Abstract
As a pattern recognition receptor, Toll-like receptor 4 (TLR4) is crucial for the development and progression of acute kidney injury (AKI). This study aims to explore whether the deubiquitinase Usp9x influences the TLR4/NF-B pathway to cause sepsis-induced acute kidney injury (S-AKI). The model of AKI was established in Sprague-Dawley rats using the cecal ligation and puncture (CLP) method, while renal tubular epithelial cell NRK-52E was stimulated with lipopolysaccharide (LPS) in vitro. All plasmids were transfected into NRK-52E cells according to the indicated group. The deubiquitinase of TLR4 was predicted by the online prediction software Ubibrowser. Subsequently, Western blot and Pearson correlation analysis identified Usp9x protein as a potential candidate. Co-IP analysis verified the interaction between TLR4 and Usp9x. Further research revealed that overexpression of Usp9x inhibited degradation of TLR4 protein by downregulating its ubiquitination modification levels. Both in vivo and in vitro experiments observed that interference with Usp9x effectively alleviated the inflammatory response and apoptosis of renal tubular epithelial cells (RTECs) induced by CLP or LPS, whereas overexpression of TLR4 reversed this situation. Transfection with sh-Usp9x in NRK-52E cells suppressed the expression of proteins associated with the TLR4/NF-κB pathway induced by LPS. Moreover, the overexpression of TLR4 reversed the effect of sh-Usp9x transfection. Therefore, the deubiquitinase Usp9x interacts with TLR4, leading to the upregulation of its expression through deubiquitination modification, and the activation of the TLR4/NF-κB signaling pathway, thereby promoting inflammation and apoptosis in renal tubular epithelial cells and contributing to sepsis-induced acute kidney injury.
Introduction
Sepsis is a systemic disease triggered by infection, characterized by heightened inflammation, prolonged host immunosuppression, and dysfunction of multiple organs [Citation1]. In sepsis, the kidneys are particularly vulnerable to early injury, with approximately two-thirds of septic patients experiencing acute kidney injury [Citation1,Citation2]. Sepsis-induced acute kidney injury (S-AKI) is a prevalent and severe condition in critically ill patients, which leads to a six- to eight-fold increase in mortality rates [Citation3]. The pathogenesis of S-AKI is not fully understood, resulting in the current clinical treatment is still nonspecific. Hence, it is crucial to comprehensively understand the underlying mechanisms and pathogenesis of S-AKI to facilitate the development of efficacious treatment strategies.
A growing body of research supports the notion that inflammatory responses play a key role in the development and progression of S-AKI [Citation4–6]. Lipopolysaccharide (LPS), known as the major component of Gram-negative bacteria, has been utilized to establish sepsis-induced AKI models. Toll-like receptor 4 (TLR4) functions as a pattern recognition receptor that directly interacts with LPS, leading to the activation of diverse signaling pathways [Citation7,Citation8]. Activation of TLR4 signaling regulates the nuclear factor (NF)-κB, leading primarily to phosphorylation of IκB-α and nuclear transport of most p65-containing heterodimers [Citation9]. In addition, NF-κB induces the transcription of pro-inflammatory cytokines, including cytokines [tumor necrosis factor (TNF) -α, interleukin (IL) -1β, and IL-6] [Citation10,Citation11]. Inflammatory responses have the capacity to directly harm renal tubular epithelial cells (RTECs), while damaged RTECs can reciprocally regulate and strengthen inflammation, leading to tubule apoptosis and the development of AKI [Citation4,Citation12]. Therefore, inhibiting TLR4/NF-κB signaling has anti-inflammatory and anti-apoptotic effects, thereby effectively alleviating LPS-induced RTECs damage and sepsis-induced AKI [Citation6,Citation13–15].
Ubiquitination is a reversible post-translational modification that covalently binds ubiquitin (Ub) protein to the substrate molecule, further regulating the activity, interaction, and stability of the target protein [Citation16]. Deubiquitinating enzymes (DUBs) remove ubiquitin modification and enhance protein stability [Citation17]. A growing number of DUBs, such as BAP1, USP36, and USP7, has been implicated in manipulating pathological changes, inflammatory responses, apoptosis, and oxidative stress in renal tissue [Citation18–20]. The termination of the inflammatory response is facilitated by the intracellular translocation of TLR4 followed by its degradation through the ubiquitin-proteasome system pathway [Citation21]. Similarly, it has been reported that TLR4 is also regulated by DUBs. For example, Tripartite Motif Containing 44 (TRIM44) regulates the ubiquitination modification of TLR4 and stabilizes its expression to ultimately activate the AKT/mTOR pathway [Citation22]. In hepatocellular carcinoma, the tumor growth and metastasis are promoted by USP13 through its regulation of TLR4 and subsequent activation of the TLR4/MyD88/NF-κB signaling cascade [Citation23]. However, the involvement of deubiquitinase in sepsis-induced AKI by regulating TLR4 has been rarely reported.
In this study, we predicted the deubiquitinase of TLR4 using the online prediction software Ubibrowser 2.0 and identified the Ubiquitin-specific peptidase 9, X-linked (Usp9x). Usp9x has been shown to impair CD8+ T cell dysfunction and inhibit cytolytic activity of cells by inhibiting autophagy [Citation24]. In renal cells, Usp9x plays a crucial role in the development of diabetic nephropathy by modulating the epithelial-to-mesenchymal transition induced by high glucose [Citation25]. Nevertheless, Usp9x prevents the accumulation of extracellular matrix by activating the Nrf1-ARE pathway, ultimately alleviating the procession of diabetic renal fibrosis [Citation26]. However, the impact of Usp9x on sepsis-induced AKI remains unexplored in current research. Therefore, we induced animal models of S-AKI using cecal ligation and puncture (CLP), as well as established AKI cell models through LPS stimulation in RTECs, to investigate the involvement of Usp9x in S-AKI. Our findings indicate that Usp9x enhances the stability of TLR4 via deubiquitination, leading to the activation of the TLR4/NF-κB signaling pathway and subsequent induction of inflammation and apoptosis in RTECs.
Materials and methods
Sepsis-induced AKI rat model
Male Sprague-Dawley rats, aged 8 weeks, were randomly divided into two groups: the S-AKI group and the sham group, n = 6. Sepsis-induced AKI rat models were constructed by CLP [Citation27]. Before the experiment, the rats drank water rapidly for 12 h. All rats were anesthetized with isoflurane. After shaving the lower abdomen and disinfecting it with alcohol, then cut about 2 cm incision in the middle of the abdomen. Next, the cecum of the rats was exposed and secured below the ileocecal valve using a silk thread. Subsequently, a sterile 18-gauge needle was used to puncture the cecum at the site of the ligature. A small quantity of feces was gently expressed from the puncture site. The cecum was carefully repositioned within the abdominal cavity, and the incision was sutured using silk thread. In contrast, during CLP performance in the sham group, cecal ligation and puncture were intentionally omitted. This study was approved by the Institute Research Medical Ethics Committee of the First Affiliated Hospital of Nanchang University (CDYFY-IACUC-202311QR024).
Renal tubular separation
Kidney tubules can be separated according to the method described in previously report [Citation28]. The SD rats were euthanized by intraperitoneal injection of 0.4 mg/g ketamine and 0.04 mg/g metyrapone. The kidneys were immediately removed, placed in a 4 °C saline dish containing dual antibiotics, and the renal capsule was removed by blunt dissection. The renal cortex was then separated and cut into approximately 1 mm pieces. The renal cortex was rinsed with saline 3 times and transferred to a Dulbecco’s Modified Eagle Medium/Nutrient Mixture F-12 (DMEM/F12) solution containing 0.25% serum and 1 g/L collagenase I at 37 °C for 20 min. The un-digested renal cortex was transferred to a fresh collagenase solution and further digested for 20 min. The cortex suspension obtained from both digestions was filtered through a cell strainer (100 mesh). The filtrate was then transferred to a centrifuge tube and centrifuged at 900 r/min for 6 min. The supernatant was discarded, and the pellet was resuspended in 4 mL of DMEM/F12 to form a cell suspension. A pre-prepared 45% Percoll separation solution was then gently poured over the cell suspension. The cell suspension was centrifuged at 1300 r/min for 30 min at 4 °C. The F2 layer, located near the bottom of the tube, was collected and considered as purified and isolated proximal tubular epithelial cell segments. These segments were then centrifuged and washed with saline at 1000 r/min for 10 min.
Lentiviral vector injection
To evaluate the function of Usp9x silencing on S-AKI, Lentivirus LV-sh-Usp9x or LV-NC (negative control) was constructed by using pGLVU6-GFP (C06001, GenePharma, China) followed by intravenous injection into rats via the tail vein (1 × 109/mL, 100 μL) [Citation29]. CLP induction was performed two weeks after injection. After 24 h of the surgery, kidney, blood, and urine were collected and renal tubules were isolated.
Cell culture and transfection
The NRK-52E (CL-0174, Pricella, China) cells were cultured in a 37 °C with 5% CO2 incubator using Dulbecco’s modification of Eagle’s medium (DMEM) supplemented with 10% fetal bovine serum and 1% penicillin/streptomycin. Then cells were inoculated to 96-well plates and divided into eight groups: NC (NRK-52E cells without any treatment), LPS (NRK-52E cells were co-cultured with LPS for 24 h), Vector (NRK-52E cells were transfected with empty p3xFlag-Vector), Usp9x-OV (NRK-52E cells were subjected to transfection with Usp9x overexpression vector p3xFlag-Usp9x), LPS + sh-RNA (After transfection with LV-NC for 48h, NRK-52E cells were exposed to LPS treatment), LPS + sh-Usp9x (Following transfection with LV-sh-Usp9x for 48h, NRK-52E cells were subjected to LPS treatment), LPS + sh-Usp9x + vector (NRK-52E cells were co-transfected with LV-sh-Usp9x or empty p3xFlag-Vector, and treated with LPS after transfection for 48 h), and LPS + sh-Usp9x + TLR4 (NRK-52E cells were co-transfected with LV-sh-Usp9x and TLR4 overexpression vector p3xFlag-TLR4, and treated with LPS after transfection for 48 h). The Usp9x and TLR4 overexpression vectors were both constructed by using the p3xFlag [Citation30]. Lipofectamine 2000 (11668030, Thermo Fisher Scientific, USA) was used to transfect the plasmid according to manufacturer’s instructions. Lentivirus LV-sh-Usp9x or LV-NC was transfected by using Polybrene (28728-55-4, Sanat Cruz, USA). After transfected with Usp9x-OV or Vector for 48 h, NRK-52E cells were treated with Cycloheximide (CHX, eukaryotic protein synthesis inhibitor, 10 μg/mL). Cells were collected at various time intervals (0, 2, 4, 6, and 8 h). For LPS treatment, NRK-52E cells were transfected for 48 h and incubated with 10 μg/mL LPS for 24 h. Following centrifugation of the cell suspension, the supernatant was collected and stored for subsequent experiments.
Immunofluorescence staining
To confirm the successful isolation of rat renal tubules, the expression of the epithelial cell marker cytokeratin 18 (CK18) was detected by immunofluorescence. The tissue slices were fixed with 4% paraformaldehyde for 15 min, followed by incubation with 5% bovine serum albumin at room temperature for 2 h to block. After the washing step, the sections were incubated with CK18 primary antibody (HY-P81181, MedChemExpress, USA) at 4 °C overnight. The cell nuclei were counterstained with 4,6-diamidino2-phenyl-indole dihydrochloride (DAPI, C1005, Beyotime, China). Finally, the slides were observed under a fluorescence microscope.
Western blot analysis
The rat kidney tissue was washed with Phosphate buffer saline (PBS) 2-3 times and fully grind. The grinded rat kidney tissue and collected NRK-52E cells were treated with radioimmunoprecipitation assay (RIPA) buffer, and subsequent centrifugation to obtain protein samples. The proteins were separated through sodium dodecyl sulfate-polyacrylamide gel electrophoresis (SDS-PAGE) and subsequently transferred onto polyvinylidene difluoride (PVDF) membranes by a transmembrane system. To minimize nonspecific binding of antibodies, the PVDF membrane was blocked using a solution of 5% skim milk. The primary antibodies were added to the membrane and incubated overnight at 4 °C. The following antibodies were used: anti-TLR4 (SAB5700684, Merck KGaA, Germany), anti-Usp49 (PA5-104202, Thermo Fisher Scientific, USA), anti-Stambp (PA5-96565, Thermo Fisher Scientific, USA), anti-Usp8 (PA5-141050, Thermo Fisher Scientific, USA), anti-Usp9x (ab19879, abcam, USA), anti-Usp33 (PA5-116665, Thermo Fisher Scientific, USA), anti-Usp20 (A48222, Antibodies.com, UK), anti-Usp7 (PA5-34911, Thermo Fisher Scientific, USA), anti-β-actin (ab8227, abcam, USA), anti-IκB-α (ab32518, abcam, USA), anti-p-IκB-α (ab166462, abcam, USA), anti-p65 (ab16502, abcam, USA), anti-p-p65 (ab76302, abcam, USA), anti-caspase 3 (ab184787, abcam, USA), anti-cleaved-caspase 3 (PA5-114687, Thermo Fisher Scientific, USA), anti-Bcl-2 (ab194583, abcam, USA), anti-Bax (MA5-14003, Thermo Fisher Scientific, USA), CHOP (PA5-88116, Thermo Fisher Scientific, USA), ATF4 (PA5-27576, Thermo Fisher Scientific, USA), GRP78 (PA1-014A, Thermo Fisher Scientific, USA). The PVDF membrane was exposed to the appropriate secondary antibody at room temperature for 1-2 h. The color development on the PVDF membrane was achieved using an ECL Western blotting substrate (PE0010, Solarbio, China), and image analysis was performed using the Amersham Imagery 600 (GE healthcare) system.
Ubiquitination assay
The NRK-52E cells were subjected to transfection with Flag-TLR4, HA-ubiquitin and other indicated vectors. After transfection for 48 h, cells were treated with 10 μM proteasome inhibitor MG132 for 4 h. Subsequently, samples were collected for further analysis. Cell lysis was performed using ubi lysis buffer containing 0.5% sodium deoxycholate, 1% Nonidet P-40, 150 mM NaCl, 0.1% SDS, 50 mM Tris-HCl (pH = 8). The supernatant was collected by centrifuging the lysed cell mixture at 16,400 g for 15 min, and then incubated with anti-Flag (ab205606, abcam, USA) or anti-TLR4 (SAB5700684, Merck KGaA, Germany) at 4 °C overnight. The protein A/G beads were incubated with the supernatant at 4 °C for 2 h and washed with washing buffer for 3 times. Finally, immunoblotting (IB) was performed using anti-HA (ab18181, abcam, USA) or anti-Ub (ab303664, abcam, USA).
Co-inmunoprecipitation (Co-IP)
Co-Immunoprecipitation was performed to validate the interaction between Usp9x and TLR4 [Citation31]. After transfection for 48 h, cells were treated with 10 μM MG132 for 4 h. The cells were subjected to lysis using RIPA lysate. The extracted protein was incubated with the specified antibody (anti-Flag or anti-myc) in a shaker at 4 °C overnight. The immunoprecipitation was performed by incubating the mixture with Protein A/G beads at 4 °C for 2 h, followed by three washes with lysis buffer to elute the bound proteins. Immunoblotting analysis was conducted utilizing anti-Usp9x, anti-Flag, anti-TLR4, and anti-myc (ab19233, abcam, USA).
Enzyme-linked immunosorbent assay (ELISA)
The kidney injury markers serum creatinine (Scr) and blood urea nitrogen (BUN), the kidney injury molecule-1 (KIM-1) and neutrophil gelatinase-associated lipocalin (NGAL) in urine, as well as the pro-inflammatory factors IL-1β, IL-6, and TNF-α in serum were assessed using ELISA. The relevant indexes were determined according to the enzyme-linked immunosorbent assay kit (CNB0011, Thermo Fisher Scientific, USA) according to its instructions. Furthermore, the ELISA technique was employed to measure the concentrations of IL-1β, IL-6, and TNF-α in the supernatant of NRK-52E cells.
Flow cytometry assay
Apoptosis was detected using flow cytometry according to the method described in the study [Citation32]. The cells were digested with trypsin, washed with PBS, and fixed with 70% ethanol at 4 °C for 1-2 h. Subsequently, the cells were centrifuged at 200 g for 5 min and the supernatant was discarded. The cells were then resuspended in Annexin V-FITC binding solution and incubated at 20-25 °C for 10 min. After incubation, the cells were subjected to staining using 50 mg/mL propidium iodide. Finally, fluorescence detection was performed by flow cytometry.
Hematoxylin–eosin (HE) staining
The kidney sections were subjected to dewaxing in xylene, followed by rehydration with anhydrous ethanol, 90% alcohol, and 75% alcohol. Sections were stained with hematoxylin (C0107, Beyotime, China) for 3-8 min. After rinsing with water, sections were further stained with eosin (C0109, Beyotime, China) for 3 min. The stained samples were examined using a microscope, and the images were captured and analyzed.
Terminal dUTP nick-end labeling (TUNEL) staining
Apoptosis evaluation was conducted on paraffin-embedded renal tissues using TUNEL staining [Citation33]. The kidney sections were immersed in xylene for 5 min and dewaxed 3 times. Subsequently, incubation with protease K (20 μg/mL) at room temperature for 15 min was carried out. The sample was incubated with 50 μL TUNEL solution at 37 °C without light for 60 min. The sections were washed with PBS, followed by sealing with an anti-fluorescence quenching sealing solution and observed under a fluorescence microscope. The quantification of TUNEL-positive cells was performed using Image J software.
Immunohistochemistry
The kidney tissue is fixed and embedded in paraffin. After dewaxing and hydration, the sections are subjected to Periodic Acid Schiff (PAS) staining. The sections are incubated with F4/80 antibody (sc-377009, Santa Cruz Biotechnology, USA), Usp9x antibody (PA5-104202, Thermo Fisher Scientific, USA), and TLR4 antibody (SAB5700684, Merck KGaA, Germany) for 1 h. Then, the sections are stained with 3,3′-diaminobenzidine (DAB, P0-203, Beyotime, China) and hematoxylin is re-stained for 2 min. Finally, the cells are observed and photographed under a fluorescence microscope.
Statistical analysis
To determine the correlation between protein expression, Pearson correlation analysis was performed. The data analysis was conducted utilizing the GraphPad Prism 5.0 software. The comparison between the different groups was performed using one-way ANOVA analysis corrected by Tukey’s multiple comparisons test or Two-way ANOVA analysis corrected by Bonferroni’s multiple comparisons test. t-test was employed to assess the difference between two groups. Statistical significance was determined with P value < 0.05.
Results
Identification of the potential deubiquitinase of TLR4
To determine the DUBs of TLR4, we predicted candidates in different species (human, rat, and mouse) by the online software Ubibrowser 2.0 (http://ubibrowser.bio-it.cn/ubibrowser_v3/). The Venn diagram showed the presence of seven overlapping genes: Usp49, Stambp, Usp8, Usp9x, Usp33, Usp20, and Usp7 (). We established a rat model of S-AKI by CLP. Rats were euthanized 24 h after surgery, and renal tubules were isolated for analysis. Furthermore, we used immunofluorescence to detect the expression of renal tubular epithelial cell marker CK18. Our results demonstrated positive staining of CK18, confirming successful separation of renal tubules (Figure S2A). Compared to the sham group, the expression levels of TLR4, Usp49, Stambp, Usp9x, and Usp7 in the renal tubule of S-AKI rats were significantly upregulated. Conversely, Usp8 expression was downregulated, while there were no significantly changes observed in the expression levels of Usp33 and Usp20 (). The protein quantification results are shown in Figure S1. The correlation between DUBs and TLR4 protein expression was determined by Pearson correlation analysis. The results indicated that Usp9x was significantly correlated with TLR4 expression, while no significant correlations were observed for Usp49, Stambp, Usp8, Usp33, Usp20, Usp7 with TLR4 expression (). Additionally, we conducted Co-IP experiments in NRK-52E cells to verify the binding situation, and the results demonstrated varying degrees of binding between Usp49, Stambp, Usp8, Usp9x, Usp33, Usp20, and Usp7 with TLR4. Among them, Usp8, Usp9x, and Usp20 bound to TLR4 most tightly (). The AKI cell models were constructed by treating rat NRK-52E cells with LPS. It was observed that LPS promoted the protein expression of Usp9x in RTECs (). Therefore, Usp9x is considered as a potential DUB of TLR4 in S-AKI.
Figure 1. Identification of the potential DUBs of TLR4. (A) Venn diagram showing the potential DUBs predicted by Ubibrowser 2.0 software to bind TLR4, with overlapping proteins in three species: human (red), rat (purple), and mouse (green). (B) The expression levels of the above seven overlapping genes in renal tubule of sham and S-AKI groups were detected by western blot. Three biological replicates were performed in each group. (C–I) The protein expression correlation of TLR4 and seven overlapping genes was analyzed by Pearson correlation analysis. (J) Co-IP assay was used to verify the binding of Usp49, Stambp, Usp8, Usp9x, Usp33, Usp20, and Usp7 to TLR4. (K–L) Usp9x protein expression levels in LPS-treated NRK-52E cells were determined by Western blot, n = 3. NC was used as negative control, and β-actin was used as the internal reference protein. T-test was used to compare the difference between the two groups, **p < 0.01, compared with the NC group.
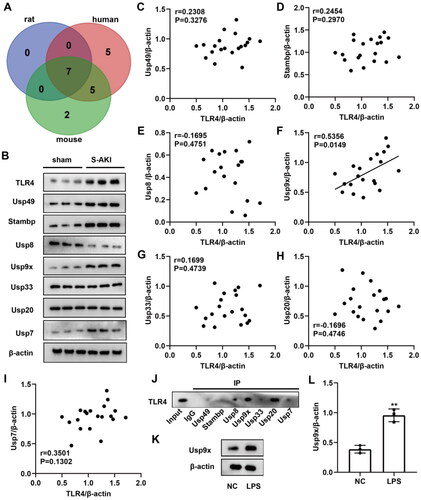
Usp9x interacts with TLR4 and regulates its ubiquitination modifications
To explore the potential interaction between Usp9x and TLR4 protein, Co-IP was used for verification. The results showed that anti-Flag immunoprecipitated Flag-TLR4 protein, and Usp9x protein was also co-precipitated (). Similarly, TLR4 protein can be detected in the immunoprecipitate of myc-Usp9x protein (). These results suggest an interaction between Usp9x protein and TLR4 protein.
Figure 2. Usp9x enhances the stability of TLR4 protein through deubiquitination modification and promotes the TLR4/NF-κB signaling pathway. (A, B) The physical interaction between Usp9x and TLR4 was detected by Co-IP assay. (A) Cells were transfected with Flag-TLR4 plasmid for 48h. The cell lysate was co-immunoprecipitated with anti-Flag (IP: Flag), and then immunoblotting assay was performed with anti-Flag and anti-Usp9x. (B) Cells transfected with myc-Usp9x plasmid were co-immunoprecipitated with anti-myc (IP: myc) and then subjected to immunoblotting assay with anti-myc and anti-TLR4. (C) NRK-52E cells were co-transfected with Flag-TLR4, Usp9x-OV (or Vector), and HA-Ub. Cells were lysed and immunoprecipitation was performed with anti-Flag, and then immunoblotting assay was performed with anti-HA. (D-F) NRK-52E cell lines were transfected with control (Vector) or Usp9x overexpressed (Usp9x-OV) plasmid for 48h. (D) Cells were treated with 10 μg/mL CHX for intervals of 0-8h. The protein expression level of TLR4 was detected by western blot. β-actin is used as the internal reference protein. Two-way ANOVA (Bonferroni’s multiple comparisons test) was performed. *p < 0.05, **p < 0.01, compared to the Vector group. (E) NRK-52E cells were treated with or without 10 μM proteasome inhibitor MG132 for 4 h. TLR4 expression was detected by western blot. (F) The expressions of TLR4, p-IκB-α, Iκb-α, p-p65 and p65 in cells were detected by Western blot. β-actin is used as the internal reference of total protein. One-way ANOVA and Tukey’s multiple comparisons test were applied for multi-group comparison. The data are presented as the mean ± standard deviation (SD) of at least three independent experiments. **p < 0.01, ***p < 0.001.
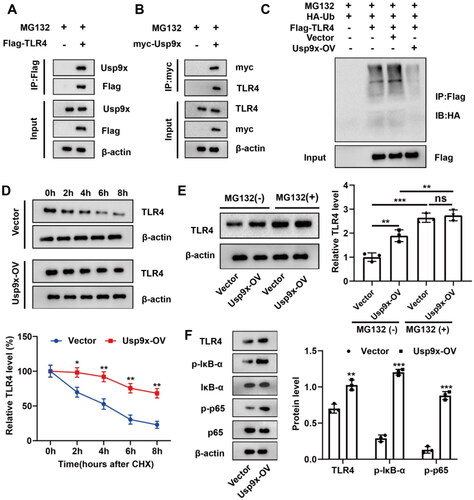
Then, we analyzed whether ubiquitination modification of TLR4 is regulated by Usp9x. NRK-52E cells were co-transfected with Flag-TLR4, HA-Ub and Usp9x-OV or Vector respectively, and then treated with MG132 to assess TLR4 ubiquitination modification level by immunoprecipitation assay. It was found that overexpression of Usp9x significantly reduced TLR4 ubiquitination modification level (). To evaluate the impact of Usp9x overexpression (Usp9x-OV) or Vector transfection, cells were treated with Cycloheximide (10 μg/mL). Protein samples were collected at various time points (0, 2, 4, 6, and 8 h), followed by western blot analysis to detect TLR4 protein expression levels. Compared to the control group, we found that overexpression of Usp9x inhibited TLR4 protein degradation (). In addition, treatment with MG132 significantly increased TLR4 protein expression, confirming that proteasome-mediated degradation of TLR4 protein was successfully blocked. Overexpression of Usp9x significantly increased TLR4 protein levels under normal conditions, but there were no significant changes after MG132 treatment, supporting that the influence of Usp9x on TLR4 involves a mechanism of proteasome-dependent degradation ().
In addition, we investigated the impact of Usp9x on the TLR4/NF-κB pathway in renal tubular epithelial cells. Usp9x upregulation enhanced the expression of TLR4, p-IκB-α, and p-p65 (). These findings suggest a direct interaction between Usp9x and TLR4, leading to inhibition of its degradation through deubiquitination and ultimately activating the TLR4/NF-κB signaling pathway.
Interference with Usp9x inhibits inflammation and apoptosis of renal tubular epithelial cells
To study the function of Usp9x in sepsis-induced AKI, we established stable Usp9x silencing cell lines in NRK-52E cells using lentiviral vectors containing Usp9x shRNA (LV-sh-Usp9x), and induced AKI cell model by LPS treatment after transfection. LPS promoted the expression of Usp9x and TLR4, while Usp9x silencing inhibited the expression of Usp9x and TLR4 (). Low ubiquitination levels were found in anti-TLR4 cell immunoprecipitation treated with LPS, while further addition of sh-Usp9x increased the ubiquitination level of TLR4 in the precipitation ().
Figure 3. Interference with Usp9x inhibits inflammation and apoptosis of renal tubular epithelial cells. NRK-52E cells were transfected with LV-sh-Usp9x (sh-Usp9x) or LV-NC (sh-RNA) vectors for 48h, and then incubated with LPS (10 μg/mL) for 24h. (A) The expressions of Usp9x and TLR4 in cells were detected by western blot. The protein expression was analyzed statistically, n = 3. (B) The cells of each group were treated with MG132 (10 μM) for 4h before sample collection, followed by immunoprecipitation using anti-TLR4, and then anti-Ubiquitin was applied to detect the ubiquitination level of TLR4. (C-E) The levels of inflammatory cytokines IL-1β, IL-6, and TNF-α in the supernatant of NRK-52E cell lysate were detected by ELISA. (F) Apoptosis was detected by flow cytometry. (G) The results of apoptosis in (F) were statistically analyzed and three biological replicates were performed. (H) The expression of apoptotic proteins cleaved-caspase 3, caspase 3, Bax and Bcl-2 were detected by western blot, n = 3. (I) The expression of ER stress markers CHOP, ATF4, and GRP78 were detected by western blot, n = 3. One-way ANOVA (Tukey’s multiple comparisons test) was performed. **p < 0.01, ***p < 0.001, compared with the NC group; #p < 0.01, ##p < 0.01, ###p < 0.001, compared with LPS + sh-RNA group.
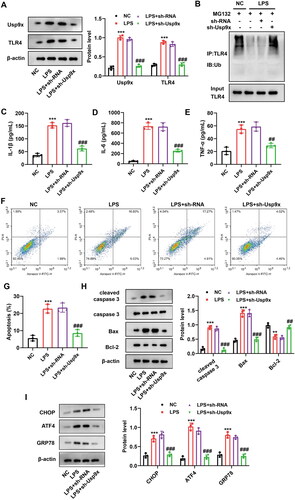
Subsequently, the effects of Usp9x silencing on cellular inflammation and apoptosis were investigated. The ELISA results showed that LPS promoted the secretion of IL-1β, IL-6, and TNF-α in cell supernatant, while interference with Usp9x effectively inhibited the secretion of above cytokines (). The flow cytometry results showed that LPS could promote apoptosis, while Usp9x silencing could reduce the apoptosis of NRK-52E cells (). Western blot analysis was employed to measure the expression levels of apoptotic proteins. Our findings demonstrated that LPS promoted the expression of cleaved-caspase 3 and Bax, while inhibited Bcl-2 expression. However, interference with Usp9x reversed these effects (). Furthermore, we further evaluated whether Usp9x is related to endoplasmic reticulum (ER) stress, and the results showed that LPS induced ER stress, while sh-Usp9x inhibited ER stress (). Therefore, it can be concluded that Usp9x interference inhibits LPS-induced inflammation response and apoptosis in RTECs.
Interference with Usp9x alleviates sepsis-induced acute kidney injury
To confirm the function of Usp9x in S-AKI, we delivered LV-sh-Usp9x or LV-NC into S-AKI rat models, which was validated by western blot (). As expected, interference with Usp9x increased the protein degradation of TLR4 and increased its ubiquitination modification in renal tubules of rat. However, in the renal tubules of the S-AKI group, Usp9x expression was significantly promoted, whereas TLR4 ubiquitination was inhibited, and its expression increased (). Similarly, the immunohistochemistry results showed that Usp9x and TLR4 were significantly upregulated in the renal tubules of the S-AKI group, while LV-sh-Usp9x reduced the expression of Usp9x and TLR4 (Figure S2B). Furthermore, we also studied the effect of Usp9x on the TLR4/NF-κB pathway. The S-AKI group exhibited elevated levels of p-IκB-α and p-p65 in tubular cells, while LV-sh-Usp9x reduced the expression of p-IκB-α and p-p65 (). In the kidney tissue of the S-AKI group, downregulation of Usp9x resulted in decreased levels of kidney injury markers Scr and BUN (), the KIM-1 and NGAL in urine (), as well as the pro-inflammatory factors IL-1β, IL-6, and TNF-α in serum (). In the S-AKI group, the levels of these markers exhibited a significant upregulation compared to the sham group. However, no improvement or remission of these markers was observed in the LV-NC negative control group (). Histological analysis of the rat kidneys revealed distinct renal tubular structures in the sham group. In contrast, pathological lesions such as tubular dilation and renal tubular swelling were observed in the S-AKI model, but downregulation of Usp9x could improve these symptoms (). In addition, TUNEL staining results demonstrated that apoptosis rate of kidney cell in the S-AKI group was significantly enhanced, while LV-sh-Usp9x injection effectively alleviated the apoptosis of kidney cells (). In addition, Western blot results showed that ER stress was activated in renal tubules in the S-AKI group, while LV-sh-Usp9x effectively suppressed this ER stress activation (). The infiltration of macrophages plays a critical role in kidney tissue during kidney injury and inflammation [Citation34]. To assess the extent of macrophage infiltration in rat kidneys, we employed a specific F4/80 antibody immunohistochemistry. The results showed that the level of macrophage infiltration was significantly increased in the S-AKI group, which was effectively inhibited by LV-sh-Usp9x (Figure S2C). These results suggest that interference with Usp9x alleviates septic acute kidney injury by reducing renal tubular injury, tissue inflammation, and apoptosis.
Figure 4. Interference with Usp9x alleviates septic acute kidney injury. Lentivirus carrying shRNA NC (LV-NC) or Usp9x shRNA (LV-sh-Usp9x) was injected into rats through the tail vein. CLP induction was performed two weeks after injection to construct S-AKI rat model. (A) The protein expressions of Usp9x, TLR4, p-IκB-α, IκB-α, p-p65, and p65 in renal tubules were detected by western blot, n = 3. β-actin is used as the internal reference protein. (B) The renal tubule lysates were IP with anti-TLR4, followed by IB with anti-Ubiquitin to analyze the ubiquitination modification of TLR4 protein. (C-I) Renal tubular biochemical indicators were measured by ELISA. One-way ANOVA (Tukey’s multiple comparisons test) was performed. (C-D) Scr and BUN levels; (E-F) Urine KIM1 and NGAL levels; (G-I) Serum levels of the inflammatory factors IL-1β, IL-6, and TNF-α. (J) HE staining of the renal tissues. The experiment was set up with three biological replicates. A representative image is shown in the figure. Bars = 50 μm. (K) TUNEL staining of the renal tissues. Bars = 50 μm. (L) Quantification of TUNEL-positive cells in each group. (M) The expression of ER stress markers CHOP, ATF4, and GRP78 were detected by western blot, n = 3. One-way ANOVA (Tukey’s multiple comparisons test) was used. ***p < 0.001, compared with sham group; ###p < 0.001, compared with S-AKI + LV-NC group.
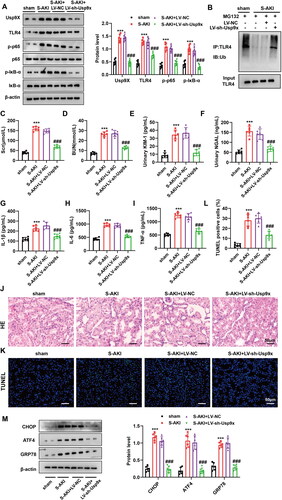
Interference with Usp9x alleviates renal tubular epithelial cell inflammation and apoptosis by inhibiting the TLR4/NF-κB pathway
To examine the function of TLR4 activation mediated by Usp9x in AKI, NRK-52E cells were transfected with lentiviral vectors containing Usp9x shRNA (LV-sh-Usp9x) or lentiviral vectors containing shRNA NC (LV-NC, as negative control), or co-transfected with LV-sh-Usp9x and TLR4 overexpression vector p3xFlag-TLR4 or empty p3xFlag-Vector. After 48 h transfection, NRK-52E cells were exposed to 10 μg/mL LPS for 24 h. Significantly, it was observed that inhibiting Usp9x resulted in the downregulation of TLR4, p-IκB-α, and p-p65 expression, while further overexpression of TLR4 promoted their expression (). We then evaluated the interaction of Usp9x and TLR4 in regulating inflammatory responses and apoptosis. Our results showed that upregulation of TLR4 enhanced the levels of pro-inflammatory cytokines, including IL-1β, IL-6, and TNF-α, in LV-sh-Usp9x transfected cells (). In the presence of Usp9x inhibition, the overexpression of TLR4 promoted the expression of cleaved-caspase 3 and Bax, while suppressing Bcl-2 expression (). Flow cytometry found that downregulation of Usp9x significantly suppressed apoptosis, while the upregulation of TLR4 reversed this effect (). In addition, Western blot results demonstrated that sh-Usp9x effectively suppresses ER stress, whereas this phenomenon can be reversed by the overexpression of TLR4 (). Collectively, these data demonstrate that Usp9x regulates inflammatory responses and apoptosis in a TLR4-dependent manner.
Figure 5. Interference with Usp9x alleviates renal tubular epithelial cell inflammation and apoptosis by inhibiting the TLR4/NF-κB pathway. (A) NRK-52E cells transfected with LV-sh-Usp9x (sh-Usp9x) or LV-NC (sh-RNA), or co-transfected with LV-sh-Usp9x (sh-Usp9x) and TLR4 overexpression vector (TLR4) or empty vector (vector) were treated with LPS. Cell lysates were then immunoblotted with the indicated antibodies (anti-TLR4, anti-p-IκB-α, anti-IκB-α, anti-p-p65, anti-p65, and anti-β-actin) to observe their expression. (B-D) Levels of the inflammatory factors IL-1β, IL-6, and TNF-α in each group were measured by ELISA. One-way ANOVA (Tukey’s multiple comparisons test) was used. (E) The expression of apoptotic proteins cleaved-caspase 3, caspase 3, Bax, and Bcl-2 were detected by western blot, n = 3. β-actin is used as the internal reference. (F) The apoptosis of each group was detected by flow cytometry. (G) The results of apoptosis rate in (F) were statistically analyzed. (H) The expression of ER stress markers CHOP, ATF4, and GRP78 were detected by western blot, n = 3. One-way ANOVA performed and statistically significant differences were indicated by: **p < 0.01, ***p < 0.001, compared with LPS + sh-RNA group; ##p < 0.01, ###p < 0.001, compared with LPS + sh-Usp9x + vector group.
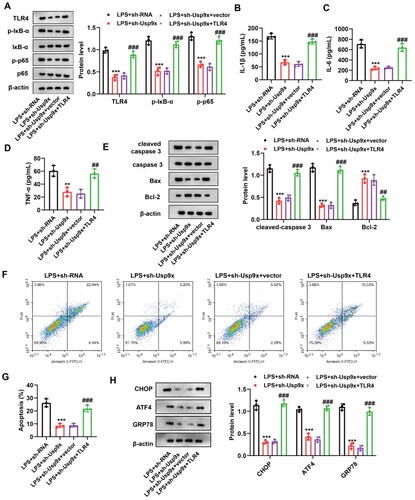
Discussion
This study focused on the role of upstream regulators of TLR4 in the regulation of inflammation and apoptosis in S-AKI renal tubular epithelial cells. Usp9x regulates the ubiquitination modification of TLR4 and inhibits its degradation. In addition, sh-Usp9x decreased the release of inflammatory cytokines and apoptosis in renal tubular epithelial cells, while TLR4 overexpression reversed this situation. Western blot analysis revealed that Usp9x enhanced TLR4, p-IκB-α and p-p65 protein expression. Based on these experimental findings, we propose that Usp9x may participate in S-AKI by modulating the TLR4/NF-κB pathway.
The pathogenesis of S-AKI is characterized by the adaptive and inflammatory response of tubular epithelial cells [Citation35]. Previous studies have highlighted the role of the TLR4/NF-κB signaling pathway in regulating inflammatory responses [Citation36,Citation37]. The interaction between TLR4 and MyD88 activates the NF-κB pathway, resulting in the production of TNF-α, IL-6β, and IL-1 [Citation38]. Specifically, these inflammatory factors can interact with transmembrane receptors to amplify the inflammatory response generated by early mediators and promote apoptosis in renal cells [Citation12]. Consequently, targeting TLR4 and its downstream regulators represents a promising strategy against AKI by preventing renal inflammation [Citation10]. Many drugs like Ulinastatin [Citation39], and Leonurine (LEO) [Citation40] showed significant renal protective effects on AKI via suppressing the TLR4/NF-κB signaling. Similarly, there are many upstream factors in S-AKI models that regulate TLR4, such as tripartite motif-containing protein 44 (TRIM44) [Citation22], miR-22 [Citation41], and long non-coding RNA SNHG5 [Citation13]. Therefore, we further explored the regulatory factors of TLR4/NF-κB to discover novel therapeutic targets for the management of S-AKI.
Ubiquitination is tightly regulated by ubiquitin E3 ligase, which can be reversed by DUBs and has been shown to be associated with numerous human diseases [Citation42,Citation43]. As E3 ubiquitin ligases, WWP2 and HRD1 have been proven to inhibit the apoptosis of RTECs caused by renal injury [Citation44,Citation45]. BAP1, acting as deubiquitinase, enhances the stability of BRCA1 protein through deubiquitination, suppresses NF-κB signaling, and ultimately mitigates LPS-induced RTEC injury and apoptosis [Citation18]. Liu et al. corroborate that Usp36 can reduce the inflammatory response to acute kidney injury [Citation19]. A related study has found that Usp9x knockdown reduces the production of pro-inflammatory cytokines, subsequently reducing tumor inflammation [Citation46]. Similarly, we observed that LPS promoted the expression of Usp9x, and interfered with Usp9x to inhibit the production of inflammatory factors, thereby alleviating apoptosis in renal tubular epithelial cells. In addition, Usp9x has been reported to inhibit diabetic renal fibrosis, suggesting that it may provide a key approach for treating renal injury [Citation26]. Our study found that LV-sh-Usp9x injection significantly attenuated the inflammatory response and renal tubule injury in S-AKI, suggesting that reducing Usp9x could be a potential and promising strategy to attenuate S-AKI.
The degradation of TLR4 via the ubiquitin-proteasome system pathway is implicated in the termination of the inflammatory response and renal protection [Citation21,Citation47–49]. Notably, DUBs can participate in cellular activities by specifically removing ubiquitin from TLR4 and inhibiting protein degradation [Citation22]. For example, the activation of TLR4/MyD88/NF-κB signaling is facilitated by Ubiquitin specific peptidase 13 (USP13) [Citation23]. Based on these theories, we discovered the deubiquitination enzyme Usp9x, which can regulate the stability of TLR4 protein. Previous finding has suggested that Usp9x overexpression promotes NF-κB activation [Citation50], which is in consistence with our results. Study has shown that the deubiquitination enzyme CYLD is a key negative regulator of TLR4-induced inflammation [Citation51], highlighting the close relationship between ubiquitination regulation of TLR4 and inflammation-related damage. Interestingly, our study also confirmed that interference with Usp9x inhibited levels of inflammatory cytokines and apoptosis in RTECs, which was reversed by overexpression of TLR4. These results suggest that Usp9x alleviates S-AKI through modulation of the TLR4/NF-κB inflammatory pathway.
Conclusion
This study provides evidence that Usp9x plays a crucial role in regulating the ubiquitination modification level of TLR4. In a sepsis-induced AKI model, interference with Usp9x resulted in decreased levels of TLR4/NF-κB pathway-associated proteins, and reduced inflammatory cytokine release and apoptosis, thereby alleviating S-AKI. These findings suggest that targeting Usp9x could be a promising therapeutic approach for treating S-AKI. However, this study was only discussed in animal models, and there is a lack of further clinical research evidence.
Supplemental Material
Download MS Word (1 MB)Disclosure statement
All authors declare that they have no conflict of interest.
Additional information
Funding
References
- Balkrishna A, Sinha S, Kumar A, et al. Sepsis-mediated renal dysfunction: pathophysiology, biomarkers and role of phytoconstituents in its management. Biomed Pharmacother. 2023;165:1. doi: 10.1016/j.biopha.2023.115183.
- He X-L, Chen J-Y, Feng Y-L, et al. Single-cell RNA sequencing deciphers the mechanism of sepsis-induced liver injury and the therapeutic effects of artesunate. Acta Pharmacol Sin. 2023;44(9):1801–14. doi: 10.1038/s41401-023-01065-y.
- Li J-C, Wang L-J, Feng F, et al. Role of heparanase in sepsis‑related acute kidney injury (review). Exp Ther Med. 2023;26(2):379. doi: 10.3892/etm.2023.12078.
- Ren Q, Guo F, Tao S, et al. Flavonoid fisetin alleviates kidney inflammation and apoptosis via inhibiting src-mediated NF-κB p65 and MAPK signaling pathways in septic AKI mice. Biomed Pharmacother. 2020;122:109772.
- Xie Y, Liu B, Pan J, et al. MBD2 mediates septic AKI through activation of PKCη/p38MAPK and the ERK1/2 axis. Mol Ther Nucleic Acids. 2021;23:76–88.
- Wang B, Xu J, Ren Q, et al. Fatty acid-binding protein 4 is a therapeutic target for septic acute kidney injury by regulating inflammatory response and cell apoptosis. Cell Death Dis. 2022;13(4):333. doi: 10.1038/s41419-022-04794-w.
- Duncan JBW, Basu S, Vivekanand P. Honey gold nanoparticles attenuate the secretion of IL-6 by LPS-activated macrophages. PLoS One. 2023;18(9):e0291076. doi: 10.1371/journal.pone.0291076.
- Gabarin RS, Li M, Zimmel PA, et al. Intracellular and extracellular lipopolysaccharide signaling in sepsis: avenues for novel therapeutic strategies. J Innate Immun. 2021;13(6):323–332. doi: 10.1159/000515740.
- Oeckinghaus A, Hayden MS, Ghosh S. Crosstalk in NF-κB signaling pathways. Nat Immunol. 2011;12(8):695–708. doi: 10.1038/ni.2065.
- Jha AK, Gairola S, Kundu S, et al. Toll-like receptor 4: an attractive therapeutic target for acute kidney injury. Life Sci. 2021;271:119155.
- Wan R, Zhang H, Liu S, et al. Role of fibro-adipogenic progenitors in skeletal muscle aging. APT. 2023;5(2):72–78. doi: 10.31491/APT.2023.06.116.
- Peerapornratana S, Manrique-Caballero CL, Gómez H, et al. Acute kidney injury from sepsis: current concepts, epidemiology, pathophysiology, prevention and treatment. Kidney Int. 2019;96(5):1083–1099.
- Wang M, Wei J, Shang F, et al. Down-regulation of lncRNA SNHG5 relieves sepsis-induced acute kidney injury by regulating the miR-374a-3p/TLR4/NF-κB pathway. J Biochem. 2021;169(5):575–583. doi: 10.1093/jb/mvab008.
- Li XK, Xu XZ, Cong Q, et al. Tri-domain proteins 27 reduce inflammation and apoptosis in HK-2 cells and protect against acute kidney injury in mice. Eur Rev Med Pharmacol Sci. 2020;24(23):12258–12266.
- Zhong Y, Wu S, Yang Y, et al. LIGHT aggravates sepsis-associated acute kidney injury via TLR4-MyD88-NF-κB pathway. J Cell Mol Med. 2020;24(20):11936–11948.
- Valles GJ, Bezsonova I, Woodgate R, et al. USP7 is a master regulator of genome stability. Front Cell Dev Biol. 2020;8:717. doi: 10.3389/fcell.2020.00717.
- Snyder NA, Silva GM. Deubiquitinating enzymes (DUBs): regulation, homeostasis, and oxidative stress response. J Biol Chem. 2021;297(3):101077.
- Luo S, Gong J, Zhao S, et al. Deubiquitinase BAP1 regulates stability of BRCA1 protein and inactivates the NF-κB signaling to protect mice from sepsis-induced acute kidney injury. Chem Biol Interact. 2023;382:110621. doi: 10.1016/j.cbi.2023.110621.
- Liu Q, Sheng W, Ma Y, et al. USP36 protects proximal tubule cells from ischemic injury by stabilizing c-myc and SOD2. Biochem Biophys Res Commun. 2019;513(2):502–508.
- Dong B, Ding C, Xiang H, et al. USP7 accelerates FMR1-mediated ferroptosis by facilitating TBK1 ubiquitination and DNMT1 deubiquitination after renal ischemia-reperfusion injury. Inflamm Res. 2022;71(12):1519–1533.
- Ciesielska A, Matyjek M, Kwiatkowska K. TLR4 and CD14 trafficking and its influence on LPS-induced pro-inflammatory signaling. Cell Mol Life Sci. 2021;78(4):1233–1261.
- Wei C-Y, Wang L, Zhu M-X, et al. TRIM44 activates the AKT/mTOR signal pathway to induce melanoma progression by stabilizing TLR4. J Exp Clin Cancer Res. 2019;38(1):137. doi: 10.1186/s13046-019-1138-7.
- Gao S, Chen T, Li L, et al. Hypoxia-Inducible ubiquitin specific peptidase 13 contributes to tumor growth and metastasis via enhancing the Toll-Like receptor 4/myeloid differentiation primary response gene 88/nuclear factor-κB pathway in hepatocellular carcinoma. Front Cell Dev Biol. 2020;8:587389. doi: 10.3389/fcell.2020.587389.
- Sheng L, Chen J, Tong Y, et al. USP9x promotes CD8 (+) T-cell dysfunction in association with autophagy inhibition in septic liver injury. Acta Biochim Biophys Sin (Shanghai). 2022;54(12):1–10.
- Sun XH, Xiao HM, Zhang M, et al. USP9X deubiquitinates connexin43 to prevent high glucose-induced epithelial-to-mesenchymal transition in NRK-52E cells. Biochem Pharmacol. 2021;188:114562.
- Huang K, Zhao X. USP9X prevents AGEs-induced upregulation of FN and TGF-β1 through activating Nrf2-ARE pathway in rat glomerular mesangial cells. Exp Cell Res. 2020;393(2):112100.
- Rittirsch D, Huber-Lang MS, Flierl MA, et al. Immunodesign of experimental sepsis by cecal ligation and puncture. Nat Protoc. 2009;4(1):31–36. doi: 10.1038/nprot.2008.214.
- Yilmaz DE, Kirschner K, Demirci H, et al. Immunosuppressive calcineurin inhibitor cyclosporine a induces proapoptotic endoplasmic reticulum stress in renal tubular cells. J Biol Chem. 2022;298(3):101589.
- Ding Y, Zhou D-Y, Yu H, et al. Upregulation of lncRNA NONRATG019935.2 suppresses the p53-mediated apoptosis of renal tubular epithelial cells in septic acute kidney injury. Cell Death Dis. 2021;12(8):771. doi: 10.1038/s41419-021-03953-9.
- Pal A, Dziubinski M, Di Magliano MP, et al. Usp9x promotes survival in human pancreatic cancer and its inhibition suppresses pancreatic ductal adenocarcinoma in vivo tumor growth. Neoplasia. 2018;20(2):152–164. doi: 10.1016/j.neo.2017.11.007.
- Ma Y, Huang L, Zhang Z, et al. CD36 promotes tubular ferroptosis by regulating the ubiquitination of FSP1 in acute kidney injury. Genes Dis. 2024;11(1):449–463.
- Tian F, Wang J, Zhang Z, et al. LncRNA SNHG7/miR-34a-5p/SYVN1 axis plays a vital role in proliferation, apoptosis and autophagy in osteoarthritis. Biol Res. 2020;53(1):9. doi: 10.1186/s40659-020-00275-6.
- Tan Z, Guo F, Huang Z, et al. Pharmacological and genetic inhibition of fatty acid-binding protein 4 alleviated cisplatin-induced acute kidney injury. J Cell Mol Med. 2019;23(9):6260–6270.
- Doi K, Leelahavanichkul A, Yuen PS, et al. Animal models of sepsis and sepsis-induced kidney injury. J Clin Invest. 2009;119(10):2868–2878.
- Manrique-Caballero CL, Del Rio-Pertuz G, Gomez H. Sepsis-Associated acute kidney injury. Crit Care Clin. 2021;37(2):279–301.
- Guo M, Gao J, Jiang L, et al. Astragalus polysaccharide ameliorates renal inflammatory responses in a diabetic nephropathy by suppressing the TLR4/NF-κB pathway. Drug Des Devel Ther. 2023;17:2107–2118.
- Rathore D, Nita-Lazar A. Phosphoproteome analysis in immune cell signaling. Curr Protoc Immunol. 2020;130(1):e105.
- Tian C, Liu X, Chang Y, et al. Rutin prevents inflammation induced by lipopolysaccharide in RAW 264.7 cells via conquering the TLR4-MyD88-TRAF6-NF-κB signalling pathway. J Pharm Pharmacol. 2021;73(1):110–117. doi: 10.1093/jpp/rgaa015.
- Wang J, Xu G, Jin H, et al. Ulinastatin alleviates Rhabdomyolysis-Induced acute kidney injury by suppressing inflammation and apoptosis via inhibiting TLR4/NF-κB signaling pathway. Inflammation. 2022;45(5):2052–2065. doi: 10.1007/s10753-022-01675-4.
- Han L, Chen A, Liu L, et al. Leonurine preconditioning attenuates ischemic acute kidney injury in rats by promoting Nrf2 nuclear translocation and suppressing TLR4/NF-κB pathway. Chem Pharm Bull (Tokyo). 2022;70(1):66–73.
- Zhang J, Chen Q, Dai Z, et al. miR-22 alleviates sepsis-induced acute kidney injury via targeting the HMGB1/TLR4/NF-κB signaling pathway. Int Urol Nephrol. 2023;55(2):409–421. doi: 10.1007/s11255-022-03321-2.
- Ren G-M, Li J, Zhang X-C, et al. Pharmacological targeting of NLRP3 deubiquitination for treatment of NLRP3-associated inflammatory diseases. Sci Immunol. 2021;6(58):eabe2933. doi: 10.1126/sciimmunol.abe2933.
- Zheng C, Chen J, Wu Y, et al. Elucidating the role of ubiquitination and deubiquitination in osteoarthritis progression. Front Immunol. 2023;14:1217466. doi: 10.3389/fimmu.2023.1217466.
- Huang Y, Sun Y, Cao Y, et al. HRD1 prevents apoptosis in renal tubular epithelial cells by mediating eIF2α ubiquitylation and degradation. Cell Death Dis. 2017;8(12):3202. doi: 10.1038/s41419-017-0002-y.
- Che H, He W, Feng J, et al. WWP2 ameliorates acute kidney injury by mediating p53 ubiquitylation and degradation. Cell Biochem Funct. 2020;38(6):695–701.
- Jaiswal A, Murakami K, Elia A, et al. Therapeutic inhibition of USP9x-mediated notch signaling in triple-negative breast cancer. Proc Natl Acad Sci USA. 2021;118(38):e2101592118.
- Wu X, Lei C, Xia T, et al. Regulation of TRIF-mediated innate immune response by K27-linked polyubiquitination and deubiquitination. Nat Commun. 2019;10(1):4115. doi: 10.1038/s41467-019-12145-1.
- Xu J, Liu J, Mi Y, et al. Triad3A-Dependent TLR4 ubiquitination and degradation contributes to the anti-inflammatory effects of pterostilbene on vascular dementia. J Agric Food Chem. 2022;70(19):5896–5910.
- Dai Y, Lu Q, Li P, et al. Xianglian pill attenuates ulcerative colitis through TLR4/MyD88/NF-κB signaling pathway. J Ethnopharmacol. 2023;300:115690. doi: 10.1016/j.jep.2022.115690.
- Xiang Y, Zhang S, Lu J, et al. USP9X promotes LPS-induced pulmonary epithelial barrier breakdown and hyperpermeability by activating an NF-κBp65 feedback loop. Am J Physiol Cell Physiol. 2019;317(3):C534–c543.
- Zhang Z, Zhang S, Jiang X, et al. Spata2L suppresses TLR4 signaling by promoting CYLD-Mediated deubiquitination of TRAF6 and TAK1. Biochemistry (Mosc). 2022;87(9):957–964. doi: 10.1134/S0006297922090085.