Abstract
Objectives: This study explored the association between tissue transglutaminase autoantibody (tTGA), high-risk human leucocyte antigen (HLA) genotypes and islet autoantibodies in children with newly diagnosed type 1 diabetes (T1D).
Patients and methods: Dried blood spots and serum samples were taken at diagnosis from children <18 years of age participating in Better Diabetes Diagnosis (BDD), a Swedish nationwide prospective cohort study of children newly diagnosed with T1D. We analyzed tTGA, high-risk HLA DQ2 and DQ8 (DQX is neither DQ2 nor DQ8) and islet auto-antibodies (GADA, IA-2A, IAA, and three variants of Zinc transporter; ZnT8W, ZnT8R, and ZnT8QA).
Results: Out of 2705 children diagnosed with T1D, 85 (3.1%) had positive tTGA and 63 (2.3%) had borderline values. The prevalence of tTGA was higher in children with the HLA genotypes DQ2/2, DQ2/X or DQ2/8 compared to those with DQ8/8 or DQ8/X (p = .00001) and those with DQX/X (p ≤ .00001). No significant differences were found in relation to islet autoantibodies or age at diagnosis, but the presence of tTGA was more common in girls than in boys (p = .018).
Conclusion: tTGA at T1D diagnosis (both positive and borderline values 5.4%) was higher in girls and in children homozygous for DQ2/2, followed by children heterozygous for DQ2. Only children with DQ2 and/or DQ8 had tTGA. HLA typing at the diagnosis of T1D can help to identify those without risk for CD.
Introduction
Celiac Disease (CD) and type 1 diabetes mellitus (T1D) are autoimmune multifactorial disorders that co-occur and both are associated with the human leucocyte antigen (HLA) class II genes on chromosome 6.21 [Citation1,Citation2]. The prevalence of CD in children with T1D varies between 3-16% in different cohorts [Citation1, reviewed in Citation3,Citation4], and previous studies in Sweden have shown the prevalence to be around 10% [Citation5–7].
CD is a small intestinal immune-mediated enteropathy in genetically predisposed individuals, which is precipitated by dietary gluten intake [Citation8]. The average prevalence of clinically detected CD in the general population is 0.4–1.0% in the western world [Citation9,Citation10] and it is one of the most common childhood diseases in Sweden [Citation11]. The prevalence has been reported to be as high as 2.9% in 12-year-old children from the general population who were born during a so-called “epidemic” of CD [Citation12] and has been described to be as high as 3.5% in children with the high-risk HLA genotypes DQ2 and/or DQ8 [Citation13].
The presence of the HLA risk genotypes DQ2 or DQ8 is necessary, but not sufficient, to develop CD [Citation2,Citation13–16]. Gluten exposure is essential to develop CD, but other environmental factors also seem to contribute to CD as it is increasing in the western world [Citation2–7,Citation9,Citation11,Citation17–20]. Tissue transglutaminase antibodies (tTGA) are autoimmune markers of CD, with a high positive predictive value [Citation21–24].
T1D is caused by an autoimmune process leading to insulin deficiency [Citation25] and the incidence in Swedish children and adolescents is the second highest in the world, after Finland. As described in other countries, the incidence of childhood T1D has doubled in Sweden in the past 25 years and today the incidence is >40 per 100,000 children aged 0–15 years [Citation26].
The autoantibodies present at T1D diagnosis are 65 kDa isoform of glutamic acid decarboxylase autoantibodies (GADA, insulinoma-associated protein 2 autoantibodies (IA-2A), insulin autoantibodies (IAA), and autoantibodies against zinc-transporter 8 (ZnT8A). Earlier studies have shown that 93 to 95% of Swedish children with newly diagnosed T1D have at least one of these autoantibodies [Citation27,Citation28], and a recent European study showed that 92% of T1D children have HLA DQ2 and/or DQ8 [Citation27], compared to 30–50% in the general population [Citation23].
The aim of this study was to investigate associations between tTGA and islet autoantibodies as well as to confirm earlier findings of the relation between celiac autoimmunity and HLA, at the diagnosis of diabetes in a large population-based cohort in Sweden. We also explored associations with gender and age.
Methods
Study design and subjects
Our study was based on data from Better Diabetes Diagnosis (BDD), an ongoing nationwide prospective population-based cohort study for children newly diagnosed with diabetes [Citation27–29]. This sub-study included 2705 children and adolescents (56% boys) with T1D, from birth to 18 years old at the time of their diabetes diagnosis. They were recruited between May 2005 and November 2009 from 40 (95%) out of the 42 pediatric diabetes clinics where all children with diabetes are diagnosed and treated in Sweden (). The two not participating clinics are small and have less than 4% of all children with diabetes.
Figure 1. Flowchart of the better diabetes diagnosis (BDD) study and immunoglobulin a tissue transglutaminase antibodies (tTGA).
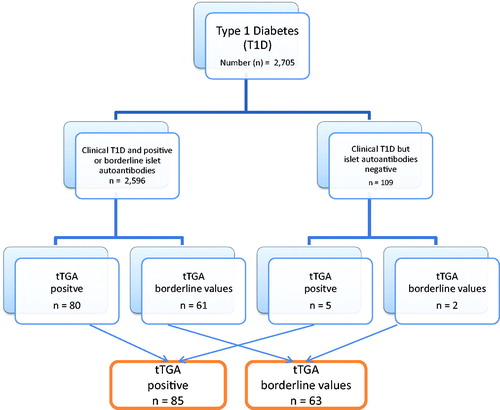
The American Diabetes Association recommendations and classification of T1D were used to determine the clinical diagnosis [Citation30] and this was re-evaluated after one year [Citation31], and all children in the study group continued to meet the criteria. Within three days of the diabetes diagnosis we obtained dried blood spots (DBS) for HLA-DQ typing, and serum samples for GADA, IA-2A, IAA, and all three ZnT8A to either one, two, or all three amino acid variants at position 325, ZnT8 arginine, ZnT8 tryptophan, ZnT8 glutamine, and for tTGA. Serum samples for the islet autoantibody assays were stored at −20 °C until analyzed. All patients and their guardians provided informed consent. The Regional Ethics Review Board in Stockholm, Sweden, approved the study.
tTGA analysis
The analysis of tTGA (kit No. L2KTD6) was used on serum samples on the Immulite 2000 analyzer according to the manufacturer’s instructions (Siemens Healthcare Diagnostics, Deerfield, IL, USA). Values >50 IU were considered positive and values between 10 and 50 were considered borderline values. The values were evenly distributed and that there were no clusters of values (data not shown).
HLA genotyping
HLA-DQB1 and DQA1 genotypes were analyzed by sequence-specific oligonucleotide probes on DBS used directly for polymerase chain reaction (PCR) amplification of DQA1 and DQB1 alleles as previously described [Citation32] using a DELFIA hybridization assay (PerkinElmer Inc., Waltham, Massachusetts, USA). The first set of probes defined HLA-DQB1*02:01, 03:02, 03:01, 06:02, 06:03, and 06:04 and the second set of probes defined the HLA-DQA1 alleles DQA1*02:01, 03:01, and 05:01. In order to simplify the nomenclature, the HLA genotype DQA1*03:01-B1*03:02/DQA1*05:01–B1*02:01 will be abbreviated to DQ2/8, DQA1*05:01–B1*02:01/DQA1*05:01–B1*02:01will be abbreviated to homozygous DQ2/2; DQA1*05:01–B1*02:01/X (X is neither DQ8 nor DQ2) will be abbreviated to DQ2/X; DQA1*03:01–B1*03:02/DQA1*03:01–B1*03:02 homozygous DQ8/8 and DQA1*03:01–B1*03:02/X will be abbreviated to DQ8/X (X is not DQ2). It should be noted that children with the DQA1*02:01–B1*02:01 haplotypes are described as DQ2.2/X. For the purpose of comparison, the HLA genotyping were classified into four groups of genotypes: (i) DQ2/2, DQ2/X, and DQ2.2/X; (ii) DQ2/8; (iii) DQ8/8 and DQ8/X and (iv) children with neither DQ2 nor DQ8, labeled as DQX/X, where DQX was any haplotype other than DQ2, DQ2.2, and DQ8. HLA genotyping was unclassified in 34 of the 2705 children (missing values 1.3%).
Autoantibodies to GAD65, IA-2, and insulin
Recombinant GAD65 and IA-2 were labelled with 35S-methionine (GE Healthcare Life Sciences, Amersham, UK) by in vitro coupled transcription and translation in the TNT SP6 coupled reticulocyte lysate system (Promega, Southampton, UK) as previously described [Citation33]. IAA was determined in a noncompetitive radioligand-binding assay using 125I-insulin, as previously described [Citation34]. Details of the procedures, the intra-assay coefficients of the variations and the validation of the laboratory have previously been described [Citation27]. Samples were considered positive if GADA was >50 U/mL, IA-2A was >10 U/mL, and IAA was >1 RU. Values for GADA of 35–50 U/mL, IA-2A of 6–10 U/mL, and IAA between 0.81–1.0 RU were considered borderline.
Autoantibodies to zinc transporter variants
The radioligand-binding assay for all three variants – ZnT8R, ZnT8W, and ZnT8Q – were performed separately, as previously described [Citation35], and the intra-assay coefficients of the variations and the results of the laboratory validation have also been previously described [Citation27]. The cutoff values for ZnT8RA were ≥75 U/mL, for ZnT8WA they were ≥75 U/mL and for ZnT8QA they were ≥100 U/mL to positive. Values between 60–74 U/mL for ZnT8RA, 60–74 U/mL for ZnT8WA and between 70–99 U/mL for ZnT8QA were considered borderline.
Statistical methods and data analysis
All tests based on proportions were carried out using the test of homogeneity based on the chi-square distribution or, in the case of small-expected frequencies, Fisher’s exact test and p values <.05 were considered significant. In addition, descriptive statistics have been used to characterize the data. The quantitative variables have been expressed as ranges, medians, means, and standard deviations of the mean and the categorical variables have been described as frequencies and/or percentages. All analyses were carried out on the SAS system for Windows, version 9.1 (SAS Institute Inc, Cary, NC, USA).
Results
Characteristics of T1D children
The study population comprised 2705 children with T1D (56% male). The majority of these (n = 2596, 96%) had one or more of the tested islet autoantibodies (GADA, IA-2A, IAA, ZnT8A), as 2568 were autoantibody positive and 28 had borderline values (, ). The median age of the children at T1D diagnosis was 10.1 years (range 0.1–17.9 years), 10.9 years for boys and 9.9 years for girls.
Table 1. Description of 2705 children newly diagnosed with type 1 diabetes mellitus (T1D) in the study group, the human leukocyte antigen (HLA) genotype distribution and tissue transglutaminase antibodies (tTGA) results.
tTGA was common at the time of clinical diagnosis
Of the 2705 children clinically diagnosed with T1D, 85 (3.1%) tested positive for tTGA and 63 (2.3%) had borderline values, together 5.4%. Of the 109 children with clinically diagnosed T1D, but no islet autoantibodies, five children (4.5%) were tTGA positive and two children (1.8%) had borderline levels (, ).
HLA-genotype DQ2 correlated to tTGA
The distribution of HLA genotypes according to the values of tTGA is shown in . Positive and borderline values of tTGA were most common in children with the HLA genotype DQ2/2 (10.5%), followed by DQ2/8 (8.3%) and DQ2/X (7.0%). The frequency of tTGA was higher in children with DQ2/2, DQ2/X, DQ2.2/X (together 7.8%) or DQ2/8 (8.0%) compared to both DQ8/8 and DQ8/X (3.7%) (p = .00001) and compared to DQX/X (0%) (p≤.00001). Of the children with the HLA genotype DQ2.2/X, only one child had borderline tTGA. No children with HLA DQX/X had tTGA present at the time of their diabetes diagnosis. The HLA genotype was missing in one of the 85 tTGA positive children and two of the 63 children with borderline values.
Table 2. The distribution of human leukocyte antigen (HLA) genotypes in 2,671 newly diagnosed type 1 diabetes children by Immunoglobulin A tissue transglutaminase antibodies (tTGA).
tTGA and islet autoantibodies
The presence of tTGA did not vary in relation to the different autoantibodies, including the three subclasses of ZnT8W, ZnT8R or ZnT8QA nor in the patients lacking autoantibodies. The distribution of islet autoantibodies in relation to tTGA is shown in and ZnT8A subclasses data are shown together.
Table 3. The distribution of islet autoantibodies among type 1 diabetes children by Immunoglobuline A tissue transglutaminase antibodies (tTGA).
Gender differences in tTGA prevalence
The presence of both positive and borderline values of tTGA was more common in girls (4.2% and 6.6%, respectively, p = .005) than in boys (2.3% and 4.6%, respectively, p = .018) Eighty-four girls had positive or borderline values (57% out of the total with tTGA), in compare to 64 boys ().
Age at diagnosis and tTGA prevalence
The median age of the children with tTGA positive or borderline values at T1D diagnosis was 11.1 years and the mean age was 10.6 years for the total study population (p = n.s.). The presence of tTGA tended to be lower in the youngest age group (4.3%) than in the group of five to nine-year-old children (5.2%) and had a tendency to be highest at 10–14 years of age (6.2%) and 15-18 years of age (5.6%) although not significant (p = n.s.). In the youngest age group, the total number of children in a specially controlled subgroup less than two years of age were 76, corresponding to 2.8% of the study population. None of them had a positive tTGA, and two children had borderline values (2.6% of these youngest children).
Discussion
In this nationwide cohort study of 2,705 children at the diagnosis of T1D, we found that 3.1% had tTGA and another 2.3% had borderline values of tTGA and all these children had DQ2 and/or DQ8. The presence of tTGA was most common in children with DQ2/2, followed by the DQ2/8 and DQ2/X HLA genotypes and was more common in girls. No significant differences were found between the groups of children based on islet autoantibodies or age at diagnosis.
The prevalence of tTGA at the diagnosis of diabetes confirmed previous studies from smaller diabetes populations in Sweden. One study found tTGA among 5.5% (9/162) of children at the diagnosis of diabetes [Citation36] and another showed that 3% (10/300) children had newly detected CD, confirmed by a biopsy, within one year of their T1D diagnosis [Citation6]. We are aware that we studied tTGA autoantibodies as a potential risk factor for CD when the child was diagnosed with diabetes, and that CD had not been confirmed by a biopsy at that point. On one hand, tTGA has been shown to be a good predictor of CD, with a positive predictive value of 90–97% in the general population [Citation21] and it is the recommended test for screening for CD [Citation22–24]. On the other hand, tTGA levels at T1D diagnosis may change over time, as one retrospective study has shown that 17 (35%) of the study population of 815 with low positive tTGA at T1D diagnosis were normalized when re-checked without any change on their diet [Citation37], but these children did not undergo a biopsy to verify the presence or absence of CD.
The distribution of HLA DQ2 and DQ8 in the T1D study population was comparable with a smaller study in another European population, where 8% had neither DQ2 or DQ8 [Citation38]. We found that tTGA was significantly more common in children homozygous for DQ2 (10.5%) tight followed by DQ2/8 (8.3%). Homozygous DQ2 alleles and DQ2/X are the most common risk alleles for developing CD in otherwise healthy children, where 90% of the children with CD have at least DQ2 and 10% lack DQ2 but almost all are positive for DQ8 [Citation39,Citation40]. In this study about CD autoimmunity at the onset of T1D, tTGA was as well common in T1D children with the DQ8/X HLA-genotype (3.7%), but the presence of DQ2 also seems to increase the risk of developing CD autoantibodies in children with T1D, as it has been supported by other studies [Citation41,Citation42]. Furthermore, around 30% of the study population with CD autoimmunity lacked DQ2 which is in line with the study investigating children with both diseases in Sweden and Denmark [Citation7]. However, an Australian study investigating the use of HLA-typing finding CD, though in a mixed population of children and adults with T1D, found the same amount of subjects with both diseases lacking DQ2 as in subjects with CD only [Citation43].
DQ2.2 is a variant of one half of the DQ2 heterodimer and only one child out of 47 with DQ2.2/X had a borderline value of tTGA, and none had a positive value, although it has been shown to be associated with a higher risk for CD in otherwise healthy individuals [Citation44]. The results indicate that the DQ2.2/X genotype is not associated with an increased risk for tTGA at the time of clinical diagnosis of T1D. None of the children who lacked DQ2 (or the variant mentioned above) and DQ8 had positive or borderline values of tTGA.
It seems that the genetic high-risk components must be accompanied by environmental determinants to enhance and trigger autoimmunity and disease onset. These could include when gluten is introduced into a child’s diet, the amount of gluten they consume in childhood, the lack of breastfeeding during a crucial period, some virus exposure and other factors not yet well-known or understood [Citation3,Citation7,Citation14,Citation18,Citation45–48].
In this study, the presence of different islet autoantibodies was not associated with the frequency of tTGA, neither the more routinely analyzed autoantibodies (GADA, IA-2A, IAA) nor the ZnT8A subclasses. It may be interpreted that these diseases develop independently of each other and that their coexistence is due to the shared genetic HLA risk, which is a strong predisposing factor in both diseases. This interpretation is also supported by the TEDDY study, investigating children with increased genetic risk for type 1 diabetes, which showed limited overlapping in children with tTGA and islet autoantibodies [Citation49]. The subgroup with no islet autoantibodies showed a slightly higher prevalence of tTGA (6.4%), but this subgroup group was small and it is not possible to draw conclusions.
As expected, we observed more T1D in boys [Citation26,Citation27,Citation29] while we found a higher prevalence of both positive and borderline tTGA in girls. It is known that females have a higher life risk of developing CD, diagnosed by clinical detection [Citation50,Citation51] and screening [Citation12] in the general population. This also seems to be the case in children who develop tTGA in addition to diabetes. There were no considerable gender differences in tTGA by HLA genotype. Taken together, these results may suggest that girls are more susceptible to environmental exposures that influence the immunological chain leading to the development of CD [Citation14,Citation50].
Standardized subgroups by age were created for comparison and the largest of them was the 1053 children aged 10–14 years (39%). This group tended to have a higher frequency of tTGA (6.2%), even if we could not see a significant difference. These results agree with previous studies, showing a mean age at CD diagnosis in screened T1D children of 8.3 in one study [Citation5] and 11.1 years in another [Citation6]. Although it has been shown that low age at T1D onset is a risk for developing CD, these results are conflicting as presented in a review [Citation4].
One limitation of the study design was the lack of clinical data on known CD before the diabetes diagnosis. Because of this, some children could have had CD and be on a gluten-free diet and therefore had normal tTGA antibodies, or some could have been diagnosed with CD with non-perfect compliance to the diet, or just a short time on the diet, and then have had borderline or positive tTGA at the time of diabetes diagnosis. We assume that some individuals fall into this category, as other screening carried out by Swedish studies have revealed that clinically diagnosed CD before T1D was between 0.7–1.8% in the T1D population [Citation5,Citation6,Citation36].
One methodological bias may be that tTGA has been reported to have a lower sensitivity in children who are less than 2 years of age [Citation36,Citation52] and the youngest children in our study had been exposed to gluten for a short time. Another possible bias could be that tTGA is a method based on immunoglobulin A (IgA) and we did not control for IgA deficiency, which is known to be more common in children who develop CD than in the general population. It was shown in another Swedish T1D cohort [Citation5] that 11/836 (1.3%) children had total IgA deficiency; defined as IgA <0.07 g/L, but none of the 300 T1D children studied in the South of Sweden [Citation6] was reported to have IgA deficiency. However, as mentioned above, possible methodological biases could have only led to underestimating the prevalence of tTGA and thereby the risk for CD in our study population.
The strength of the present study was the cohort design: a nationwide prospective population-based pediatric cohort. We evaluated the association between tTGA as a CD marker and the HLA genotype, which could have implications for clinical practice, as it may not be necessary to continue the annual screening of children without DQ2 and DQ8, which was 8% in this cohort.
In conclusion, we found a high prevalence (5.4%) of tTGA at the time of the T1D diagnosis in this nationwide population-based cohort of children from birth to 18 years of age. The presence of tTGA was more common in girls and most common in children with the homozygous HLA genotype DQ2/2, follow by DQ2 in combination with the DQ8 and DQ2/X genotypes. None of the children who lacked DQ2 and DQ8 had tTGA and probably do not need further screening.
Supplemental Material
Download MS Word (12.5 KB)Acknowledgments
The authors are grateful to the children and parents who participated in the study and thank the BBD Group participants - listed in the supplementary appendix - for their excellent participation over the years. The authors also acknowledge Per Näsman, Ph.D., Senior Statistician at the Royal Institute of Technology, for his help with the statistical analysis. We would also like to pay tribute to our dear co-author Assistant Professor Lena Grahnquist, who has passed away, and dedicate this paper to her memory.
Disclosure statement
No potential conflict of interest was reported by the authors.
Additional information
Funding
References
- Sud S, Marcon M, Assor E, et al. Celiac disease and pediatric type 1 diabetes: diagnostic and treatment dilemmas. Int J Pediatr Endocrinol. 2010;2010:161285
- Redondo MJ, Steck AK, Pugliese A. Genetics of type 1 diabetes. Pediatr Diabetes. 2018;19:346–353.
- Pham-Short A, Donaghue KC, Ambler G, et al. Screening for celiac disease in type 1 diabetes: a systematic review. Pediatrics. 2015;136:e170–e176.
- Elfstrom P, Sundstrom J, Ludvigsson JF. Systematic review with meta-analysis: associations between coeliac disease and type 1 diabetes. Aliment Pharmacol Ther. 2014;40:1123–1132.
- Bybrant MC, Ortqvist E, Lantz S, et al. High prevalence of celiac disease in Swedish children and adolescents with type 1 diabetes and the relation to the Swedish epidemic of celiac disease: a cohort study. Scand J Gastroenterol. 2014;49:52–58.
- Larsson K, Carlsson A, Cederwall E, et al. Annual screening detects celiac disease in children with type 1 diabetes. Pediatr Diabetes. 2008;9:354–359.
- Adlercreutz EH, Svensson J, Hansen D, et al. Prevalence of celiac disease autoimmunity in children with type 1 diabetes: regional variations across the Oresund strait between Denmark and southernmost Sweden. Pediatr Diabetes. 2015;16:504–509.
- Dicke WK. Treatment of celiac disease. Ned Tijdschr Geneeskd. 1951;95:124–130.
- Lohi S, Mustalahti K, Kaukinen K, et al. Increasing prevalence of coeliac disease over time. Aliment Pharmacol Ther. 2007;26:1217–1225.
- Ludvigsson JF, Rubio-Tapia A, van Dyke CT, et al. Increasing incidence of celiac disease in a North American population. Am J Gastroenterol. 2013;108:818–824.
- Carlsson A, Agardh D, Borulf S, et al. Prevalence of celiac disease: before and after a national change in feeding recommendations. Scand J Gastroenterol. 2006;41:553–558.
- Myleus A, Ivarsson A, Webb C, et al. Celiac disease revealed in 3% of Swedish 12-year-olds born during an epidemic. J Pediatr Gastroenterol Nutr. 2009;49:170–176.
- Bjorck S, Brundin C, Lorinc E, et al. Screening detects a high proportion of celiac disease in young HLA-genotyped children. J Pediatr Gastroenterol Nutr. 2010;50:49–53.
- Liu E, Lee HS, Aronsson CA, et al. Risk of pediatric celiac disease according to HLA haplotype and country. N Engl J Med. 2014;371:42–49.
- Smigoc S, Mendez DA, Kunilo Jamnik S, et al. High-risk genotypes HLA-DR3-DQ2/DR3-DQ2 and DR3-DQ2/DR4-DQ8 in cooccurrence of type 1 diabetes and celiac disease. Autoimmunity. 2016;49:240–247.
- Gutierrez-Achury J, Romanos J, Bakker SF, et al. Contrasting the genetic background of type 1 diabetes and celiac disease autoimmunity. Diabetes Care. 2015;38:37–44.
- Adlercreutz EH, Weile C, Larsen J, et al. A gluten-free diet lowers NKG2D and ligand expression in BALB/c and non-obese diabetic (NOD) mice. Clin Exp Immunol. 2014;177:391–403.
- Aronsson CA, Lee HS, Liu E, et al. Age at gluten introduction and risk of celiac disease. Pediatrics. 2015;135:239–245.
- Ivarsson A, Myleus A, Norstrom F, et al. Prevalence of childhood celiac disease and changes in infant feeding. Pediatrics. 2013;131:e687–e694.
- Myleus A, Hernell O, Gothefors L, et al. Early infections are associated with increased risk for celiac disease: an incident case-referent study. BMC Pediatr. 2012;12:194.
- Toftedal P, Nielsen C, Madsen JT, et al. Positive predictive value of serological diagnostic measures in celiac disease. Clin Chem Lab Med. 2010;48:685–691.
- Leffler DA, Schuppan D. Update on serologic testing in celiac disease. Am J Gastroenterol. 2010;105:2520–2524.
- Sandstrom O, Rosen A, Lagerqvist C, et al. Transglutaminase IgA antibodies in a celiac disease mass screening and the role of HLA-DQ genotyping and endomysial antibodies in sequential testing. J Pediatr Gastroenterol Nutr. 2013;57:472–476.
- Sandstrom O, Rosen A, Ivarsson A. The Role of HLA-DQ Genotyping in Celiac Disease. J Pediatr Gastroenterol Nutr. 2016;62(3):e30–1.
- Roep BO, Peakman M. Antigen targets of type 1 diabetes autoimmunity. Cold Spring Harb Perspect Med. 2012;2:a007781.
- Berhan Y, Waernbaum I, Lind T, et al. Thirty years of prospective nationwide incidence of childhood type 1 diabetes: the accelerating increase by time tends to level off in Sweden. Diabetes. 2011;60:577–581.
- Andersson C, Vaziri-Sani F, Delli A, et al. Triple specificity of ZnT8 autoantibodies in relation to HLA and other islet autoantibodies in childhood and adolescent type 1 diabetes. Pediatr Diabetes. 2013;14:97–105.
- Andersson C, Larsson K, Vaziri-Sani F, et al. The three ZNT8 autoantibody variants together improve the diagnostic sensitivity of childhood and adolescent type 1 diabetes. Autoimmunity. 2011;44:394–405.
- Delli AJ, Lindblad B, Carlsson A, et al. Type 1 diabetes patients born to immigrants to Sweden increase their native diabetes risk and differ from Swedish patients in HLA types and islet autoantibodies. Pediatr Diabetes. 2010;11:513–520.
- Diagnosis and classification of diabetes mellitus. Diabetes Care. 2007;30: S42–S47.
- Hanberger L, Samuelsson U, Lindblad B, et al. A1C in children and adolescents with diabetes in relation to certain clinical parameters: the Swedish Childhood Diabetes Registry SWEDIABKIDS. Diabetes Care. 2008;31:927–929.
- Kiviniemi M, Hermann R, Nurmi J, et al. A high-throughput population screening system for the estimation of genetic risk for type 1 diabetes: an application for the TEDDY (the Environmental Determinants of Diabetes in the Young) study. Diabetes Technol Ther. 2007;9:460–472.
- Grubin CE, Daniels T, Toivola B, et al. A novel radioligand binding assay to determine diagnostic accuracy of isoform-specific glutamic acid decarboxylase antibodies in childhood IDDM. Diabetologia. 1994;37:344–350.
- Williams AJ, Bingley PJ, Bonifacio E, et al. A novel micro-assay for insulin autoantibodies. J Autoimmun. 1997;10:473–478.
- Vaziri-Sani F, Delli AJ, Elding-Larsson H, et al. A novel triple mix radiobinding assay for the three ZnT8 (ZnT8-RWQ) autoantibody variants in children with newly diagnosed diabetes. J Immunol Methods. 2011;371:25–37.
- Agardh D, Nilsson A, Tuomi T, et al. Prediction of silent celiac disease at diagnosis of childhood type 1 diabetes by tissue transglutaminase autoantibodies and HLA. Pediatr Diabetes. 2001;2:58–65.
- Waisbourd-Zinman O, Hojsak I, Rosenbach Y, et al. Spontaneous normalization of anti-tissue transglutaminase antibody levels is common in children with type 1 diabetes mellitus. Dig Dis Sci. 2012;57:1314–1320.
- Binder E, Loinger M, Mühlbacher A, et al. Genotyping of coeliac-specific human leucocyte antigen in children with type 1 diabetes: does this screening method make sense? Arch Dis Child. 2017;102:603–606.
- Ludvigsson JF, Green PH. Clinical management of coeliac disease. J Intern Med. 2011;269:560–571.
- Sollid LM, Markussen G, Ek J, et al. Evidence for a primary association of celiac disease to a particular HLA-DQ alpha/beta heterodimer. J Exp Med. 1989;169:345–350.
- Bao F, Yu L, Babu S, et al. One third of HLA DQ2 homozygous patients with type 1 diabetes express celiac disease-associated transglutaminase autoantibodies. J Autoimmun. 1999;13:143–148.
- Viken MK, Flåm ST, Skrivarhaug T, et al. HLA class II alleles in Norwegian patients with coexisting type 1 diabetes and celiac disease. HLA. 2017;89:278–284.
- Doolan A, Donaghue K, Fairchild J, et al. Use of HLA typing in diagnosing celiac disease in patients with type 1 diabetes. Diabetes Care. 2005;28:806–809.
- Karell K, Louka AS, Moodie SJ, et al. HLA types in celiac disease patients not carrying the DQA1*05-DQB1*02 (DQ2) heterodimer: results from the European genetics cluster on celiac disease. Hum Immunol. 2003;64:469–477.
- Svensson M, Ramelius A, Nilsson AL, et al. Antibodies to influenza virus A/H1N1 hemagglutinin in Type 1 diabetes children diagnosed before, during and after the SWEDISH A(H1N1)pdm09 vaccination campaign 2009–2010. Scand J Immunol. 2014;79:137–148.
- Wahlberg J, Fredriksson J, Vaarala O, et al. Vaccinations may induce diabetes-related autoantibodies in one-year-old children. Ann N Y Acad Sci. 2003;1005:404–408.
- Adlercreutz EH, Wingren CJ, Vincente RP, et al. Perinatal risk factors increase the risk of being affected by both type 1 diabetes and coeliac disease. Acta Paediatr. 2015;104:178–184.
- Andren Aronsson C, Lee HS, Koletzko S, et al. Effects of gluten intake on risk of celiac disease: a case-control study on a Swedish birth cohort. Clin Gastroenterol Hepatol. 2016;14:403–409 e3.
- Hagopian W, Lee H-S, Liu E, et al. Co-occurrence of Type 1 Diabetes and celiac disease autoimmunity. Pediatrics Nov. 2017;140:e20171305.
- Ivarsson A, Persson LA, Nystrom L, et al. The Swedish coeliac disease epidemic with a prevailing twofold higher risk in girls compared to boys may reflect gender specific risk factors. Eur J Epidemiol. 2003;18:677–684.
- Megiorni F, Mora B, Bonamico M, et al. HLA-DQ and susceptibility to celiac disease: evidence for gender differences and parent-of-origin effects. Am J Gastroenterol. 2008;103:997–1003.
- Husby S, Koletzko S, Korponay-Szabo IR, et al. European society for pediatric gastroenterology, hepatology, and nutrition guidelines for the diagnosis of coeliac disease. J Pediatr Gastroenterol Nutr. 2012;54:136–160.