Abstract
α-Hemolytic streptococci represent a numerically significant fraction of human commensal oropharyngeal bacteria and various species have preferences for particular surfaces. On the basis of the production of neuraminidase, β1-N-acetylglucosaminidase, and β1-N-acetylgalactosaminidase we identified four phenotypes of Streptococcus mitis biovar 1, a pioneer in the human mouth. Isolates contained in these four groups differed in their ability to produce IgA1 protease and to bind salivary α-amylase, two properties proposed as ecological determinants for viridans streptococci. The purpose of our study was to determine whether production of these glycosidases, IgA1 protease activity, and salivary α-amylase binding conferred an advantage on S. mitis biovar 1 for soft and hard tissue colonization. We studied 3330 isolates of S. mitis biovar 1 obtained from buccal mucosa, dorsum of tongue and labial surfaces of the primary central incisors of four infants on eight or nine visits from birth to 1 year of age. Isolates producing neuraminidase predominated and showed a preference for colonizing mucosal surfaces. In contrast, the production of IgA protease and/or salivary α-amylase binding did not confer an ecological advantage. While isolates producing neuraminidase were predominant, the production of this enzyme was not required for successful colonization, since neuraminidase-negative isolates were consistently recovered from the infants’ mouths, albeit in lower numbers. Clearly, physiological properties required for successful colonization of the mouth extend beyond the determinants examined in the current study. However, our data are a small first step in understanding properties that enable strains of S. mitis biovar 1 to colonize and persist in the human oral cavity.
Introduction
The genus Streptococcus represents a numerically significant fraction of the commensal bacteria found in the oropharynx of humans and other mammals Citation[1]. Clearly, streptococci are very efficient at adhering to host surfaces and are capable of binding to a large variety of substrates Citation[2]. Despite such promiscuous binding ability, different streptococcal species demonstrate tropism for various oropharyngeal surfaces Citation[3–7]. For example, Streptococcus pneumoniae inhabits the pharyngeal mucosa but is not found in the mouth, S. mitis biovar 1 inhabits both the buccal and pharyngeal mucosae, S. oralis inhabits the teeth, S. salivarius and S. mitis biovar 2 inhabit the tongue, while S. mutans inhabits non-shedding, dental surfaces, only. The oral substrata are coated with a thin film derived from saliva, and organisms attempting to colonize the mouth are, themselves, suspended in saliva. Thus, both host and microbial surfaces may be modified by salivary components such as α-amylase and secretory immunoglobulin A (SIgA), secreted microbial products, such as glucans and glucosyltransferases, plasma proteins derived from gingival crevicular fluid, and food components Citation[8–13].
α-Amylase is a principal component of human saliva and may reach concentrations exceeding 2.0 mg/ml Citation[14]. In addition to its function in digestion, α-amylase binds to certain oral bacteria and oral surfaces with high affinity and retains enzyme activity in bound form Citation[15]. Thus, depending on whether a bacterium encounters fluid phase or surface-bound α-amylase, the enzyme may aid in clearance or attachment of the organism, respectively. Among the viridans streptococci α-amylase has been reported to bind to S. gordonii, S. mitis, S. cristatus, and S. anginosus, but not to S. sanguinis, S. oralis, or S. mutansCitation[11], Citation[16], Citation[17]. Therefore, in addition to its possible role in facilitating adherence, the binding of α-amylase by certain species of viridans streptococci may aid in their differentiation Citation[10], Citation[16], Citation[17].
Since the principal mediator of adaptive humoral immunity at human mucosal surfaces is SIgA, bacteria synthesizing IgA1 proteases that cleave subclass 1 of this immunoglobulin have been considered to be at a selective advantage in colonizing mucosal surfaces Citation[18]. Among the streptococci in the oropharynx, other than S. pneumoniae, S. oralis, S. sanguinis and about two-thirds of the isolates of S. mitis biovar 1 produce IgA1 protease Citation[19–21]. Both S. mitis biovar 1 and S. oralis are pioneer species in the mouth and it has been proposed that the production of IgA1 protease may confer an ecological advantage on these bacteria Citation[19], Citation[21], Citation[22]. Interestingly, in addition to S. pneumoniae, S. oralis, some S. mitis biovar 1 and 2 strains, and S. intermedius produce neuraminidase, which is capable of removing terminal sialic acid from oligosaccharide side chains located at the hinge region and elsewhere along the α1 heavy chain Citation[20]. Once sialic acid is removed, exoglycosidases, such as β-galactosidase and N-acetyl-glucosaminidase, also can attack the carbohydrate moiety of the α1 heavy chain Citation[20]. It is significant that, while limited deglycosylation may increase the susceptibility of SIgA1 to IgA1 protease, more extensive deglycosylation of the hinge region decreases, and may eliminate, the susceptibility of SIgA1 to IgA1 protease Citation[19], Citation[20].
The purpose of our study was to determine whether production of neuraminidase, β1-N-acetylglucosaminidase, and β1-N-acetylgalactosaminidase, IgA1 protease, and salivary α-amylase binding conferred an advantage on S. mitis biovar 1 for oral soft and hard tissue colonization in infants from birth to 1 year of age.
Materials and methods
Study population
Four neonates (nos 3, 6, 8, and 10) were enrolled in the study without regard to race or sex after obtaining signed, informed consent from the infants’ mothers. Neonate–mother pairs were included in the study provided that they were full term (38–42 weeks of gestation), appropriate for gestational age and without underlying illness. Criteria that served to exclude neonates from participation in the study were perinatal complications of maternal fever, prolonged rupture of fetal membranes (> 24 h), and antibiotic treatment of mother or infant during the first 30 days of the infant's life. Also excluded from the study were infants with severe metabolic or infectious complications during the first 30 days post partum, and mothers who were HIV-positive and/or hepatitis B-positive. The study population comprised three males and one female, all of whom were breastfed for the first 3 months post partum. Two subjects were white (not of Hispanic origin), one was Hispanic and one was Asian. The Institutional Review Board of Georgetown University Medical Center approved the clinical protocol.
Sample collection, processing and culture
Swab samples of the oral mucosa were obtained from the infants, 1–3 days, 2 weeks, 4 weeks and 2, 4, 6, 8, 10, and 12 months post partum for a maximum of nine sampling points. Three of the infants missed the 2-week visit, giving a maximum eight sampling points. Mucosal samples were collected with swabs (BBL™ CultureSwab™, Collection & Transport System, Becton Dickinson and Co., Sparks, MD, USA). Two areas of the oral mucosa were sampled at each visit using separate swabs. The left and right buccal mucosae were sampled with one swab and the dorsum of the tongue was sampled with a second swab. As soon as teeth erupted (usually the lower central incisors), their labial surfaces were swabbed using a third swab. The swabs were transported to the laboratory and plated within 1 h of collection. The head of each swab was cut off with sterile scissors and dropped into an individual tube containing 2 ml of phosphate-buffered saline (PBS), pH 7.4. The bacteria were dispersed by ultrasound at 80 W for 10 s using a Branson Sonifier 250 (Branson Ultrasonics Corp., Danbury, CT, USA) equipped with a 3 mm diameter micro probe. The dispersed samples were serially diluted in PBS to 10–5 and immediately inoculated onto trypticase soy agar containing 5% sheep blood using an Autoplate 4000 spiral plater (Spiral Biotech, Bethesda, MD, USA). The plates were incubated in an atmosphere of 5% CO2 in air at 37°C for 48 h.
Sixty α-hemolytic colonies were picked at random from primary isolation plates of the cheek, tongue, and tooth samples containing, wherever possible, between 60 and 100 colonies and were subcultured to purity. Thus, 120 isolates were collected from pre-dentate infants and 180 isolates were collected from dentate infants at each visit. Subcultured strains were re-examined for α-hemolysis, stained by Gram's method, and tested for catalase production. α-Hemolytic, gram-positive, catalase-negative cocci were retained for further identification.
Identification of streptococci
Determinative tests used were fermentation of amygdalin, tagatose and glucose; production of ammonia from arginine; hydrolysis of esculin; production of neuraminidase, β1-N-acetylglucosaminidase, and β1-N-acetyl-galactosaminidase; α-amylase binding; IgA1 protease production; production of extracellular polysaccharide from sucrose; and optochin sensitivity. These tests were performed as described previously Citation[23] except where otherwise stated. In this study hydrolysis of esculin was determined in nutrient broth (Difco) containing 0.1% esculin and 0.05% ferric ammonium citrate. Results were read after incubation for 2 and 7 days. The production of extracellular polysaccharide from sucrose was performed in the sucrose broth described by Bailey and Oxford Citation[24]. Glucose broth was used as control. Polysaccharide was detected as described previously Citation[25]. Optochin sensitivity was determined using Taxo P discs (Becton Dickinson and Co.). Streptococcal binding of salivary α-amylase was determined as described previously Citation[16], Citation[17]. IgA1 protease activity was determined by assessing cleavage of an IgA1 paraprotein using an ELISA Citation[26] with the following modifications. The capture antibody coated to the wells of microtiter plates was rabbit anti-human light chains (Dako, Carpinteria, CA, USA). After blocking, 100 µl of the diluted substrate was added to each well for 1 h at RT. Following washing, a biotinylated mouse IgG1 monoclonal antibody to the Fc region of human IgA (clone HP6111B, Hydridoma Labs, Baltimore, MD, USA) was added to the wells as the reporter for 1 h at room temperature. After washing the wells were charged with horseradish peroxidase conjugated to streptavidin (Tago, Burlingame, CA, USA). O-Phenylenediamine served as the substrate. S. oralis ATCC 35037 incubated with the IgA1κ paraprotein served as the positive control and IgA1κ incubated with buffer served as the negative control. Cleavage was determined by reference to the absorbance values of the positive and negative controls. Absorbance was at or near background for isolates producing IgA1 protease. To confirm the assignment of isolates in the four phenotypic groups to S. mitis biovar 1, DNA–DNA hybridization and partial sequencing of the manganese-dependent superoxide dismutase gene (sodA) Citation[27] were performed. As it was not possible to perform these tests on every isolate, a sampling frame was created by assigning a unique number to each isolate and then 12 isolates from each phenotypic group were chosen using a computer generated random numbers list. Appropriate reference strains were also included.
Statistical analysis
To examine the preference of phenotype for site (buccal mucosa, tongue or teeth) a nonparametric one-way ANOVA with multiple comparison tests was employed. To examine patterns of colonization of the phenotypes by site over time repeated measures ANOVA with contrast statements was performed. The dependent variable for all analyses was transformed logarithmically. All analyses were performed using SAS software version 8.2 (SAS Institute, Cary, NC, USA).
Results
Of the 4440 isolates collected, 3330 (75%) that were unable to hydrolyse arginine and esculin, ferment amygdalin and tagatose and were resistant to optochin were retained for further examination. Of these isolates 94.6% were assigned to S. mitis biovar 1 by virtue of their inability to produce extracellular polysaccharide from sucrose Citation[7]. Assignment to S. mitis biovar 1 was confirmed by examination of a randomly selected subset of isolates by DNA–DNA hybridization and partial manganese-dependent superoxide dismutase gene (sodA) sequencing. Based on the production of neuraminidase, β1-N-acetyl-glucosaminidase, and β1-N-acetylgalactosaminidase, 3144 (94.4%) of these 3330 isolates could be divided into four large phenotypic groups (). Group 1 (0-0-0), comprised isolates that did not produce any of the three glycosidases (233 isolates); group 2 (0-1-1), isolates that did not produce neuraminidase, but produced β1-N-acetylglucosaminidase and β1-N-acetylgalactosaminidase (685 isolates); group 3 (1-0-0), isolates that produced neuraminidase, only (1172 isolates); and group 4 (1-1-1), isolates that produced all three glycosidases (1054 isolates). Significantly, isolates that produced neuraminidase (groups 3 and 4) predominated, together comprising over two-thirds (70.8%) of the total number of isolates assigned to the four phenotypic groups. Furthermore, isolates producing neuraminidase had a statistically significant preference for the shedding surfaces (cheeks and tongue) compared with the non-shedding surfaces of the teeth ().
Table I. Division of S. mitis biovar 1 into four groups based on production of neuraminidase, β1-N-acetylglucosaminidase and β1-N-acetylgalactosaminidase and their distribution on cheeks, tongue and teeth.
Table II. Preference of phenotypes of S. mitis biovar 1 for buccal mucosa, tongue and teeth.
Because the abilities to bind α-amylase and to cleave human IgA1 have been proposed as ecological determinants of viridans streptococci Citation[11], Citation[16], Citation[24], Citation[26], Citation[27], these properties were also evaluated in each of the four phenotypic groups. Despite the fact that group 1 (0-0-0) and group 2 (0-1-1) isolates comprised only 29.2% of the S. mitis biovar 1 isolates included in the four phenotypic groups, the percentages of isolates binding salivary α-amylase in these groups was not significantly different from those of the numerically significant groups, 3 (1-0-0) and 4 (1-1-1).
All four phenotypic groups contained isolates that produced IgA1 protease and, overall, 54% of the isolates produced this enzyme. However, the percentages of strains positive for IgA1 protease differed markedly between the groups. In group 4 (1-1-1), 91%, and in group 1 (0-0-0), 80% of the isolates produced IgA1 protease, whereas in group 2 (0-1-1) only 12% and in group 3 (1-0-0) only 39% of the isolates produced this enzyme. We were interested to determine whether there were any patterns of colonization by each of the phenotypes by site (cheek, tongue, and teeth) over time in these infants. These data are arrayed by phenotype in and by site in . In order to look for temporal patterns of colonization, we used repeated measures analysis of variance. However, although there was the suggestion of some patterns of colonization over time by phenotype, few statistically significant differences were found, most likely due to the low number of observations resulting from the small number of infants examined.
Figure 1. Percentage of total S. mitis biovar 1 by phenotype, site and visit. Visits 1–9 correspond to 1–3 days, 2 weeks, 4 weeks and 2, 4, 6, 8, 10, and 12 months post partum, respectively. Group 1 (0-0-0 phenotype); group 2 (0-1-1 phenotype); group 3 (1-0-0 phenotype); group 4 (1-1-1 phenotype).
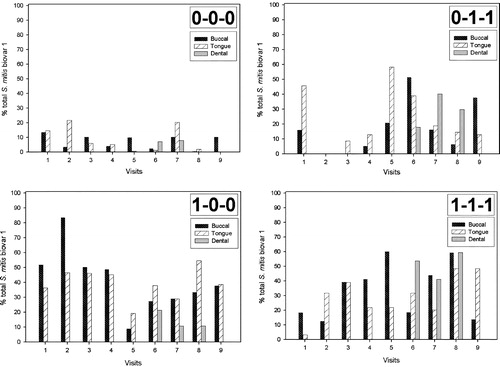
Figure 2. Percentage of total S. mitis biovar 1 by site, phenotype and visit. Visits 1–9 correspond to 1–3 days, 2 weeks, 4 weeks and 2, 4, 6, 8, 10, and 12 months post partum, respectively. Dentate infants were not sampled at visit no. 9. Group 1 (0-0-0 phenotype); group 2 (0-1-1 phenotype); group 3 (1-0-0 phenotype); group 4 (1-1-1 phenotype).
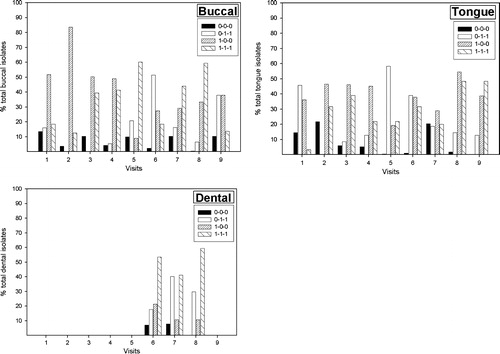
Discussion
The mouth is unique in the human body in the variety of surfaces available for colonization by commensal bacteria. Not only does it contain both keratinized and non-keratinized smooth and papillated desquamating epithelia, but also enamel tooth surfaces that are abiotic and non-shedding. In addition, the gingival epithelium forms a cuff around the base of the crowns of the teeth, forming a sulcus that is a protected environment not subject to the mechanical forces of mastication and salivary flow. The tropism of viridans streptococci for these various oropharyngeal surfaces likely results from the interaction of specific bacterial surface adhesins with complementary receptors found in the acquired films that coat oral surfaces. These films are derived principally from the selective binding of salivary glycoproteins, but also crevicular fluid constituents and secreted bacterial products Citation[2], Citation[11].
α-Amylase is a principal component of saliva and is found incorporated into the acquired films, termed pellicles, that adsorb to mucosal Citation[28] and tooth surfaces Citation[12], Citation[13], Citation[29] that are exposed to saliva in the mouth. Consistent with the observation that α-amylase is a component of the enamel pellicle, the species of viridans streptococci that are early colonizers of tooth surfaces bind α-amylase and amylase-binding streptococci are numerous in supragingival plaque Citation[10], Citation[30]. Streptococci binding α-amylase have been identified as S. gordonii, S. mitis, S. cristatus, and S. anginosus, although the ability to bind α-amylase does not appear to be a uniform property of these species Citation[11], Citation[16], Citation[17]. While the ability of streptococci to bind α-amylase appears to confer an advantage for binding to non-shedding tooth surfaces, the same does not appear to be the case for binding to shedding mucosal surfaces. If the ability to bind α-amylase confers an ecological advantage on S. mitis biovar 1 for colonization then it would be expected that this trait would be found disproportionately in phenotypic groups 3 and 4 that were numerically dominant. However, this was not the case. Indeed, the ability to bind α-amylase was not a uniform property of any of the four phenotypic groups and the percentage of isolates positive for this trait did not differ significantly between the phenotypic groups. These data are consistent with those of Hohwy et al. Citation[31], who failed to find an association between the ability to bind salivary α-amylase and persistence of genotypes of S. mitis biovar 1 in the mouth.
Since SIgA antibodies are the principal mediators of humoral adaptive immunity at mucosal surfaces and SIgA in the saliva of neonates is almost entirely of subclass 1 Citation[32], it has been hypothesized that the production of IgA1 protease by certain species of viridans streptococci aids in their colonization by allowing them to evade SIgA1-mediated immunity Citation[18]. In support of this contention is the finding that a high proportion of S. mitis biovar 1 isolated from neonates produce IgA1 protease Citation[23] and that, in adults, IgA1 protease-producing streptococci are primarily isolated from initial dental plaque and buccal mucosa Citation[3]. However, in contradistinction, similar proportions of IgA1 protease-producing streptococci were found in the initial dental plaque of individuals with selective IgA deficiency to those found in the initial dental plaque of normal individuals Citation[33]. Furthermore, as with α-amylase binding, IgA1 protease activity was not a preferential characteristic of persistent clones of S. mitis biovar 1 Citation[31]. In the current study, while 91% of isolates in phenotypic group 4 (one of the two numerically dominant phenotypic groups) produced IgA1 protease, this enzyme was produced by only 39% of the other dominant phenotypic group (group 3). Moreover, although group 1 isolates were numerically insignificant, representing only 7.4% of the total, 80% of these isolates produced IgA1 protease.
One property that was significantly correlated with the ability of S. mitis biovar 1 to colonize mucosal surfaces was the production of neuraminidase. This is particularly interesting in light of the established role of neuraminidase in nasopharyngeal colonization Citation[34], Citation[35] and in the pathogenesis of S. pneumoniaeCitation[36], a viridans streptococcus that is extremely closely related to S. mitis biovar 1 at the level of 16S ribosomal RNA Citation[37]. It appears likely that neuraminidase acts to remove terminal sialic acid from glycoconjugates on the surface of epithelial cells or from mucin in the acquired film covering the mucosa to reveal neo-epitopes that serve as receptors for bacterial adhesins Citation[38]. In addition, S. pneumoniae and other neuraminidase-producing viridans streptococci have been shown to desialyate host defense factors such as IgA of both subclasses, secretory component and lactoferrin, and in so doing impair their effector functions Citation[19], Citation[20], Citation[39].
In a recent study of the population dynamics of S. mitis in the oropharynx Citation[31], although the ability of genotypes to persist over long periods of time was not randomly distributed in the population, it was not correlated with the abilities to bind α-amylase and/or to produce IgA1 protease. Our results are in accordance with these findings. While isolates that produced neuraminidase were most numerous, the production of this enzyme was not required for successful colonization of the mouths of the infants since neuraminidase-negative isolates were consistently recovered, albeit at lower numbers than isolates that produced this enzyme.
It is clear that the physiological properties required for successful colonization of the oral cavity extend beyond the limited range of determinants that have been examined in the current study, as there were hardly any differences in the temporal patterns of colonization by site between the phenotypic groups. What renders one particular strain more fit to occupy a niche than another is important in understanding the commensal–host relationship and is the subject of ongoing investigations.
This work was supported by Public Health Service grant DE08171 from the National Institute of Dental and Craniofacial Research. G.H.B. is supported by grant MT 7611 from the Medical Research Council of Canada. The authors wish to thank Manju Chauhan and Shelley Tunwall for excellent technical assistance.
References
- Hardie JM, Bowden GH. The normal microbial flora of the mouth. The normal microbial flora of man, FA Skinner, JG Carr. Academic Press, London 1974; 47–83
- Jenkinson HF, Lamont RJ. Streptococcal adhesion and colonization. Crit Rev Oral Biol Med 1997; 8: 175–200
- Frandsen EV, Pedrazzoli V, Kilian M. Ecology of viridans streptococci in the oral cavity and pharynx. Oral Microbiol Immunol 1991; 6: 129–33
- Carlsson J, Grahnen H, Jonsson G, Wikner S. Early establishment of Streptococcus salivarius in the mouth of infants. J Dent Res 1970; 49: 415–18
- Carlsson J. Presence of various types of non-haemolytic streptococci in dental plaque and in other sites of the oral cavity in man. Odontol Revy 1967; 18: 55–74
- Carlsson J, Grahnen H, Jonsson G, Wikner S. Establishment of Streptococcus sanguis in the mouths of infants. Arch Oral Biol 1970; 15: 1143–8
- Kononen E, Jousimies-Somer H, Bryk A, Kilp T, Kilian M. Establishment of streptococci in the upper respiratory tract: longitudinal changes in the mouth and nasopharynx up to 2 years of age. J Med Microbiol 2002; 51: 723–30
- Vacca-Smith AM, Venkitaraman AR, Schilling KM, Bowen WH. Characterization of glucosyltransferase of human saliva adsorbed onto hydroxyapatite surfaces. Caries Res 1996; 30: 354–60
- Vacca-Smith AM, Venkitaraman AR, Quivey RG, Jr, Bowen WH. Interactions of streptococcal glucosyltransferases with alpha-amylase and starch on the surface of saliva-coated hydroxyapatite. Arch Oral Biol 1996; 41: 291–8
- Scannapieco FA, Torres G, Levine MJ. Salivary α-amylase: role in dental plaque and caries formation. Crit Rev Oral Biol Med 1993; 4: 301–7
- Scannapieco FA. Saliva-bacterium interactions in oral microbial ecology. Crit Rev Oral Biol Med 1994; 5: 203–48
- Orstavik D, Kraus FW. The acquired pellicle: immunofluorescent demonstration of specific proteins. J Oral Pathol 1973; 2: 68–76
- Orstavik D, Kraus FW. The acquired pellicle: enzyme and antibody activities. Scand J Dent Res 1974; 82: 202–5
- Aguirre A, Levine MJ, Cohen RE, Tabak LA. Immunochemical quantitation of α-amylase and secretory IgA in parotid saliva from people of various ages. Arch Oral Biol 1987; 32: 297–301
- Scannapieco FA, Bergey EJ, Reddy MS, Levine MJ. Characterization of salivary α-amylase binding to Streptococcus sanguis. Infect Immun 1989; 57: 2853–63
- Douglas CWI, Pease AA, Whiley RA. Amylase-binding as a discriminator among oral streptococci. FEMS Microbiol Lett 1990; 66: 193–7
- Kilian M, Nyvad B. Ability to bind salivary α-amylase discriminates certain viridans streptococcal species. J Clin Microbiol 1990; 28: 2576–7
- Kilian M, Reinholdt J, Lomholt H, Poulsen K, Frandsen EV. Biological significance of IgA1 proteases in bacterial colonization and pathogenesis: critical evaluation of experimental evidence. APMIS 1996; 104: 321–38
- Cole MF, Evans M, Fitzsimmons SP, Johnson J, Pearce C, Sheridan MJ, et al. Pioneer oral streptococci produce immunoglobulin A1 protease. Infect Immun 1994; 62: 2165–8
- Reinholdt J, Tomana M, Mortensen SB, Kilian M. Molecular aspects of immunoglobulin A1 degradation by oral streptococci. Infect Immun 1990; 58: 1186–94
- Kilian M, Holmgren K. Ecology and nature of immunoglobulin A1 protease-producing streptococci in the human oral cavity and pharynx. Infect Immun 1981; 31: 868–73
- Kilian M, Reinholdt J, Nyvad B, Frandsen EV, Mikkelsen L. IgA1 proteases of oral streptococci: ecological aspects. Immunol Invest 1989; 18: 161–70
- Pearce C, Bowden GH, Evans M, Fitzsimmons SP, Johnson J, Sheridan MJ, et al. Identification of pioneer viridans streptococci in the oral cavity of human neonates. J Med Microbiol 1995; 42: 67–72
- Bailey RW, Oxford AE. Pre-requisites for dextran production by Streptococcus bovis. Nature (Lond) 1958; 182: 185–6
- Hehre EJ, Neill JM. Formation of serologically reactive dextrans by streptococci from subacute bacterial endocarditis. J Exp Med 1946; 83: 147–62
- Reinholdt J, Kilian M. A sensitive enzyme-linked immunosorbent assay for IgA protease activity. J Immunol Methods 1983; 63: 367–76
- Kawamura Y, Whiley RA, Shu S-E, Ezaki T, Hardie JM. Genetic approaches to the identification of the mitis group within the genus Streptococcus. Microbiology 1999; 145: 2605–13
- Bradway SD, Bergey EJ, Scannapieco FA, Ramasubba N, Zawacki S, Levine MJ. Formation of salivary-mucosal pellicle: the role of transglutaminase. Biochem J 1992; 284: 557–64
- Al-Hashimi I, Levine MJ. Characterization of in vivo salivary-derived enamel pellicle. Arch Oral Biol 1989; 34: 289–95
- Scannapieco FA, Soloman L, Wadenya RO. Emergence in human dental plaque and host distribution of amylase-binding streptococci. J Dent Res 1994; 73: 1627–35
- Hohwy J, Reinholdt J, Kilian M. Population dynamics of Streptococcus mitis in its natural habitat. Infect Immun 2001; 69: 6055–63
- Fitzsimmons SP, Evans MK, Sheridan MJ, Wientzen R, Cole MF. Immunoglobulin A subclasses in infant's saliva and in saliva and milk from their mothers. J Pediatr 1994; 124: 566–73
- Reinholdt J, Friman V, Kilian M. Similar proportions of immunoglobulin A1 (IgA1) protease-producing streptococci in initial dental plaque of selectively IgA-deficient and normal individuals. Infect Immun 1993; 61: 3998–4000
- Orihuela CJ, Gao G, Francis KP, Yu J, Tuomanen EI. Tissue specific contributions of pneumococcal virulence factors to pathogenesis. J Infect Dis 2004; 190: 1661–9
- Tong HH, Blue LE, James MA, DeMaria TF. Evaluation of the virulence of a Streptococcus pneumoniae neuraminidase-deficient mutant in nasopharyngeal colonization and development of otitis media in the chinchilla model. Infect Immun 2000; 68: 921–4
- Long JP, Tong HH, DeMaria TF. Immunization with native or recombinant Streptococcus pneumoniae neuraminidase affords protection in the chinchilla otitis media model. Infect Immun 2004; 72: 4309–13
- Kawamura Y, Hou XG, Sultana F, Miura H, Ezaki T. Determination of 16S rRNA sequences of Streptococcus mitis and Streptococcus gordonii and phylogenetic relationships among members of the genus Streptococcus. Int J Syst Bacteriol 1995; 45: 406–8
- Gibbons RJ, Hay DI, Childs WC 3rd, Davis G. Role of cryptic receptors (cryptitopes) in bacterial adhesion to oral surfaces. Arch Oral Biol 1990; 35(Suppl): 107S–114S
- King SJ, Hippe KR, Gould JM, Bae D, Peterson S, Cline RT, et al. Phase variable desialylation of host proteins that bind to Streptococcus pneumoniae in vivo and protect the airway. Mol Microbiol 2004; 54: 159–71