation71] studied the impact of BM-MSCs on CCl4-induced nephrotoxicity and the protective effect of BM-MSCs in rats and discovered that BM-MSCs have protective and cell-reinforcing effects against CCl4-prompted oxidative kidney damage [Citation7]. Similarly, Yilmaz-Ozden et al. [Citation64] investigated the kidney-damaging effects of CCl4 in rats.
BM-MSC-treated rats in this study most likely had a normal renal tubule structure compared to the CCl4 group. When Hamam [Citation72], El Wakeel and Mohamed [Citation71], and other scientists looked at the impact of BM-MSCs on CCl4 in the renal cortex of adult male albino rats, they found similar outcomes [Citation64,Citation73]. Despite these ameliorative changes, peritubular blood capillary congestion was observed in the recovery group, which is consistent with Makni et al.’s [Citation63] findings, who documented the rat Deoxyribonucleic acid (DNA) damage caused by CCl4’s nephrotoxic effects [Citation72].
According to MT-stained sections, the Bowman’s capsules and interstitium in this study’s CCl4-treated rats included more collagen fibers. The scattering of collagen fibers in the renal cortex did not differ noticeably between the groups of mice and rats treated with BM-MSC and the control rats, though. These findings supported Ahmed et al. [Citation74], who investigated the anti-inflammatory impact of What in rats treated to lessen renal damage and employed MT stain for normal collagen fiber distribution. El Wakeel and Mohamed [Citation71] investigated the role of BM-MSCs in the amelioration of renal injury and employed Mallory trichrome stain to address collagen accumulation around glomeruli [Citation75].
BM-MSCs have been proven to be crucial in a recent treatment for severe diseases due to their capacity to migrate to lesion sites and their multilineage differentiation functions [Citation76]. Our research focused on how BM-MSC therapy affected the ultrastructural and immunohistochemical alterations brought on by the rat nephrotoxicity caused by CCl4. While we found a positive reaction of COX-2 and p53 in CCl4-treated rats, the glomerulus, renal tubules, or interstitium showed a negative response in the control and BM-MSC-treated rats. These results support those of Rini et al. [Citation77] who found that renal cell cancer exhibits elevated COX-2 immunoreactivity. Additionally, Khan et al. [Citation78] discovered that the macula densa and thick ascending limbs of kidneys taken from sodium-depleted rats and dogs displayed a noticeable increase in COX-2 immunoreactivity (about three times greater than that of the control).
Lin et al. [Citation79] reported immunohistochemistry for the p53 protein in renal cell carcinoma, with nuclear staining in cancer cells. Uchida et al. [Citation80] immunohistochemically reported the prognostic relevance of the overexpression of the p53 protein in a homogeneous series of renal cell carcinoma tumor samples. According to Bashandy et al. [Citation81], the immunohistochemistry examination of p53 immunoreactive cells in renal tissues revealed no immunological reaction in the control group. There were diffusely stained glomerular and renal tubular epithelial cells in the treated group. The intensity of the colors, however, significantly decreased in the treated group. DNA damage and apoptosis are brought on by oxidative stress, which may be precisely measured by the p53 gene’s transcriptional regulation [Citation82]. The activation of p53 expression by exacerbated oxidative stress and production of ROS leads to activation of caspase-3 and apoptosis through intrinsic pathway ().
Chemically induced kidney injury in rats has been linked to increased inflammatory TNF-α [Citation83]. TNF-α protein expression was also enhanced in the renal tissue of these rats according to immunohistochemistry. Our findings corroborate prior findings, demonstrating that CCl4-induced renal damage in rats is linked to kidney inflammation. In the CCl4 group, TNF-α protein expression was also elevated in the kidney tissue. Notably, TNF-α can also stimulate other inflammatory pathways, including those mediated by COX-2 [Citation84,Citation85]. Similar findings were made by Morsy et al. [Citation86], who noted that the immunological staining of the TNF-α treated BM-MSC group revealed few immune reactive cells. The inhibition of kidney TNF-α protein expression by intravenous treatment of CCl4-injected rats with either mice or rats BM-MSC in this study may result in suppression of inflammation on one hand, and attenuation of apoptosis through abating the extrinsic pathway of apoptosis on the other ().
In this investigation, rats given CCl4 showed dilated capsular spaces, pronounced glomerular BM thickening, and reduced primary thick foot process nuclei in the renal corpuscle. In addition, El Wakeel and Mohamed [Citation71] found that the renal corpuscles of the recovery group of BM-MSC-treated rats showed more or less normal podocytes with a euchromatic nucleus. Compared to the control group, this podocyte contained several secondary foot processes and a primary thick foot process on the BM of the glomerular capillary. Other researchers, however, discovered that transplanted MSCs had a negligible direct effect on tissue regeneration. The same researchers noted that 85% of MSCs failed to display endothelium and macrophage markers even after renal artery injection [Citation87]. In this manner, several researches have supported the alternative theory that the paracrine actions of MCSs, through their mitogenic, angiogenic, and antiapoptotic effects, are accountable for the observed improvement of renal functions [Citation88].
The proximal convoluted tubules in the nuclei of the CCl4-treated rats in our study had a shorter brush border. These ultrastructural alterations were consistent with the observations made by Hermenean et al. [Citation8] and El Wakeel and Mohamed [Citation71], who discovered alterations in both proximal and distal tubular epithelial cells, as shown by the presence of numerous lysosomes and dense granular bodies, altered mitochondria, and basal enfolding dilatation brought on by CCl4 [Citation71]. Histological examination revealed that the proximal convoluted tubules of the treated rats had a better structure of kidney tissues than the control rats’ kidney tissues. These findings support earlier research [Citation8,Citation89,Citation90].
Along with dilated basolateral plasma membrane and loss of apical plasma membrane microprojection, the CCl4 group rats also showed swollen degraded basal infolding (BI) in the mitochondria of distal convoluted tubules. Noted similar outcomes by Huuskes et al. [Citation90] and El Wakeel and Mohamed [Citation71]. The distal convoluted tubules of mice and rats BM-MSC-treated rats had preserved tubular cell ultrastructure compared to the control rats. The proximal and distal convoluted tubules of rats treated with mice and rats BM-MSCs showed euchromatic nuclei and a normal cytoplasmic appearance, indicating that BM-MSCs have antiapoptotic properties. MSCs can also secrete microvesicles, which appear to be a crucial part of the intercellular milieu, according to some scientists [Citation91]. According to other researchers [Citation87], MSCs are crucial for the apparent bidirectional connection between damaged and stem cells [Citation92]. Therefore, it is necessary to induce self-repair or reprogram the stem cell phenotypic to acquire particular tissue properties [Citation71].
In this study, CCl4 groups treated with mice and rats BM-MSCs likeliest showed the normal structure of renal CTs compared to the CCl4 control group. The collecting ducts are separated into cortical, outer medullary, and inner medullary collecting ducts, according to Bulger [Citation93], who also noted similar findings. Principal cells (bright) and intercalated cells (dark) line the cortical collecting ducts. After potassium adaptation or in response to high amounts of endogenous or exogenous mineralocorticoids, which promote potassium secretion through the collecting ducts, principal cells have shown dramatic increases in basolateral membrane surface density [Citation94–97]. When mice BM-MSCs or rats BM-MSCs were compared with CCl4- treated group, it was revealed that the group receiving injections of the rats BM-MSCs was more effective.
Conclusion
Although rats BM-MSCs and mice BM-MSCs potentially ameliorated the kidney function and structural integrity of CCl4-intoxicated rats, rats BM-MSCs seemed to be more effective.
Ethical approval
Ethics approval and consent to participate every animal’s procedures followed and guidelines set forth by the Scientific Animal Ethics Committee of the Faculty of Science at Beni-Suef University in Egypt for the Use and Care of Animals (Ethical Approval Number BSU/FS/ 2019-73).
Consent for publication
Not applicable.
Authors’ contributions
MA, SHA, and OMA conceived and designed the experiments. AA performed the experiments and analyzed the data. MA, SHA, and OMA provided experimental technical support and assisted in completing the study at different stages. AA drafted the manuscript. MA, SHA, and OMA finalized the article. All authors are in agreement with the contents of the manuscript. All authors read and approved the final manuscript.
Acknowledgments
The publication was supported by the Deanship of Scientifid Research at Prince Sattam Bin Abdulaziz University.
Disclosure statement
The authors declare no competing interests.
Data availability statement
This published article includes all of the data generated or analyzed during this investigation.
Additional information
Funding
References
- Yoshioka H, Usuda H, Fukuishi N, et al. Carbon tetrachloride-induced nephrotoxicity in mice is prevented by pretreatment with zinc sulfate. Biol Pharm Bull. 2016;39(6):1–22. doi: 10.1248/bpb.b16-00078.
- Staszak K. Halogened hydrocarbons–current trends. Phys Sci Rev. 2020;5(10):20190032. doi: 10.1515/psr-2019-0032.
- Unsal V, Cicek M, Sabancilar İ. Toxicity of carbon tetrachloride, free radicals and role of antioxidants. Rev Environ Health. 2021;36(2):279–295. doi: 10.1515/reveh-2020-0048.
- Ogeturk M, Kus I, Colakoglu N, et al. Caffeic acid phenethyl ester protects kidneys against carbon tetrachloride toxicity in rats. J Ethnopharmacol. 2005;97(2):273–280. doi: 10.1016/j.jep.2004.11.019.
- Ma JQ, Ding J, Xiao ZH, et al. Puerarin ameliorates carbon tetrachloride-induced oxidative DNA damage and inflammation in mouse kidney through ERK/Nrf2/ARE pathway. Food Chem Toxicol. 2014;71:264–271. doi: 10.1016/j.fct.2014.06.017.
- Liang X, Zhang J, Guo F, et al. Oxidative stress and inflammatory responses in the liver of swamp eel (monopterus albus) exposed to carbon tetrachloride. Aquaculture. 2018;496:232–238. doi: 10.1016/j.aquaculture.2018.07.026.
- Lock EA, Reed CJ. Xenobiotic metabolizing enzymes of the kidney. Toxicol Pathol. 1998;26(1):18–25. doi: 10.1177/019262339802600102.
- Hermenean A, Ardelean A, Stan M, et al. Protective effects of naringenin on carbon tetrachloride-induced acute nephrotoxicity in mouse kidney. Chem Biol Interact. 2013;205(2):138–147. doi: 10.1016/j.cbi.2013.06.016.
- Ritesh KR, Suganya A, Dileepkumar HV, et al. A single acute hepatotoxic dose of CCl4 causes oxidative stress in the rat brain. Toxicol Rep. 2015;2:891–895. doi: 10.1016/j.toxrep.2015.05.012.
- Cheng N, Ren N, Gao H, et al. Antioxidant and hepatoprotective effects of Schisandra chinensis pollen extract on CCl4-induced acute liver damage in mice. Food Chem Toxicol. 2013;55:234–240. doi: 10.1016/j.fct.2012.11.022.
- Popović D, Đukić D, Katić V, et al. Antioxidant and proapoptotic effects of anthocyanins from bilberry extract in rats exposed to hepatotoxic effects of carbon tetrachloride. Life Sci. 2016;157:168–177. doi: 10.1016/j.lfs.2016.06.007.
- Adewole S, Salako A, Doherty O, et al. Effect of melatonin on carbon tetrachloride-induced kidney injury in Wistar rats. Afr J Biomed Res. 2010;10(2):153–164. doi: 10.4314/ajbr.v10i2.50619.
- Ozturk F, Ucar M, Ozturk IC, et al. Carbon tetrachloride-induced nephrotoxicity and protective effect of betaine in Sprague-Dawley rats. Urology. 2003;62(2):353–356. doi: 10.1016/s0090-4295(03)00255-3.
- Javaid R, Aslam M, Nizami Q, et al. Role of antioxidant herbal drugs in renal disorders: an overview. Free Radicals and Antioxidants. 2012;2(1):2–5. doi: 10.5530/ax.2012.2.2.
- Jena AB, Samal RR, Bhol NK, et al. Cellular Red-Ox system in health and disease: the latest update. Biomed Pharmacother. 2023;162:114606. doi: 10.1016/j.biopha.2023.114606.
- Ganta S, Amuru SR, Anchapakula SL, et al. Hepato and nephroprotective effects of six important medicinal plants against toxic oxidative stress. Med Plants-Int J Phytomed Relat Ind. 2023;15(1):13–25. doi: 10.5958/0975-6892.2023.00004.7.
- Brentnall M, Rodriguez-Menocal L, De Guevara RL, et al. Caspase-9, caspase-3 and caspase-7 have distinct roles during intrinsic apoptosis. BMC Cell Biol. 2013;14:32. doi: 10.1186/1471-2121-14-32.
- Donder E, Baydas G, Ozkan Y, et al. Investigation of antioxidant effect of melatonin against carbon tetrachloride toxicity in various tissues. Biomed Res. 1999;10:141–145.
- Honda T, Hirakawa Y, Nangaku M. The role of oxidative stress and hypoxia in renal disease. Kidney Res Clin Pract. 2019;38(4):414–426. doi: 10.23876/j.krcp.19.063.
- Jha JC, Banal C, Chow BS, et al. Diabetes and kidney disease: role of oxidative stress. Antioxid Redox Signal. 2016;25(12):657–684. doi: 10.1089/ars.2016.6664.
- Chen PM, Yen ML, Liu KJ, et al. Immunomodulatory properties of human adult and fetal multipotent mesenchymal stem cells. J Biomed Sci. 2011;18(1):49. doi: 10.1186/1423-0127-18-49.
- Trivanović D, Kocić J, Mojsilović S, et al. Mesenchymal stem cells isolated from peripheral blood and umbilical cord Wharton’s jelly. Srp Arh Celok Lek. 2013;141(3–4):178–186. doi: 10.2298/sarh1304178t.
- Zhao G, Ge Y, Zhang C, et al. Progress of mesenchymal stem cell-derived exosomes in tissue repair. Curr Pharm Des. 2020;26(17):2022–2037. doi: 10.2174/1381612826666200420144805.
- Iwamoto T, Terai S, Hisanaga T, et al. Bone-marrow-derived cells cultured in serum-free medium reduce liver fibrosis and improve liver function in carbon-tetrachloride-treated cirrhotic mice. Cell Tissue Res. 2013;351(3):487–495. doi: 10.1007/s00441-012-1528-z.
- Chen L, Zhang C, Chen L, et al. Human menstrual blood-derived stem cells ameliorate liver fibrosis in mice by targeting hepatic stellate cells via paracrine mediators. Stem Cells Transl Med. 2017;6(1):272–284. doi: 10.5966/sctm.2015-0265.
- Lee CW, Hsiao WT, Lee OKS. Mesenchymal stromal cell-based therapies reduce obesity and metabolic syndromes induced by a high-fat diet. Transl Res. 2017;182:61–74.e8. doi: 10.1016/j.trsl.2016.11.003.
- Talukdar S, Das SK, Emdad L, et al. Autophagy and senescence: insights from normal and cancer stem cells. Adv Cancer Res. 2021;150:147–208. doi: 10.1016/bs.acr.2021.01.005.
- Terai S, Tsuchiya A. Status of and candidates for cell therapy in liver cirrhosis: overcoming the “point of no return” in advanced liver cirrhosis. J Gastroenterol. 2017;52(2):129–140 doi: 10.1007/s00535-016-1258-1.
- Tsuchiya A, Takeuchi S, Watanabe T, et al. Mesenchymal stem cell therapies for liver cirrhosis: MSCs as “conducting cells” for improvement of liver fibrosis and regeneration. Inflamm Regen. 2019;39(1):18. doi: 10.1186/s41232-019-0107-z.
- Tsuchiya A, Kojima Y, Ikarashi S, et al. Clinical trials using mesenchymal stem cells in liver diseases and inflammatory bowel diseases. Inflamm Regen. 2017;37(1):16. doi: 10.1186/s41232-017-0045-6.
- Kisseleva T, Uchinami H, Feirt N, et al. Bone marrow-derived fibrocytes participate in pathogenesis of liver fibrosis. J Hepatol. 2006;45(3):429–438. doi: 10.1016/j.jhep.2006.04.014.
- Pilling D, Roife D, Wang M, et al. Reduction of bleomycin-induced pulmonary fibrosis by serum amyloid P. J Immunol. 2007;179(6):4035–4044. doi: 10.4049/jimmunol.179.6.4035.
- Haudek SB, Trial J, Xia Y, et al. Fc receptor engagement mediates differentiation of cardiac fibroblast precursor cells. Proc Natl Acad Sci USA. 2008;105(29):10179–10184. doi: 10.1073/pnas.0804910105.
- Lin SL, Kisseleva T, Brenner DA, et al. Pericytes and perivascular fibroblasts are the primary source of collagen-producing cells in obstructive fibrosis of the kidney. Am J Pathol. 2008;173(6):1617–1627. doi: 10.2353/ajpath.2008.080433.
- Haddock R, Lin-Gibson S, Lumelsky N, et al. Manufacturing cell therapies: the paradigm shift in health care of this century. NAM Perspect. 2017;7(6):1–13. doi: 10.31478/201706c.
- Srinivasan A, Sathiyanathan P, Yin L, et al. Strategies to enhance immunomodulatory properties and reduce heterogeneity in mesenchymal stromal cells during ex vivo expansion. Cytotherapy. 2022;24(5):456–472. doi: 10.1016/j.jcyt.2021.11.009.
- Mason C, Dunnill P. Assessing the value of autologous and allogeneic cells for regenerative medicine. Regen Med. 2009;4(6):835–853. doi: 10.2217/rme.09.64.
- Paul G, Özen I, Christophersen NS, et al. The adult human brain harbors multipotent perivascular mesenchymal stem cells. PLoS One. 2012;7(4):e35577. doi: 10.1371/journal.pone.0035577.
- Fu X, Jiang B, Zheng B, et al. Heterogenic transplantation of bone marrow-derived rhesus macaque mesenchymal stem cells ameliorates liver fibrosis induced by carbon tetrachloride in mouse. Peer J. 2018;6:e4336. doi: 10.7717/peerj.4336.
- Mohamed HA, Elbastawisy YM, Elsaed WM. Attenuation of lipopolysaccharide-induced lung inflammation by ascorbic acid in rats: histopathological and ultrastructural study. SAGE Open Med. 2019;7:2050312119828260. doi: 10.1177/2050312119828260.
- Ahmed RH, Galaly SR, Moustafa N, et al. Curcumin and mesenchymal stem cells ameliorate ankle, testis, and ovary deleterious histological changes in arthritic rats via suppression of oxidative stress and inflammation. Stem Cells Int. 2021;2021:3516834–3516820. doi: 10.1155/2021/3516834.
- Abd-Allah GA, El-Bakry KA, Bahnasawy MH, et al. Protective effects of curcumin and ginger on liver cirrhosis induced by carbon tetrachloride in rats. Int J Pharmacol. 2016;12(4):361–369. doi: 10.3923/ijp.2016.361.369.
- Ahmed OM, Hassan MA, Saleh AS. Combinatory effect of hesperetin and mesenchymal stem cells on the deteriorated lipid profile, heart and kidney functions and antioxidant activity in STZ-induced diabetic rats. Biocell. 2020;44(1):27.
- Schultz A. Uric acid. Clin Chem. 1984;16(10):1261–1266.
- Koller A, Kaplan A, The CV Mosby Co St. Louis Toronto princeton. Clin Chem. 1984;418:1316–1324.
- Yagi K. Lipid peroxides and human diseases. Chem Phys Lipids. 1987;45(2–4):337–351. doi: 10.1016/0009-3084(87)90071-5.
- Matkovics B, Kotorman M, Varga IS, et al. Oxidative stress in experimental diabetes induced by streptozotocin. Acta Physiol Hungaric. 1997;85(1):29–38.
- Beutler E, Duron O, Kelly BM. Improved method for the determination of blood glutathione. J Lab Clin Med. 1963;61:882–888.
- Mannervik B, Guthenberg C. [28] glutathione transferase (human placenta). Method Enzymol. 1981;77: 231–235. doi: 10.1016/s0076-6879(81)77030-7.
- Marklund S, Marklund G. Involvement of the superoxide anion radical in the autoxidation of pyrogallol and a convenient assay for superoxide dismutase. Eur J Biochem. 1974;47(3):469–474. doi: 10.1111/j.1432-1033.1974.tb03714.x.
- Bancroft JD, Gamble M, editors. Theory and practice of histological techniques. London: Churchill Livingstone Elsevier; 2008.
- Bancroft JD, Layton C, Suvarna SK. Bancroft’s theory and practice of histological techniques. London: Churchill Livingstone Elsevier; 2013.
- Arsad SS, Esa NM, Hamzah H. Histopathologic changes in liver and kidney tissues from male Sprague Dawley rats treated with Rhaphidophora decursiva (roxb.) Schott extract. J Cytol Histol. 2014;s4(01):1–6. doi: 10.4172/2157-7099.S4-001.
- Cemek M, Kağa S, Simşek N, et al. Antihyperglycemic and antioxidative potential of Matricaria chamomilla L. in streptozotocin-induced diabetic rats. J Nat Med. 2008;62(3):284–293. doi: 10.1007/s11418-008-0228-1.
- Bozzola JJ, Russell LD. Electron microscopy: principles and techniques for biologists. Burlington (MA): Jones & Bartlett Learning; 1999.
- Duncan BD. Multiple range test for correlated and heteroscedastic means. Biometrics. 1957;13(2):164. doi: 10.2307/2527799.
- Aslan A, Gok O, Erman O, et al. Ellagic acid impedes carbontetrachloride-induced liver damage in rats through suppression of NF-kB, bcl-2 and regulating nrf-2 and caspase pathway. Biomed Pharmacother. 2018;105:662–669. doi: 10.1016/j.biopha.2018.06.020.
- Rahmat AA, Dar FA, Choudhary IM. Protection of CCl4-induced liver and kidney damage by phenolic compounds in leaf extracts of Cnestis ferruginea (de Candolle). Pharmacognosy Res. 2014;6(1):19–28. doi: 10.4103/0974-8490.122913.
- Antar SA, Ashour NA, Marawan ME, et al. Fibrosis: types, effects, markers, mechanisms for disease progression, and its relation with oxidative stress, immunity, and inflammation. Int J Mol Sci. 2023;24(4):4004. doi: 10.3390/ijms24044004.
- Ayache J, Beaunier L, Boumendil J, et al. Sample preparation handbook for transmission electron microscopy: techniques. Vol. 2. Berlin, Germany: Springer Science & Business Media; 2010.
- Zaahkouk SMA, Bakry S, Mansour A, et al. Therapeutic role of mesenchymal stem cells in cisplatin induced renal failure in adult male rats. Advan Biol Res. 2015;9(3):201–209.
- Elbaghdady HAM, Alwaili MA, El-Demerdash RS. Regenerative potential of bone marrow mesenchymal stem cells on cadmium chloride-induced hepato-renal injury and testicular dysfunction in Sprague Dawley rats. Ecotoxicol Environ Saf. 2018;164:41–49. doi: 10.1016/j.ecoenv.2018.07.019.
- Makni M, Chtourou Y, Garoui EM, et al. Carbon tetrachloride-induced nephrotoxicity and DNA damage in rats: protective role of vanillin. Hum Exp Toxicol. 2012;31(8):844–852. doi: 10.1177/0960327111429140.
- Yilmaz-Ozden T, Can A, Karatug A, et al. Carbon tetrachloride-induced kidney damage and protective effect of Amaranthus lividus L. in rats. Toxicol Ind Health. 2016;32(6):1143–1152. doi: 10.1177/0748233714555390.
- El-Haskoury R, Al-Waili N, Kamoun Z, et al. Antioxidant activity and protective effect of carob honey in CCl4-induced kidney and liver injury. Arch Med Res. 2018;49(5):306–313 doi: 10.1016/j.arcmed.2018.09.011.
- Khan MR, Siddique F. Antioxidant effects of Citharexylum spinosum in CCL4 induced nephrotoxicity in rat. Exp Toxicol Pathol. 2012;64(4):349–355. doi: 10.1016/j.etp.2010.09.009.
- Purucker E, Wernze W, Krandik G. Glutathione in plasma, liver, and kidney in the development of CCl 4-induced cirrhosis of the rat. Res Exp Med (Berl). 1995;195(4):193–199. doi: 10.1007/BF02576788.
- Ramarajan L, Somasundaram ST, Subramanian S, et al. Nephroprotective effects of colpomenia sinuosa (derbes & solier) against carbon tetrachloride induced kidney injury in wistar rats. Asian Pacific J Trop Dis. 2012;2:s435–S441. doi: 10.1016/S2222-1808(12)60199-6.
- Ozden S, Catalgol B, Gezginci-Oktayoglu S, et al. Methiocarb-induced oxidative damage following subacute exposure and the protective effects of vitamin E and taurine in rats. Food Chem Toxicol. 2009;47(7):1676–1684. doi: 10.1016/j.fct.2009.04.018.
- Ozden S, Catalgol B, Gezginci-Oktayoglu S, et al. Acute effects of methiocarb on oxidative damage and the protective effects of vitamin E and taurine in the liver and kidney of Wistar rats. Toxicol Ind Health. 2013;29(1):60–71. doi: 10.1177/0748233712446719.
- El Wakeel EE, Mohamed AZ. The protective effect of bone marrow-derived mesenchymal cells on nephrotoxicity induced by carbone tetrachloride in adult male albino rats: histological and ultrastructural study. J Am Sci. 2018;14(6):13–24. doi: 10.7537/marsjas140618.02.
- Hamam GG. Effect of bone marrow-derived mesenchymal stem cells on carbon tetrachloride-induced injury in the renal cortex in adult male albino rat: a histological study. Egypt J Histol. 2015;38(3):484–492. doi: 10.1097/01.EHX.0000470836.21211.60.
- Pontikoglou C, Deschaseaux F, Sensebé L, et al. Bone marrow mesenchymal stem cells: biological properties and their role in hematopoiesis and hematopoietic stem cell transplantation. Stem Cell Rev Rep. 2011;7(3):569–589. doi: 10.1007/s12015-011-9228-8.
- Ahmed A, Hassanin A, Hassan A, et al. Therapeutic effects of milk thistle extract against renal toxicity induced by diethylnitrosamine and carbon tetrachloride in adult rats. J Vet Anat. 2017;10(1):107–124. doi: 10.21608/jva.2017.37166.
- Ross MH, Pawlina W. Histology: a text and atlas. Philadelphia (PA): Harper & Row; 2011.
- Carlo Magliano D, Bringhenti I, Souza-Mello V. GW501516 ameliorates a Fructose-Induced inflammation independent of AT1r downregulation in kidney. Nuclear Recept Res. 2016;3(2):1–10. doi: 10.11131/2016/101206.
- Rini BI, Weinberg V, Dunlap S, et al. Maximal COX-2 immunostaining and clinical response to celecoxib and interferon alpha therapy in metastatic renal cell carcinoma. Cancer. 2006;106(3):566–575. doi: 10.1002/cncr.21661.
- Khan KNM, Venturini CM, Bunch RT, et al. Interspecies differences in renal localization of cyclooxygenase isoforms: implications in nonsteroidal antiinflammatory drug-related nephrotoxicity. Toxicol Pathol. 1998;26(5):612–620. doi: 10.1177/019262339802600504.
- Lin R, Flerova E, Wamsley CE, et al. Sarcomatoid renal cell tumor harboring a novel BYSL: TFEB fusion with concurrent TFEB amplification. Genes Chromosomes Cancer. 2023;62(6):353–360. doi: 10.1002/gcc.23125.
- Uchida T, Gao JP, Wang C, et al. Clinical significance of p53, mdm2, and bcl-2 proteins in renal cell carcinoma. Urology. 2002;59(4):615–620. doi: 10.1016/s0090-4295(01)01601-6.
- Bashandy SA, Salama A, Fayed AM, et al. Protective effect of mandarin (citrus reticulata) peel extract on potassium dichromate induced hepatotoxicity and nephrotoxicity in rats. Plant Arch. 2020;20(1):2231–2242.
- Redza-Dutordoir M, Averill-Bates DA. Activation of apoptosis signalling pathways by reactive oxygen species. Biochim Biophys Acta. 2016;1863(12):2977–2992. doi: 10.1016/j.bbamcr.2016.09.012.
- Abdel-Zaher AO, Abd-Ellatief RB, Aboulhagag NA, et al. The interrelationship between gasotransmitters and lead-induced renal toxicity in rats. Toxicol Lett. 2019;310:39–50. doi: 10.1016/j.toxlet.2019.04.012.
- Yang CM, Yang CC, Hsiao LD, et al. Upregulation of COX-2 and PGE2 induced by TNF-α mediated through TNFR1/MitoROS/PKCα/P38 MAPK, JNK1/2/FoxO1 Cascade in human cardiac fibroblasts. J Inflamm Res. 2021;14:2807–2824. doi: 10.2147/JIR.S313665.
- Alanazi A, Nagi MN, Alhareth DY, et al. Crosstalk of TNF-α, IFN-γ, NF-kB, STAT1 and redox signaling in lipopolysaccharide/d-galactosamine/dimethylsulfoxide-induced fulminant hepatic failure in mice. Saudi Pharm J. 2023;31(3):370–381. doi: 10.1016/j.jsps.2023.01.005.
- Morsy WA, Kadry SM, Hassen MT, et al. Beetroot improves the therapeutic efficacy of bone marrow-derived mesenchymal stem cells in preventing cisplatin induced nephrotoxicity in male rats. Biointerface Res Applied Chem. 2022;13(1):1–21. doi: 10.33263/BRIAC131.031.
- Hao Q, Zhu YG, Monsel A, et al. Study of bone marrow and embryonic stem cell-derived human mesenchymal stem cells for treatment of Escherichia coli endotoxin-induced acute lung injury in mice. Stem Cells Transl Med. 2015;4(7):832–840. doi: 10.5966/sctm.2015-0006.
- Kunter U, Rong S, Djuric Z, et al. Transplanted mesenchymal stem cells accelerate glomerular healing in experimental glomerulonephritis. J Am Soc Nephrol. 2006;17(8):2202–2212. doi: 10.1681/ASN.2005080815.
- Donizetti-Oliveira C, Semedo P, Burgos-Silva M, et al. Adipose tissue-derived stem cell treatment prevents renal disease progression. Cell Transplant. 2012;21(8):1727–1741. doi: 10.3727/096368911X623925.
- Huuskes BM, Wise AF, Cox AJ, et al. Combination therapy of mesenchymal stem cells and serelaxin effectively attenuates renal fibrosis in obstructive nephropathy. FASEB J. 2015;29(2):540–553. doi: 10.1096/fj.14-254789.
- Gooneratne SR, Howell JM, Aughey E. An ultrastructural study of the kidney of normal, copper poisoned and thiomolybdate-treated sheep. J Comp Pathol. 1986;96(6):593–612. doi: 10.1016/0021-9975(86)90057-5.
- Camussi G, Deregibus MC, Bruno S, et al. Exosomes/microvesicles as a mechanism of cell-to-cell communication. Kidney Int. 2010;78(9):838–848. doi: 10.1038/ki.2010.278.
- Bulger RE. Kidney morphology: update 1985. Toxicol Pathol. 1986;14(1):13–25. doi: 10.1177/019262338601400103.
- Wade JB, O’Neil RG, Pryor JL, et al. Modulation of cell membrane area in renal collecting tubules by corticosteroid hormones. J Cell Biol. 1979;81(2):439–445. doi: 10.1083/jcb.81.2.439.
- Rastegar A, Biemesderfer D, Kashgarian M, et al. Changes in membrane surfaces of collecting duct cells in potassium adaptation. Kidney Int. 1980;18(3):293–301. doi: 10.1038/ki.1980.139.
- Stanton BA, Biemesderfer D, Wade JB, et al. Structural and functional study of the rat distal nephron: effects of potassium adaptation and depletion. Kidney Int. 1981;19(1):36–48. doi: 10.1038/ki.1981.5.
- Le Hir M, Kaissling B, Dubach UC. Distal tubular segments of the rabbit kidney after adaptation to altered Na-and K-intake: II. Changes in Na-K-ATPase activity. Cell Tissue Res. 1982;224(3):493–504. doi: 10.1007/BF00213747.