Abstract
Candida albicans is the primary cause of candidemia in hospitalized patients, and the intestinal tract is considered the source of most systemic infections. C. glabrata has emerged as the second or third most frequent cause of candidemia, but little is known about its epidemiology and pathogenesis. Our goal was to compare the intestinal colonization and extra-intestinal dissemination of C. glabrata and C. albicans (wild type and filamentation-defective mutant). Mice were pretreated with antibacterial agents to alter their resident microflora, and then orally inoculated with C. glabrata and/or C. albicans. Elimination of detectable cecal bacteria facilitated colonization with both Candida species. Selective elimination of aerobic/facultative gram-negative bacilli did not noticeably affect Candida colonization, but Escherichia coli overgrowth inhibited colonization. In all situations, C. glabrata colonized the cecum equally well or better than C. albicans, and the ability of C. albicans to form filaments did not facilitate colonization. In vitro generation times had little relevance to the resulting cecal population levels of C. glabrata and C. albicans, and neither species readily disseminated to mesenteric lymph nodes. Thus, like C. albicans, the intestinal tract may be an epidemiological reservoir for C. glabrata and antibiotic-induced alterations in intestinal bacteria may facilitate colonization.
Introduction
Candida species are the fourth most common cause of nosocomial bloodstream infections Citation[1–3], with associated mortality ranging from 38% to 75% Citation[2], Citation[3]. High-risk patients include neonates, diabetics, and those with the human immunodeficiency virus (HIV), but most cases occur in immunosuppressed, post-surgical, and trauma patients and a large proportion of cases occur in intensive care units Citation[2], Citation[4]. The gastrointestinal tract is believed to be the major colonizing habitat, and the source of most Candida infections Citation[2], Citation[5].
Of the more than 150 Candida spp., a single species (C. albicans) accounts for 50–75% of isolates from human clinical specimens Citation[6]. Morphogenesis (conversion from yeast to filamentous forms, namely germ tubes, pseudohyphae, and true hyphae) has been thought to play a role in colonization and virulence Citation[7]. With the exception of pseudohyphal growth under nitrogen-limiting conditions in vitroCitation[8], C. glabrata has not been observed to form filaments, and C. glabrata has emerged as the second or third most frequent cause of candidiasis Citation[6]. For example, in seven surgical intensive care units in the United States, Candida spp. from bloodstream infections were 48% C. albicans, 24% C. glabrata, 19% C. tropicalis, 7% C. parapsilosis, and 2% Candida spp. not otherwise specified Citation[5]. In a large survey of candidemia in the United States, Canada, and Latin America, 54% of episodes were attributed to C. albicans, 16% to C. glabrata, 15% to C. parapsilosis, 8% to C. tropicalis, 2% to C. krusei, and 5% to other Candida spp. Citation[9].
Compared with C. albicans, little is known about the pathogenesis of C. glabrata. Interestingly, C. glabrata is often isolated along with other microbes, including C. albicans. (We could not find comparable data for C. albicans.) Of 139 patients with sustained (>2 days) C. glabrata fungemia, 28 (20%) had polymicrobial blood cultures and the most common co-pathogens were coagulase-negative staphylococci, enterococci, and C. albicans. In addition, 59 (42%) of these patients had at least a single positive blood culture for bacteria or other Candida spp. during the 30 days preceding C. glabrata fungemia Citation[10]. Of 98 medical intensive care unit and bone marrow transplant patients, C. glabrata was isolated from 24 patients, and most (17 patients or 71%) had concomitant isolates of other Candida spp., of which the vast majority were C. albicansCitation[11]. Fidel et al. Citation[6] commented that the incidence of symptomatic oropharyngeal C. glabrata infection was difficult to determine because C. glabrata was generally co-isolated with C. albicans.
Risk factors for C. glabrata infections are similar to C. albicans, namely neutropenia, prolonged hospitalization, antibiotic use, abdominal surgery, instrumentation, and a break in anatomic barriers Citation[6], Citation[10]. Like C. albicans, mortality from systemic C. glabrata infection is high Citation[10]. However, C. glabrata is considered relatively avirulent compared with C. albicans, and it has been speculated that lack of hyphae may contribute to this relatively low virulence Citation[6]. Compared with C. albicans, mice injected intravenously require a much higher inoculum of C. glabrata to initiate a similar infection Citation[6], Citation[12]. Although the lack of virulence for normal mice is not consistent with the high mortality associated with systemic infection in humans, patients with systemic C. glabrata infection are often elderly and/or debilitated Citation[6], Citation[13]. The intestinal tract likely provides at least one epidemiological reservoir for C. glabrata, and C. glabrata can be isolated from the oropharynx and rectum of high-risk patients Citation[6], Citation[14]. However, there is no established animal model of intestinal C. glabrata colonization. The present experiments were designed to compare the abilities of C. glabrata and C. albicans (a wild-type strain and a mutant strain defective in filamentation) to colonize the ceca and translocate to the draining mesenteric lymph nodes (MLN) of orally inoculated mice.
Materials and methods
C. albicans and C. glabrata
C. glabrata ATCC 15126 was obtained from the American Type Culture Collection (ATCC; Manassas, VA, USA) and this strain was used in experiments, unless otherwise stated. C. albicans YA062 and C. glabrata 3328-7 are human clinical isolates. C. albicans CAF2 Citation[15] is the wild-type parent strain of HLC54. C. albicans HLC54 has the cph1/cph1 efg1/efg1 genotype reflecting a lack of CPH1 and EGF1 gene function Citation[16]. C. albicans CAF2 filaments normally in the ceca of antibiotic-treated mice, but strain HLC54 remains in the yeast form Citation[17]. Strain HLC54 was initially described as a nonfilamentous mutant Citation[16], but subsequent studies revealed limited filamentation in specific environments, i.e. on the surface of rough agar, and on the superficial tongue epithelium of orally inoculated germ-free piglets Citation[18], and in the kidney of intravenously inoculated mice Citation[19]. For experiments, Candida inocula were prepared as described previously Citation[19].
To assess the in vitro generation time, a pure culture was inoculated into a minimal medium broth supplemented with 5% dextrose, incubated with shaking, and sampled at 2-h intervals. The doubling time in minutes was calculated as 120/(log10N1 − log10N0/log2), where N0 and N1 are the smaller and larger numbers from a 2-h interval during log phase growth.
Mice
Female Swiss Webster mice weighing 18–22 g (Harlan Sprague-Dawley, Indianapolis, IN, USA) were housed under controlled conditions that have been shown to prevent cross-contamination of organisms from one treatment group to another Citation[19]. Mice were killed by cervical dislocation. Experiments were performed according to the National Institutes of Health guidelines. The University of Minnesota Institutional Animal Care and Use Committee approved all protocols.
Mice were orally inoculated (feeding needle) with 0.1 ml containing saline suspensions of 107 (unless otherwise stated) C. albicans or C. glabrata, or mixtures of C. albicans and C. glabrata. To alter the populations of intestinal bacteria, mice were given oral antibiotic(s) for 3 days prior to oral Candida, and antibiotic treatment was continued for the duration of the experiment. To eliminate all detectable cecal bacteria including strictly anaerobic bacteria, mice were given 1 mg/ml bacitracin, 2 mg/ml streptomycin sulfate, and 0.1 mg/ml gentamicin sulfate in the drinking water Citation[19]. To selectively eliminate aerobic/facultative gram-negative bacteria, mice were given 2 mg/ml streptomycin Citation[20]. To induce intestinal Escherichia coli overgrowth, mice were given 1 mg/ml bacitracin and 2 mg/ml streptomycin before oral inoculation with 108 streptomycin-resistant E. coli M21 Citation[20]. Eight in vivo models used in this study are summarized in . Viable microbes were cultured quantitatively from the MLN and ceca of all mice. Tissues were homogenized, serially diluted, plated on agar media, and incubated at 35°C for 48 h. Agar media included colistin-nalidixic acid agar supplemented with 5% sheep red blood cells for selective isolation of gram-positive bacteria and yeast, MacConkey agar for selective isolation of gram-negative bacilli, and CHROMagar Candida (Becton Dickinson Microbiology Systems, Cockeysville, MD, USA) to distinguish C. albicans (green colonies) from C. glabrata (rose colonies). Lower limits of assay detection were 3.0 log10 microbes per gram of cecum and 10 microbes per MLN. Microbes were identified by standard techniques.
Table I. Summary of eight experimental mouse models describing cecal bacterial flora, orally inoculated Candida species, numbers of mice per treatment group, and where data describing Candida colonization are presented.*
Statistical analyses were performed using StatView 5.0.1 (SAS Institute, Cary, NC, USA). Invivo experiments were replicated on two or three separate days to verify reproducibility, and data were pooled prior to analysis. Numbers of cecal microbes were converted to log10 and analyzed by unpaired Mann-Whitney (two groups) or by Kruskal-Wallis followed by unpaired Mann-Whitney post hoc (more than two groups). In vitro data were analyzed by unpaired Student's t test (two groups) or by analysis of variance followed by Fisher's test for significant difference (more than two groups). Statistical significance was p<0.05.
Results
Comparative colonization of C. glabrata and wild-type C. albicans
Mice were inoculated orally with 107C. albicans CAF2 or C. glabrata or both, and cecal flora was characterized 24 h later. This time point was chosen because, in mice with a normal intestinal flora, orally inoculated microbes are typically eliminated quickly, with maximal recovery at 24 h. All treatment groups had similar concentrations of cecal gram-negative and gram-positive bacteria, and C. albicans and C. glabrata were recovered only from mice inoculated with the corresponding yeast (A). There was no difference in the persistence of cecal C. albicans CAF2 and C. glabrata in mice inoculated with pure cultures, but in mice given both Candida spp., C. albicans was decreased compared with C. glabrata.
Figure 1. Cecal flora after oral inoculation of 107C. albicans CAF2 (wild type), or 107C. glabrata, or both Candida spp. into untreated mice (A) and into mice pretreated with bacitracin, streptomycin, and gentamicin to eliminate detectable cecal bacteria (B). The lower limit of assay detection is 3.0 log10/g indicated by a horizontal line. Bars with the same letters (a or b) differ at p≤0.05 and bars with the same letters (c, d, or e) differ at p≤0.01.
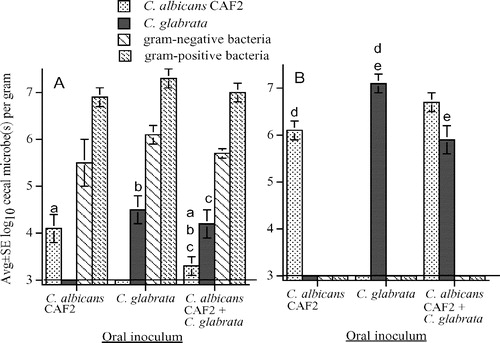
To enhance Candida cecal colonization, bacitracin, streptomycin, and gentamicin were used to eliminate detectable cecal bacteria Citation[19], Citation[21], and mice were killed 3 days after oral Candida administration. In mice given 107C. glabrata or C. albicans CAF2, cecal C. glabrata was recovered in 10-fold higher (p<0.05) numbers (107.2/g) compared with C. albicans (106.2/g) (B). In mice given both Candida spp., C. glabrata was decreased compared with C. albicans, but this difference was not significant (p=0.08). To verify that recovery of 107.2/g C. glabrata and 106.2/g C. albicans CAF2 represented intestinal colonization (with active fungal replication) of the 107 inoculated yeast, additional groups of antibiotic-treated mice were orally inoculated with 105C. albicans CAF2 or 105C. glabrata, and 5.8±0.5/g C. albicans and 7.2±0.2/g C. glabrata (avg log10±SE, n=8, p<0.01) were recovered; here, enlarged ceca weighed approximately 1 g, so these concentrations clearly reflected in vivo replication of the oral inoculum.
In the above experiments, each treatment group contained four mice and experiments were replicated two or three times. MLN were cultured to monitor extra-intestinal microbial dissemination. Thus, MLN were cultured from 24 otherwise untreated mice that had been orally inoculated with Candida, and 52 mice given antibiotics before oral inoculation. Microbes were recovered from only three MLN, i.e. 30 E. coli from each of two mice not given antibiotics (one mouse orally inoculated with 107C. glabrata and one mouse inoculated with 107C. glabrata plus 107C. albicans CAF2) and 10 C. albicans CAF2 from one mouse pretreated with antibiotics before oral inoculation with 107C. albicans CAF2.
Clinical isolates (C. glabrata 3328-7 and C. albicans YA062) were used to verify that C. glabrata replicated equally well or even better than C. albicans in the ceca of mice pretreated with bacitracin, streptomycin, and gentamicin. Concentrations (avg log10±SE, n=8) of cecal C. albicans (6.7±0.2) and C. glabrata (7.0±0.3) were similar in mice orally inoculated with pure cultures, but the concentration of C. glabrata (7.6±0.1) was higher (p < 0.01) in mice simultaneously inoculated with C. albicans (5.6±0.3).
Comparative colonization of C. glabrata and a filamentation-defective mutant C. albicans
To clarify the role of filamentation in the abilities of C. glabrata and C. albicans to persist in the mouse cecum, the above experiments were repeated, substituting C. albicans HLC54 (defective in filament formation) for wild-type C. albicans CAF2. Again, mice had similar concentrations of indigenous cecal bacteria, and C. albicans and C. glabrata were recovered only from mice given the corresponding Candida sp. (A). There were no significant differences in the abilities of C. albicans HLC54 and C. glabrata to persist in the ceca of normal mice given pure cultures of these organisms (A). However, in mice that received both Candida spp., C. albicans was decreased compared with C. glabrata (A). Antibiotic-treated mice had no detectable cecal bacteria and concentrations of C. albicans or C. glabrata were similar following oral inoculation with pure cultures (B). In mice given a mixture of C. albicans and C. glabrata, cecal concentrations of each yeast were similar, but each was decreased compared with the corresponding organism inoculated as a pure culture; this decrease was significant with C. glabrata, and almost significant with C. albicans (p=0.06). These experiments included 24 mice not treated with antibiotics and 36 mice pretreated with antibiotics before oral inoculation with pure or mixed cultures of Candida, and microbes were not recovered from these 60 MLN cultures.
Figure 2. Cecal flora after oral inoculation of 107C. albicans HLC54 (defective in filament formation), or 107C. glabrata, or both Candida spp., into untreated mice (A) and into mice pretreated with bacitracin, streptomycin, and gentamicin to eliminate detectable cecal bacteria (B). The lower limit of assay detection is 3.0 log10/g indicated by a horizontal line. Bars with the same letters (a or f) differ at p≤0.05 and bars with the same letters (b, c, d, or e) differ at p≤0.01.
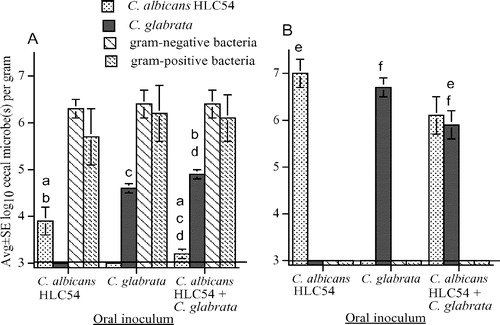
Cecal colonization of C. albicans and C. glabrata in mice with antibiotic-induced alterations of selected populations of intestinal bacteria
To assess the effect of E. coli overgrowth on intestinal colonization with Candida, mice were pretreated with bacitracin and streptomycin before oral inoculation with streptomycin-resistant E. coli mixed with C. albicans CAF2, C. albicans HLC54, or C. glabrata. This antibiotic cocktail typically eliminates all detectable bacteria including anaerobes, facilitating overgrowth with streptomycin-resistant E. coliCitation[20]. Ceca had no detectable bacteria other than high numbers of streptomycin-resistant E. coli (A). Numbers of cecal C. glabrata and C. albicans CAF2 were relatively low, and each was recovered in higher numbers (p<0.01) compared to the undetectable C. albicans HLC54 (A). Ten C. glabrata were recovered from the MLN of 1 of 8 mice orally inoculated with this yeast, and 10–30 streptomycin-resistant E. coli per MLN were recovered from 6 of 8 (75%) mice in each of these 3 treatment groups.
Figure 3. Cecal flora of mice pretreated with streptomycin and bacitracin (to eliminate detectable cecal bacteria) followed by oral inoculation with streptomycin-resistant E. coli mixed with either wild-type C. albicans CAF2, mutant C. albicans HLC54, or C. glabrata (A), and cecal flora of mice pretreated with streptomycin (to selectively eliminate aerobic/facultative gram-negative bacteria) before oral inoculation with wild-type C. albicans CAF2, mutant C. albicans HLC54, or C. glabrata (B). The lower limit of assay detection is 3.0 log10/g indicated by a horizontal line. Error bars not apparent if < 0.1. Bars with the same letters (a or b) differ at p < 0.01.
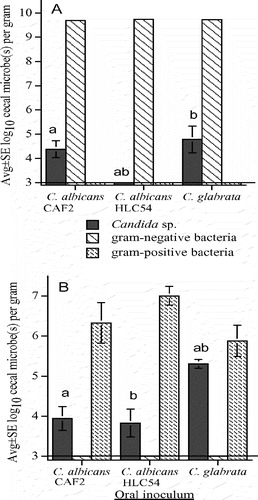
To selectively eliminate cecal gram-negative bacilli, mice were pretreated with streptomycin and killed 2 days after oral inoculation with a pure culture of C. albicans CAF2, C. albicans HLC54, or C. glabrata. Cecal aerobic/facultative gram-negative bacteria were not detected and there were no significant differences in the concentrations of aerobic/facultative gram-positive bacteria (B). Numbers of cecal C. glabrata were 23-fold and 30-fold higher than C. albicans CAF2 and HLC54, respectively. MLNs had no detectable microbes, except 20 C. glabrata were recovered from 1 of 8 mice inoculated with this yeast.
Comparative in vitro and in vivo growth of C. glabrata and C. albicans
The generation times of C. albicans CAF2, C. albicans HLC54, and C. glabrata in broth culture did not differ significantly and were 59±5 min, 67±4 min, and 57±4 min, respectively (avg±SE, n=3; p=0.3). However, if C. glabrata was incubated with C. albicans CAF2 or HLC54, C. glabrata inhibited both C. albicans CAF2 and C. albicans HLC54 (). After 24 h in mixed culture with strain CAF2 or HLC54, C. glabrata accounted for 80% and 72%, respectively, of the total yeast cells. And, compared with the corresponding C. albicans strain in pure culture, co-culture with C. glabrata resulted in a 2.5-fold decrease in strain CAF2 and a 3-fold decrease in strain HLC54. Growth of C. glabrata was not affected by co-culture with C. albicans CAF2 or HLC54 ().
Table II. Effect of C. glabrata on 24-h growth of C. albicans CAF2 (wild type) and HLC54 (filamentation-defective mutant) in broth culture following inoculation of 105/ml of each yeast.
presents a summary of the data in , 2, 3 and , showing how the relative concentrations of C. glabrata, C. albicans CAF2, and C. albicans HLC54 varied in different environments. Under all conditions tested, C. glabrata was either not inhibited by the presence of C. albicans, or C. glabrata had a competitive advantage and inhibited the persistence or growth of C. albicans.
Table III. Summary of statistically significant differences in the concentrations of C. glabrata compared to C. albicans CAF2 and C. albicans HLC54, following in vitro inoculation (broth culture) and in vivo inoculation (normal and antibiotic-treated mice) of pure and mixed cultures.
Discussion
In humans with a normal intestinal flora, Candida spp. are recovered in relatively low numbers of no more than 3.3 log10 per gram of feces Citation[22]. Although antibiotic-induced alterations in bacterial flora are known to facilitate Candida overgrowth, little is known about the modulatory effects of selected bacterial populations. This work represents an initial description of the ability of C. glabrata to persist and colonize the mouse intestine, including the ability of C. glabrata to compete with C. albicans, as well as the ability of E. coli cecal overgrowth to control the persistence of both C. glabrata and C. albicans.
There were no significant differences in the abilities of pure cultures of C. glabrata and C. albicans (both wild-type strain CAF2 and filamentation-defective strain HLC54) to persist in the ceca of normal mice (A and 2A). Surprisingly, when inoculated together, C. glabrata had a positive competitive advantage over both C. albicans strains, and the ability of C. albicans to form filaments did not appear to play a critical role in the inter-species competition between C. glabrata and C. albicans. Comparable results were obtained with broth cultures, where C. glabrata inhibited growth of both C. albicans CAF2 and HLC54 (). Some C. glabrata strains secrete a ‘killer toxin’ that inhibits microbial growth Citation[23], and C. glabrata might have secreted a substance that inhibited replication of C. albicansin vitro, although it would have been technically challenging to determine if such a mechanism was active in vivo.
Compared with mice with a normal bacterial flora, antibiotic-induced elimination of cecal bacteria facilitated colonization with pure cultures of C. glabrata and C. albicans (B and 2B). In mice inoculated with pure cultures of Candida spp., C. glabrata colonized the cecum in 10-fold higher numbers compared with wild-type C. albicans CAF2, but in similar numbers compared to the filamentation-defective mutant HLC54. If considered alone, these observations would suggest that the ability to form filaments was associated with decreased colonization of C. albicans compared with C. glabrata. However, following oral inoculation of mixed cultures into these antibiotic-treated mice, C. glabrata did not appear to have a competitive advantage over C. albicans CAF2 or HLC54. Thus, the inter-species competition between C. glabrata and C. albicans differed in normal and antibiotic-treated mice, indicating that the presence of a bacterial flora played a direct or indirect role in the outcome of inter-species competition. Kirkpatrick et al. Citation[24] reported that C. albicans had a competitive advantage over C. dubliniensis in broth culture under conditions favoring growth as a biofilm, but C. dubliniensis had a competitive advantage over C. albicans in the presence of a structure to support biofilm formation, namely polyvinyl chloride tubing. Thus, others have noted that environmental differences play an important role in inter-species competition in vitro and the present experiments indicated that environmental differences also modulate inter-species competition in vivo.
Because patients with systemic candidiasis often have antibiotic-induced alterations in selected populations of intestinal bacteria Citation[6], pure cultures of Candida spp. were orally inoculated into mice with streptomycin-induced elimination of aerobic/facultative gram-negative bacteria (B). (Streptomycin preserves the anaerobic microflora, noted to play a key role in limiting microbial colonization in the intestinal tract [20].) Compared with mice with no detectable cecal bacteria (B and 2B), concentrations of cecal Candida spp. were relatively low, but C. glabrata was increased compared with C. albicans CAF2 and HLC54. C. albicans CAF2 and HLC54 were recovered in similar numbers, again indicating that the ability to form filaments did not play a critical role in persistence of cecal C. albicans. Concentrations of cecal C. albicans and C. glabrata were comparable to normal mice, implying that absence of aerobic/facultative gram-negative bacteria did not facilitate colonization of Candida spp.
We previously reported that parenteral injection of E. coli lipopolysaccharide increased the concentrations of cecal C. albicans HLC54, but not CAF2, in antibiotic-treated mice Citation[21]. Thus, it was interesting to note how cecal overgrowth with viable E. coli affected Candida colonization (A). In these mice, streptomycin-resistant E. coli and Candida spp. were the only detectable cecal microbes, and Candida spp. were recovered in relatively low (or undetectable) numbers. Thirty years ago, Hummel et al. Citation[25] reported that E. coli can inhibit both in vitro and in vivo growth of C. albicans, and the present study confirmed these initial observations and extended them to include C. glabrata. The mechanism by which E. coli limited Candida colonization deserves further study, but this observation is consistent with Gumbo et al. Citation[10] who failed to find E. coli as a co-pathogen with Candida spp. in 28 patients with polymicrobial fungemia. Nonetheless, as expected Citation[20], the high level of E. coli cecal overgrowth was associated with extra-intestinal dissemination to the MLN of the majority of mice, but Candida sp. was not recovered from any of these MLN, indicating that active E. coli dissemination did not facilitate Candida dissemination.
Although the in vitro generation times of C. glabrata, C. albicans CAF2, and C. albicans HLC54 were similar, this had little relevance to the differing climax populations of these yeast in vivo. This observation could have broad implications. In analyzing the pathogenic effects of specific gene mutations in C. albicans, it has often been assumed that if mutant and parent strains have similar growth rates in vitro, then differences in virulence after intravenous injection (assessed by mortality or by fungal burden in organs such as kidney or liver) must be due to the genetic mutation under study. However, if the climax population levels of a given strain differ in different environments, then the doubling time in vitro could have little relevance to the resulting fungal burden in vivo. In the present study, C. albicans CAF2 and C. glabrata had similar growth rates in vitro, yet pure cultures of these organisms achieved different cecal population levels in some mouse models. In a previous study using antibiotic-treated immunosuppressed mice, both the incidences of translocation to the MLN and liver, and the Candida concentration in the liver, were greater with C. albicans HLC54 than CAF2 Citation[19]. Thus, the in vitro growth rate has little relevance to the resulting in vivo tissue burden, and the in vivo environment appears to play an important role in modulating the resulting fungal burden.
Conclusions
The results of this study can be summarized as follows. (a) Both C. albicans and C. glabrata persisted in the ceca of normal mice and colonization was facilitated by antibiotic-induced elimination of cecal bacteria. (b) Like C. albicans, C. glabrata did not readily disseminate from the intestinal tract, even in mice with high cecal concentrations. (c) Selective elimination of aerobic/facultative gram-negative bacteria did not facilitate cecal colonization with C. albicans or C. glabrata. (d) Compared with mice with no detectable cecal bacteria, E. coli overgrowth was effective in limiting colonization of both C. albicans and C. glabrata. (e) The ability of C. albicans to form filaments did not appear to play a critical role in cecal colonization, or in the ability of C. glabrata to compete with C. albicans. (f) In all in vitro (broth culture) and in vivo models (normal and antibiotic-treated mice) studied, C. glabrata persisted and/or replicated equally well or better than C. albicans. Thus, assuming that data from experimental mice are applicable to humans, the greater frequency of C. albicans compared with C. glabrata infections in high-risk patients is likely not related to an increased ability of C. albicans to colonize the intestinal tract.
This work was supported in part by the USA Public Health Service grant GM059221 from the National Institutes of Health and Grant 6-FY04-53 from the USA March of Dimes Birth Defects Foundation.
References
- Edmond MB, Wallace SE, McClish DK, Pfaller MA, Jones RN, Wenzel RP. Nosocomial bloodstream infections in United States hospitals: a three year analysis. Clin Infect Dis 1999; 29: 239–44
- Cole GT, Halawa AA, Anaissie EJ. The role of the gastrointestinal tract in hematogenous candidiasis: from laboratory to the bedside. Clin Infect Dis 1996; 22(Suppl 2)S73–S88
- White MH. Epidemiology of invasive candidiasis: recent progress and current controversies. Int J Infect Dis 1997; 1(Suppl 1)S7–S10
- Vincent JL, Anaissie E, Bruining H, Demajo W, el-Ebiary M, Haber J, et al. Epidemiology, diagnosis, and treatment of systemic Candida infection in surgical patients under intensive care. Intensive Care Med 1998; 24: 206–16
- Rangel-Frausto, MS, Wiblin, T, Blumberg, HM, Saiman, L, Patterson, J, Rinaldi, M, et al. and the, NEMIS Study Group. National epidemiology of mycoses survey (NEMIS): variations in rates of bloodstream infections due to Candida species in seven surgical intensive care units and six neonatal intensive care units. Clin Infect Dis 1995;29:253–8.
- Fidel PL, Jr, Vazquez JA, Sobel JD. Candida glabrata: review of epidemiology, pathogenesis, and clinical disease with comparison to C. albicans. Clin Microbiol Rev 1999; 12: 80–96
- Fidel, PL, Jr. Section IV immunity. In:. RA Calderone, ed., Candida and candidiasis. Washington, DC: American Society for Microbiology Press, 2002:179–256.
- Csank C, Haynes K. Candida glabrata displays pseudohyphal growth. FEMS Microbiol Lett 2000; 189: 115–20
- Pfaller, NA, Jones, RN, Sader, HS, Messer, SA, Houston, MA, Coffman, S, et al. and the, Sentry Participant Group. Bloodstream infections due to Candida species: SENTRY antimicrobial surveillance program in North America and Latin America, 1997–1998. Antimicrob Agents Chemother 2000;44:747–51.
- Gumbo T, Isada CM, Hall G, Karafa T, Gordon SM. Candida glabrata fungemia: clinical features of 139 patients. Medicine 1999; 78: 220–7
- Vazquez JA, Dembry LM, Sanchez V, Vazquez MA, Sobel JD, Dmuchowsk C, et al. Nosocomial Candida glabrata colonization: an epidemiologic study. J Clin Microbiol 1998; 36: 421–6
- Brieland J, Essig D, Jackson C, Frank D, Loebenberg D, Menzel F, et al. Comparison of pathogenesis and host immune responses to Candida glabrata and Candida albicans in systemically infected immunocompetent mice. Infect Immun 2001; 69: 5046–55
- Blot S, Vandewoude K, Hoste E, Poelaert J, Colardyn F. Outcome in critically ill patients with candidal fungaemia: Candida albicans vs. Candida glabrata. J Hosp Infect 2001; 47: 308–13
- Hedderwick SA, Lyons MJ, Liu M, Vazquez JA, Kauffman CA. Epidemiology of yeast colonization in the intensive care unit. Eur J Clin Microbiol Infect Dis 2000; 19: 663–70
- Fonzi WA, Irwin MY. Isogenic strain construction and gene mapping in Candida albicans. Genetics 1993; 134: 717–28
- Lo H-J, Kohler JR, DiDomenico B, Loebenberg D, Cacciapuoti A, Fink GR. Nonfilamentous C. albicans mutants are avirulent. Cell 1997; 90: 939–49
- Kim AS, Garni RM, Bendel CM, Wells CL. Hypoxia and extraintestinal dissemination of Candida albicans yeast forms. Shock 2003; 19: 257–62
- Riggle PR, Andrutis KA, Chen X, Tzipori SR, Kumamoto CA. Invasive lesions containing filamentous forms produced by a Candida albicans mutant that is defective in filamentous growth in culture. Infect Immun 1999; 67: 3649–52
- Bendel CM, Hess DJ, Garni RM, Henry-Stanley M, Wells CL. Comparative virulence of Candida albicans yeast and filamentous forms in orally and intravenously inoculated mice. Crit Care Med 2003; 31: 501–7
- Wells CL, Feltis BA, Hanson DF, Jechorek RP, Erlandsen SL. Oral infectivity and bacterial interactions with mononuclear phagocytes. J Med Microbiol 1993; 38: 345–53
- Henry-Stanley MJ, Hess DJ, Erickson EA, Garni RM, Wells CL. Effect of lipopolysaccharide on virulence of intestinal Candida albicans. J Surg Res 2003; 113: 42–9
- Bernhardt HM, Knoke M. Mycological aspects of gastrointestinal microflora. Scand J Gastroenterol 1997; 222: 102–6
- Kandel JS, Stern TA. Killer phenomenon in pathogenic yeast. Antimicrob Agents Chemother 1979; 15: 568–71
- Kirkpatrick ER, Lopez-Ribot JL, Mcatee RK, Patterson TF. Growth competition between Candida dubliniensis and Candida albicans under broth and biofilm growing conditions. J Clin Microbiol 2000; 38: 902–4
- Hummel RP, Maley MP, Miskell PW, Altemeier WA. Suppression of Candida albicans by Escherichia coli. J Trauma 1975; 15: 413–18