Abstract
Fat-soluble vitamin K is an essential component of the blood clotting process. Menaquinones are the naturally occurring form of vitamin K identified in bacteria. Lipid extracts were made from three bacteria originally isolated from the human neonatal gut and identified as Enterobacter agglomerans, Serratia marcescens and Enterococcus faecium. Following preparative thin layer chromatography (TLC), the lipid extracts were subjected to liquid chromatography-mass spectrometry (LC-MS) analysis. Peak analysis of the LC-MS data showed that the three bacteria produce various forms of menaquinone.
Introduction
Vitamin K is a generic term for a family of fat-soluble vitamins. Two of the naturally occurring quinone forms are phylloquinone (vitamin K1 or 2-methyl-3eicosa-2′-ene-1,4-naphthoquinone), which is obtained from plants, and menaquinone (vitamin K2 or 2-methyl-3-multiprenyl-1,4-naphthoquinone), which is produced by certain bacteria during anaerobic respiration Citation[1], Citation[2]. Menaquinone variants are differentiated on the basis of the number of isoprene-5-carbon prenyl units on their side chains. Chain length varies from 2 to 15 isoprene units Citation[3]. The variations in the structure of the menaquinones produced by bacteria have been used in bacterial classification Citation[4]. Several bacteria that have been isolated from the human intestine have previously been identified as producing menaquinones of various chain lengths and it is thought that these bacteria contribute to the vitamin K requirements of the human body Citation[5]. This paper discusses the identification of three previously unknown menaquinone-producing bacteria isolated from faecal samples of neonates.
Materials and methods
Cultures and cultivation
Bacterial strains were isolated from faecal samples obtained from neonates aged between birth and 6 weeks old in a study carried out previously within our laboratory Citation[6]. The isolates were identified using selective media, Gram stain and API tests. Several bacterial species were identified as producing vitamin K using the techniques as outlined in this paper; however, the data presented in this paper only relate to the three bacterial species Enterobacter agglomerans, Serratia marcescens and Enterococcus faecium. Purified cultures were grown in 1 litre Duran containing tryptone soy broth powder (Lab M) dissolved in deionized water with haemin (10 mg) and cysteine (0.05% w/v) Citation[3]. The cultures were incubated anaerobically at 37°C for 72 h. Cultures were checked for purity using Gram stain as well as selective and non-selective agar. The bacterial pellets were harvested in a Sorvall RC-5B centrifuge at 16 266 g for 10 min at 4°C. The pellet was washed three times in 20 ml volumes of 0.01 M sterile phosphate-buffered saline (PBS) and centrifuged again under the same conditions. Menaquinone 4 standard was purchased from Sigma, UK.
Extraction, purification and analysis of menaquinones
Extraction of suspected menaquinones from the wet bacterial pellets was carried out using the modified Bligh and Dyer method Citation[7] developed by Hammond and White Citation[8], which involves the extraction of total lipid content. Menaquinone 4 (currently the only commercially available menaquinone) was used as a standard, as menaquinones of different side chain lengths migrate similar distances on thin layer chromatographic (TLC) plates Citation[9]. The extracted menaquinones were then purified using the procedure as described by Fernandez and Collins Citation[9], on preparative silica gel GF254 prep TLC plates. The suspected menaquinones were viewed under a UV light box emitting light at 254 nm. Bands from the lipid extracts of the bacteria that were in line with the menaquinone 4 standard were removed and dissolved in dichloromethane:methanol (30:70), as was the menaquinone 4 standard, and all were subsequently analysed by mass spectrometry (MS). Liquid chromatography-MS (LC-MS) analysis was carried out using a Bruker Daltronics Esquire LC ion trap mass spectrometer with a Synergi Hydro C18 reverse phase (4.6 µm×250 mm) HPLC column (00G-4375-E0) supplied by Phenomenex (Cheshire, UK) using the conditions outlined in .
Table I. Conditions used for monitoring of menaquinones on the Bruker Esquire-LC Ion Trap LC/MS.
Results
Several Gram-positive and Gram-negative bacterial strains were identified in the study as vitamin K producers using the techniques as outlined in this paper. These included the strains Bacteroides ovatus, Enterobacter agglomerans, Enterococcus faecalis, Escherichia coli, Prevotella buccae, Staphylococcus capitis, Staphylococcus epidermidis, Staphylococcus haemolyticus and Staphylococcus warneri, all of which were isolated in faecal samples from breast-fed infants, while vitamin K production was also identified in the following strains isolated from infants fed exclusively on formula – Bacteroides sp., Citrobacter freundii, Enterococcus faecium, Serratia marcescens, Staphylococcus capitis and Staphylococcus warneri. However, the data presented in this paper only relate to the three bacterial species Enterobacter agglomerans, Serratia marcescens and Enterococcus faecium, which were selected as they have not previously been reported to produce vitamin K.
Previous studies have shown that menaquinones of differing side chain length give similar Rf values following TLC analysis Citation[7]. Therefore lipid extracts with bands which co-migrated with the menaquinone 4 standard (unpublished results) were subjected to further analysis to determine the exact menaquinone form produced by the respective bacteria being studied.
The LC-MS data obtained for the standard menaquinone 4 are presented in . Peak X, indicated in the total ion count shown in a, was further analysed, yielding the three significant peaks shown in b. The molecular weights of the three peaks in b correlated with the molecular weights of the protonated (mass = 445.3), sodiated (mass = 467.4) and potassiated (mass = 483.1) forms of menaquinone 4.
Figure 1. (a) Total ion count of a directly injected menaquinone 4 standard (6 µg/ml). (b) Mass spectrometric analysis of peak X in (a) detected at 13.8 min.
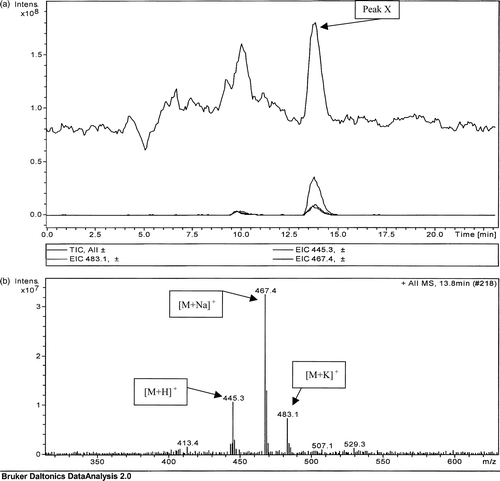
LC-MS analysis of the extract from Enterobacter agglomerans (a, peak A) showed molecular weight bands at 717.5 (protonated), 739.7 (sodiated) and 755.5 (potassiated) (b) correlating to the expected molecular weight for menaquinone 8 analogues. The sodiated analogue of menaquinone 8 forms the base peak analogue, as was detected for the menaquinone 4 standard (b).
Figure 2. (a) Extrapolated ion count for a TLC extract prepared from an Enterobacter agglomerans extract. (b) Mass spectrometric analysis of peak A detected at a retention time of 30 min.

In addition to E. agglomerans, a strain of Serratia marcescens was also found to produce menaquinone 8 (a). As with the analysis of E. agglomerans, the MS analysis showed molecular weights corresponding to the protonated, sodiated and potassiated forms and, as with the other bacterial extracts, the base peak was the sodiated form of menaquinone 8 (b).
Figure 3. (a) Extrapolated ion count for a TLC extract of Serratia marcescens extract. (b) Mass spectrometric analysis of peak B detected at a retention time of 30.6 min.
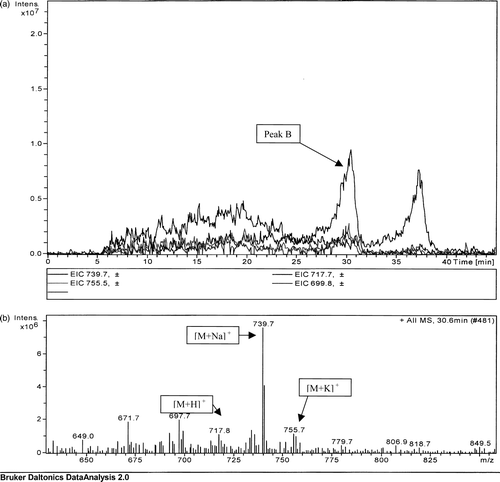
The peak detected for Enterococcus faecium TLC lipid extract (a, peak C), although of weak intensity, gave sufficient signal to allow for identification as menaquinone 7. As with the analysis for the previous strains, the protonated, sodiated and potassiated forms of menaquinone were detected, with the sodiated analogue forming the base peak.
Discussion
The LC-MS results presented in this paper for the three bacterial strains E. agglomerans, S. marcescens and E. faecium show peaks correlating with sodiated, protonated and hydrogenated derivatives of the menaquinones. Molecules of sodium, potassium and hydrogen are abundant in the environment and in this experiment they could be sourced from the glass vials used to store the samples. Ion contaminants have been reported previously to form apparently stable complexes with the menaquinones during electro-spray ionization Citation[10]. The sodium, which forms the base peak analogue, is most likely forming weak covalent bonds with the oxygen on the ring structures of the menaquinones, as are the hydrogen and potassium.
This previously unreported identification of the strains E. agglomerans, S. marcescens and E. faecium as menaquinone producers will in the first instance contribute to aiding in their microbiological classification Citation[11]. In addition, the sourcing of these additional vitamin K-producing strains from the neonatal gut flora shows their potential as bacteria that could contribute in the neonate to the overall requirements of vitamin K, an essential vitamin in the human blood clotting process Citation[6]. In the study presented here, the newly identified vitamin K-producing strains together with the several other previously reported vitamin K-producing strains identified in this study namely, Bacteroides ovatus, Citrobacter freundii, Enterococcus faecalis, Escherichia coli, Prevotella buccae, Staphylococcus capitis, Staphylococcus epidermidis, Staphylococcus haemolyticus and Staphylococcus warneri, were predominantly isolated only in the 6-week-old faecal samples rather than in the 0–1- or 2–5-day-old samples analysed (unpublished results). This study looked at the ability of isolated bacterial strains to produce vitamin K; however, further quantitative studies would be required to confirm whether the bacteria listed above contribute significantly to the overall vitamin K requirement of the newborn infant in the age group studied, which could have implications in relation to the duration of hospital regimes for oral administration of vitamin K Citation[9], Citation[10] as a prophylactic treatment for the prevention of haemorrhagic disease of the newborn Citation[12–14]. The bacterial strains reported as vitamin K producers in this paper are predominantly facultative anaerobes rather than strict anaerobes, which is reflective of the young neonatal gut only developing a more anaerobic environment at the age of the neonates involved in this study. It would be interesting to further develop the study to other age groups in a more quantitative manner over a longer period to determine the contribution of strictly anaerobic bacteria to the vitamin K concentration in the gut.
The authors would like to acknowledge the support from the Irish Department of Education PDRSP Strand 1 funding together with Seed and PhD continuance funding from ITT Dublin. The authors would especially like to acknowledge Dr Winfred Gorman and Sr Nicola Clarke of the National Maternity Hospital, Ireland, who collaborated in the study of the gut flora which led to the isolation of the strains reported in this paper, and finally the mothers and their newborns of the NMH Ireland who provided the source faecal samples that were analysed in this study. Thanks also go to Dr Brett Paul and Leon Bannon of Dublin City University for the use of their mass spectrometer as well as their help and advice. Thanks are also extended to Mary Deasy, Brian Murray and Eithne Dempsey from ITT Dublin for their technical advice.
References
- Fauler G, Muntean W, Leis HJ. Vitamin K. Modern Chromatographic Analysis of Vitamins 2000; 3: 229–70
- Ramotar K, Conly JM, Chubb H, Louie TJ. Production of menaquinones by intestinal anaerobes. J Infect Dis 1984; 150: 213–19
- Conly JM. Assay of menaquinones in bacterial cultures, stool samples and intestinal contents. Methods Enzymol 1997; 282: 457–66
- Collins MD. Distribution of menaquinones within members of the genus Staphylococcus. FEMS Microbiol Lett 1981; 12: 83–5
- Suttie JW. The importance of menaquinones in human nutrition. Annu Rev Nutr 1995; 15: 399–417
- Cooke G, Behan J, Clarke N, Gorman W, Costello M. Comparing the gut flora of Irish breast- and formula-fed neonates aged between birth and 6 weeks old. J Microb Ecol Health Dis (in press).
- Bligh EG, Dyer WJ. A rapid method of total lipid extraction and purification. Can J Biochem Physiol 1959; 37: 912–17
- Hammond RK, White DC. Separation of vitamin K2 isoprenalogues by reversed phase thin layer chromatography. J Chromatogr 1969; 45: 446–52
- Fernandez F, Collins MD. Vitamin K composition of anaerobic gut bacteria. FEMS Microbiol Lett 1987; 41: 175–80
- Chapman JR. Practical organic mass spectrometry. Wiley, New York 1995; 1–38
- Yamamoto K, Murakami R, Takamura Y. Isoprenoid quinone, cellular fatty acid composition and diaminopimelic acid isomers of newly classified thermophillic anaerobic Gram-positive bacteria. FEMS Microbiol Lett 1998; 161: 351–8
- Jonville-Bera AP, Auret E. Study of use of vitamin K in neonates in France. Eur J Pharmacol 1997; 52: 333–7
- Philip RK, Gul R, Dunworth M, Keane. Ireland lacks consensus on neonatal vitamin K prophylaxis. BMJ 2001; 323: 1068
- Von Kries R. Vitamin K prophylaxis – a useful public health measure. Paediatr Perinat Epidemiol 1992; 6: 7–13