Abstract
Study objectives: Narcolepsy type 1 (NT1) is a chronic sleep disorder characterized by loss of hypocretin-producing neurons. Increased NT1 incidence was observed in Sweden following mass-vaccination with Pandemrix®. Genetic association to HLA DQB1*06:02 implies an autoimmune origin, but target autoantigen remains unknown. Candidate autoantigens for NT1 have previously been identified in solid-phase immunoassays, while autoantibodies against conformation-dependent epitopes are better detected in radiobinding assays. The aims are to determine autoantibody levels against nine candidate autoantigens representing (1) proteins of the hypocretin transmitter system; Preprohypocretin (ppHypocretin), Hypocretin peptides 1 and 2 (HCRT1 and HCRT2) and Hypocretin receptor 2 (HCRTR2); (2) proteins previously associated with NT1; Tribbles homologue 2 (TRIB2), Pro-opiomelanocortin/alpha-melanocyte-stimulating-hormone (POMC/α-MSH) and Prostaglandin D2 Receptor DP1 (DP1); (3) proteins suggested as autoantigens for multiple sclerosis (another HLA DQB1*06:02-associated neurological disease); ATP-dependent Inwardly Rectifying Potassium Channel Kir4.1 (KIR4.1) and Calcium-activated chloride channel Anoctamin 2 (ANO2).
Methods: Serum from post-Pandemrix® NT1 patients (n = 31) and their healthy first-degree relatives (n = 66) were tested for autoantibody levels in radiobinding assays separating autoantibody bound from free labelled antigen with Protein A-Sepharose. 125I-labelled HCRT1 and HCRT2 were commercially available while 35S-methionine-labelled ppHypocretin, HCRTR2, TRIB2, α-MSH/POMC, DP1, KIR4.1 or ANO2 was prepared by in vitro transcription translation of respective cDNA. In-house standards were used to express data in arbitrary Units/ml (U/ml).
Results: All radiolabelled autoantigens were detected in a concentration-dependent manner by respective standard sera. Levels of autoantibodies in the NT1 patients did not differ from healthy first-degree relatives in any of the nine candidate autoantigens.
Conclusions: None of the nine labelled proteins proposed to be autoantigens were detected in the radiobinding assays for conformation-dependent autoantibodies. The results emphasise the need of further studies to identify autoantigen(s) and clarify the mechanisms in Pandemrix®-induced NT1.
1. Introduction
Following the influenza pandemic 2009 vaccination with Pandemrix® (A/H1N1pdm09) an increased incidence of narcolepsy type 1 (NT1) was observed among children and adolescents in Sweden and Finland [Citation1–3]. The pathophysiology of hypocretin cell-destruction leading to NT1 remains unknown. An autoimmune aetiology has been proposed due to the strong association between NT1 and HLA-DQB1*06:02 allele [Citation4,Citation5]. Despite the strong genetic association, attempts to demonstrate humoural NT1 autoimmunity have up to date been unsuccessful [Citation6].
NT1 is associated with the loss of the ∼70,000 hypocretin producing neurons located in the lateral hypothalamus [Citation7]. These cells are exclusively responsible for the production of prepro-hypocretin (ppHypocretin). The neuropeptides hypocretins 1 and 2 (HCRT1 and HCRT2 also known as orexins A and B) are generated from ppHypocretin. Released HCRT1 and HCRT2 act as excitatory neurotransmitters, mediating their action through G-protein coupled receptors, hypocretin receptors 1 and 2 (HCRTR1 and HCRTR2) [Citation8]. The prepro-hypocretin and the hypocretin neuropeptides have been hypothesised as target autoantigens because of the autoimmune reaction directed towards hypocretin neurons. T-cell reactivity [Citation9,Citation10] but no autoantibodies have been demonstrated to hypocretin [Citation11–13]. NT1-specific autoantibodies towards the Hypocretin receptor 2 (HCRT2R) have been investigated with conflicting results [Citation14–16].
Other suggested NT1 autoantigens include Tribbles homologue 2 (TRIB2) [Citation17–20], α-melanocyte stimulating hormone (α-MSH) [Citation21], Prostaglandin D2 Receptor DP1 (DP1) [Citation22], methyltransferase-like 22 (METTL22) and 5′-nucleotidase cytosolic 1A (NT5C1A) [Citation23]. The suggestions of non-hypocretin neuron-specific autoantigens are of interest when considering alternative pathogenic mechanisms behind NT1 as opposed to a direct immune-attack against these cells. Both α-MSH and DP1 have known implications to NT1-associated side-effects. α-MSH is presumed to be involved in weight homeostasis by inhibiting feeding behaviour [Citation24]. NT1 patients are known to have a higher risk to develop obesity and other metabolic problems associated with the loss of hypocretin neurons [Citation25]. Among its many functions in the central nervous system, DP1 is known to be involved in the regulation of sleep through its activation by prostaglandin D2, a sleep-promoting substance [Citation22].
Some studies have suggested, in opposite to the outlined hypocretin-neuron-specific degeneration for NT1, a broader immune reactivity against other areas of the brain [Citation26,Citation27]. Here, we test the autoantibody reactivity for NT1 patients against multiple sclerosis, as another HLA-DQB1*06:02-related neurological disorder, associated autoantigens ATP-dependent Inwardly Rectifying Potassium Channel (KIR4.1) [Citation28–36] and calcium-activated chloride channel Anoctamin 2 (ANO2) [Citation37].
Most previous studies have used assays that require the autoantigen candidate to be displayed in solid phase. Such tests have failed in other cell-specific autoimmune diseases such as autoimmune type 1 diabetes, Addison’s disease, thyroiditis demonstrating that the autoantibodies in these disorders are conformation dependent (for reviews see [Citation38–43]). In these disorders, disease predictive autoantibodies require the analysis to be carried out in immunoassays preserving protein conformation. The aim of this study is, therefore, to determine the presence and levels of autoantibodies towards putative NT1 candidate autoantigens. We used cDNA of ppHypocretin, HCRTR2, TRIB2, α-MSH/POMC, DP1, KIR4.1 and ANO2 to label these proteins with 35S-methionin as well as commercially obtained 125I-labelled HCRT1 or HCRT2 (as these peptides were of inadequate size (too small) to be expressed in our in vitro transcription translation system). Autoantibody levels were determined using radiobinding assays with serum from NT1 patients and their first-degree relatives (FDR) as controls.
2. Materials and methods
2.1. Study population
Serum samples from 31 NT1 patients and 66 FDR were obtained in 2015–2017. Research subjects were Swedish residents diagnosed with NT1 following vaccination with Pandemrix® during 2009–2010. Vaccine-coverage with Pandemrix® was 100% among the NT1 patients and 81.8% for FDR (). Serum samples from a total of 200 healthy blood donors (median age 43, range 19–65 years of age) were used as controls to determine the upper levels of normal in the autoantibody analyses. The blood donor samples were obtained in 2008, which was prior to the Pandemrix®-vaccination campaign in 2009 [Citation44].
Table 1. Study populations.
The AMINA study is a Swedish research project with a focussed aim to investigate the autoimmune aetiology of Pandemrix®-induced NT1 and multiple sclerosis.
2.2. Ethical consideration
The study was approved by the Regional Ethical Review Board in Lund (dnr 2015/257) and all participants gave informed consent. The parents or guardians of under aged study participants gave informed consent.
2.3. Coupled in vitro transcription translation
The cDNA encoding for candidate autoantigens were obtained (sequences are specified in Supplementary Table 1) and subcloned into the pTNT vector as previously described in detail [Citation20]. DNA sequencing was verified by GATC (GATC Biotech AG, Konstanz, Germany) and Thermo Fisher Scientific (Carlsbad, CA). Radio-labelled proteins were expressed through coupled in vitro transcription translation by mixing cDNA with the TnT® SP6 Coupled Reticulocyte Lysate System, as described by the manufacturer (Promega, Madison, WI), and 35S-methionine (PerkinElmer Life and Analytical Sciences, Brussels, Belgium). Unincorporated 35S-methionine was removed following incubation (90 min at 30 °C) using a Nap5 column (GE Healthcare Bio-Sciences, Uppsala, Sweden). The mean incorporation rate of radio-labelled proteins were ppHypocretin 19%, HCRTR2 16%, TRIB2 10%, α-MSH/POMC 17%, DP1 47%, KIR4.1 25% and ANO2 39%.
In-house in vitro transcription translation and 35S-labelling of α-MSH was considered problematic, as the peptide size (too small, 14aa) and amino acid sequence (only one methionine residue) was inadequate. Instead, autoantibodies were determined against POMC as a precursor to α-MSH.
2.4. RadioBinding assay for ppHypocretin, HCRTR2, POMC, TRIB2, DP1, KIR4.1 and ANO2 autoantibodies
35S-labelled proteins obtained through coupled in-vitro transcription translation were used as antigens for the radiobinding assays [Citation45]. Serum samples from NT1 patients, FDR and healthy blood donors were incubated overnight (4 °C) in duplicates (2.5 µl/sample) with 60 µl radiolabelled protein diluted in assay buffer (Tris-buffered saline (pH 7.4), 0.15% polysorbate 20, 0.1% bovine serum albumin) corresponding to a total of 24.000 cpm (400 cpm/µl). A samples volume of 50 µl was transferred together with 50 µl Protein A Sepharose (diluted to 20% in assay buffer) (Zymed Laboratories Inc., San Francisco, CA) to pre-coated (with assay buffer) filtration plates (Millipore, Solna, Sweden). Due to problems with high non-specific binding in blank analyses for ppHypocretin and POMC, the filtration plates used for the analyses were pre-coated with 5% powder-milk diluted in assay buffer (Semper, Sundbyberg, Sweden). Plates were incubated 90 min (+4 °C) and washed three times in a microplate washer (ELx50TM Microplate Strip Washer, Biotek Instruments, Inc. Winooski, VT) using TBST (Tris-buffered saline (pH 7.4), 0.15% polysorbate 20) to separate unbound labelled antigen from Protein A Sepharose-bound antigen-antibody immunecomplexes. An aliquot of 50 µl Ultima gold LSC cocktail (PerkinElmer, Waltham, MA) was added per sample and levels of 35S-labelled ppHypocretin/HCRTR2/TRIB2/POMC/DP1/KIR4.1/ANO2 antibody bound antigens were counted in beta counter (Wallac Microbeta Trilux beta counter, Perkin Elmer, Waltham, MA). The Sepharose-bound radioactivity was converted to arbitrary Units/ml (U/ml) using as positive controls commercially available rabbit or sheep antisera as outlined in Supplementary Table 2 (diluted in a human negative control serum).
A smaller DP1 experiment with four NT1 patients and four of their FDR (three siblings and one parent, all Pandemrix®-vaccinated and associated to HLA DQB1*06:02) was conducted as the ligand to DP1 was added in concentration-dependent steps to the assay with the 35S-radiolabeled receptor. It was hypothesised that interaction with ligand would induce a conformational change to the receptor to possible modify the autoantibody binding repertoire.
2.5. RadioBinding assay for Hypocretin 1 and 2 autoantibodies
Commercially obtained radiolabeled 125I-Hypocretin 1 and 2 antigens (Phoenix Pharmaceuticals INC, Burlingame, CA) were analyzed as described in detail above. Serum samples were incubated in duplicates (7 µl/sample) in 36 µl radiolabelled antigen diluted in assay buffer corresponding to a total of 21.600 cpm (600 cpm/µl). After 48 h incubation (+4 °C) samples were transferred together with 40% Protein A Sepharose to filterplates (coated with assay buffer) and incubated in 90 min before wash (using TBST) in a microplate washer. Removal of the antigen complexes from filter plates to γ-counting columns via the addition of assay (three times per sample á 200 µl TBST) was followed by measurements in an automatic Gamma counter (Perkin Elmer, Waltham, MA). Hypocretin 1 and 2 autoantibody levels were converted to arbitrary Units/ml (U/ml) using commercially obtained rabbit hypocretin sera (anti-hypocretin 1 and anti-hypocretin 2, Phoenix Pharmaceuticals INC., Burlingame, CA) for standard and positive control.
2.6. Radioautography and SDS-PAGE
In vitro transcription-translated antigens were verified by 16% sodium dodecyl sulphate (SDS) polyacrylamide gel electrophoresis (PAGE) (ClearPageTM precast 16%, VWR, West Chester, PA). Antigens were diluted in ddH2O corresponding to a total of 1.2–1.5 × 105 counts per minute (cpm). Diluted antigens (10 µl) were mixed with 3.5 µl sample buffer and 3 µl reducing buffer (ClearPage™), heat incubated for 10 min (+70 °C) and applied onto the gel. Molecular weight ladder (14 C-methylated, NEC811, PerkinElmer Life Sciences, Waltham, MA), 10 µl, was mixed with 7 µl sample buffer and 6 µl reducing buffer (ClearPage™), and heat-incubated for 4 min (+100 °C). Furthermore, 5 µl unlabelled prestained protein ladder (PageRulerTM Plus (SM1811) Fermentas, Waltham, MA) was also loaded onto the gel. Electrophoresis ran (SDS running buffer, cassette) first by 50 V for 10 min, followed by 90 V for 1 h. The gel was washed with 212.5 ml ddH2O (42.5%), 200 ml ethanol (40%), 50 ml glycerol (10%) and 37.5 ml acetic acid, and dried on gel drying film (Promega, Madison, WI). Autoradiography was performed in dark room, placing the gel in a cassette on X-ray-sensitive film (Amersham HyperfilmTM ECL, GE Healthcare Bio-Sciences AB, Pittsburgh, PA) for 24 h. Developing mix (DAB Dental, Malmö, Sweden) and Fixation mix (DAB Dental) were used to visualize the autoradiography ().
Figure 1. Verification of molecular weight and protein sizes by SDS-PAGE electrophoresis and radio autography. From left to right: (A) 125I-Hypocretin 2 (2.89 kDa), 125I-Hypocretin 1 (3.58 kDa), 35S-POMC (27.25 kDa), 35S-TRIB2 (38.8 kDa); (B) 35S-KIR4.1 (42.51 kDa), 35S-HCTR2 (32.66 kDa), 35S-ppHypocretin (13.37 kDa); (C) 35S-ANO2 (95.13 kDa), and 35S- DP1 (40.3 kDa).
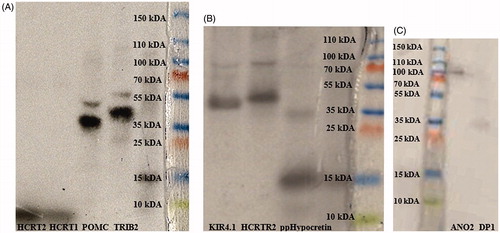
2.7. Statistics
Statistical analyses were performed using IBM® SPSS® Statistic, Version 25 (IBM. Corp. Armonk, NY) and GraphPad Prism, version 7 (GraphPad Software, Inc. La Jolla, CA). Median levels between groups were compared using Mann–Whitney’s U test. The significance threshold in the study was set to 0.05.
3. Results
3.1. Autoantibodies of the hypocretin transmitter system
This study determined conformational-dependent autoantibodies towards all components in the hypocretin signalling pathway. Autoantibodies were low and did not differ between NT1 patients compared with FDR for ppHypocretin (p = .576, ), 125I-HCRT1 (p = 1, ), 125I-HCRT2 (p = .33, ) or HCRTR2 (p = .145, ).
Figure 2. Autoantibodies to putative NT1 autoantigens. Comparison between narcolepsy patients and their first-degree relatives. Analyses of serum autoantibodies in narcolepsy patients (n = 31) and first-degree relative healthy family members (n = 66). Horizontal lines indicate median levels of autoantibodies and the dotted lines indicate cut-off level based on the analysis of serum samples from healthy blood donors, samples ascertained in 2008 (n = 200). Each dot represents one study participant. Subjects with autoantibody levels above the dotted line are considered positive. (A) Median levels of ppHypocretin autoantibodies were 2 (1–6) U/ml for NT1 patients and 2 (1–5) U/ml for controls in the family study (p = .576). No NT1 patients or FDR were considered positive for ppHypocretin autoantibodies. (B) Median autoantibody levels towards HCRT1 were 1 U/ml for both the NT1 patients and FDRs (p = 1). No NT1 patients or FDR were considered positive for HCRT1 autoantibodies. (C) Median levels of HCRT2 autoantibodies were low and did not differ between patients 1 (1) U/ml and FDRs 1 (1–3) U/ml (p = .33). No NT1 patients or FDR were considered positive for HCRT2 autoantibodies. (D) Median levels of HCRTR2 autoantibodies for NT1 patients were 2 (1–6) U/ml compared to 2 (1–10) U/mL for FDRs (p = .145). No NT1 patients or FDR were considered positive for HCRTR2 autoantibodies. (E) Median levels of TRIB2 autoantibodies were 5 (1–26) U/ml among NT1 patients compared to 6 (1–28) U/ml among FDRs (p = .286). Both NT1 patients and FDR were considered negative for TRIB2 autoantibodies. (F) The median levels for POMC/α-MSH autoantibodies were comparable between NT1 patients 1 (1–2) U/ml and FDRs 1 (1–5) U/ml (p = .371). Both NT1 patients and FDR were considered negative for POMC/α-MSH autoantibodies. (G) Median autoantibody levels for DP1 were comparable between patients 4 (1–14) U/ml and FDRs 4 (1–21) U/ml (p = .782). Two FDRs were considered positive for DP1 autoantibodies. (H) DP1 autoantibody levels were found to be consistent in both patients and FDRs, and not dependent on concentrations of DP1-ligand. (I) Median KIR4.1 autoantibody levels were 16 U/ml (1–391) U/ml for NT1 patients and 16 U/ml (1–622) U/ml for FDRs (p = .804). One patient and one FDR, not belonging to the same family, were considered positive for ANO2 autoantibodies. (J) ANO2 autoantibody levels were comparable between patients 1 (1–11) U/ml and FDRs 1 (1–12) U/ml (p = .572). Both patients and FDRs were negative for KIR4.1 autoimmunity.
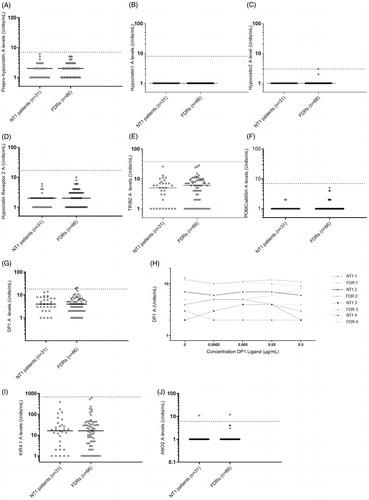
A cut-off limit for positivity was determined for each antigen based on autoantibody levels among healthy blood donors (ppHypocretin- 7 U/ml-98.74th percentile; HCRT1- 8 U/ml-98.25th percentile; HCRT2- 3 U/ml-99.25th percentile; HCRTR2- 17 U/ml-98.24th percentile (Supplementary Figure 1(A–D)). Neither NT1 patients nor FDR was considered positive for autoantibodies against hypocretin neurotransmitter system antigens.
3.2. Autoantibodies to proteins previously associated to NT1: TRIB2, POMC/α-MSH and DP1
The median levels of autoantibodies to TRIB2 (p = .286, ), POMC/α-MSH (p = .371, ) and DP1 (p = .782, ) were comparable between NT1 patients and FDR.
Autoantibody levels from healthy blood donors were used to determine cut-off limits for positivity (TRIB2-38U/ml-98.75th percentile; POMC/α-MSH- 6 U/ml-97.25th percentile; DP1- 18 U/ml-97.73th percentile, Supplementary Figure 1(E–G)). Two FDR were considered positive for DP1 autoantibodies. All study participants were considered negative for TRIB2 and POMC/α-MSH autoantibodies.
The DP1 experiment in which receptor ligand was added in concentration-dependent steps revealed that autoantibody levels were found to be consistent as no fluctuations could be demonstrated for neither patients nor FDR ().
3.3. Autoantigens suggested for MS as another neurological diseases associated to HLA DQB1*06:02; KIR4.1 and ANO2
Median autoantibodies levels to KIR4.1 (p = .804, ) and ANO2 (p = .572, ) were comparable between NT1 patients and FDR.
The cut-off limits for positivity were determined based on healthy blood donors (KIR4.1- 684 U/ml-99.24th percentile; ANO2- 6 U/ml-97.73th percentile, Supplementary Figure 1(H,I)). One patient and one FDR, not belonging to the same family, were considered positive for ANO2 autoantibodies. Both patients and FDR were negative for KIR4.1 autoimmunity.
4. Discussion
The present study could not confirm humoural autoimmunity to any of the candidate antigens of the hypocretin transmitter system. Two recent studies found autoreactive T-cells against hypocretin peptides to be linked to NT1 [Citation9,Citation10]. Further studies are warranted to clarify if the immune response in NT1 is HLA DR rather than DQ-restricted [Citation10] as well as if NT1-autoimmunity is restricted towards c-amidated but not against naive HCRT peptides [Citation9].
The current study could not associate NT1 to autoantibodies against TRIB2, POMC/α-MSH, DP1, KIR4.1 or ANO2. TRIB2 is the only NT1-associated autoantigen for which the results from the initial study have been re-confirmed by other authors [Citation17–Citation19]. Studies suggesting TRIB2 as an autoantigen for NT1 have been able to detect autoantibody positivity among 16–26% of studied narcolepsy cases, proposing the autoantigen to be present in a subgroup of patients. Results in the current study were consistent with previous reports from our lab which could not demonstrated increased levels of TRIB2 autoantibodies among patients with Pandemrix®-induced narcolepsy [Citation20]. It is recognized for many autoimmune diseases, including type 1 diabetes [Citation38], Graves’ disease, Hashimoto’s thyroiditis and Addison’s disease [Citation39,Citation40], that autoantibodies predominately detect conformational epitopes. The advantage of the current study is the use of in vitro transcription translation for expression of proteins together with radiobinding assay which enable detection of such conformational dependent autoantibodies [Citation46]. A possible limitation with the use of RBA is the consideration of post-translational modifications (PTMs), mechanisms linked to autoimmunity [Citation47].
PpHypocretin, HCTR2, POMC/α-MSH, DP1, KIR4.1 and AN02 are all transmembrane-spanning proteins in vivo. In line with the argument of the importance of conformational integrity [Citation41–Citation43], it could be argued that the technique used in the current project to express these transmembrane receptors as soluble proteins (to exclude membrane regions as those are prone to causing high background in the assay) affect the folding to alter the surface-exposed epitopes. What contradicts this argument is the strong reactivity to commercially obtained rabbit anti-sera against all tested autoantigens. For the NT1 candidate-autoantigens in the current study, with transmembrane parts excluded, it should be noted that the suggested antigenic epitope sequences in previous studies were included (PpHypocretin – aa54-66 “HGAGNHAAGILTL” and aa86-97 “ASGNHAAGILTM” [Citation9]; HCRTR2- aa34-45 “YDDEEFLRYLWR” [Citation16]; POMC- sequence coding for α-MSH aa138-150 “SYSMEHFRWGKPV” [Citation21]; AN02- aa136–147, “HAGGPGDIELGP” [Citation37]. For DP1 and KIR4.1 full-length receptors were expressed with transmembrane regions included. To increase the incorporation rate of 35S-methionine during in vitro translation, we excluded transmembrane sequences. It can, therefore, not be excluded that epitopes detected by sera from NT1 patients have been lost. Blood samples from the studied patients were obtained a long time after disease onset; six to eight years after vaccination with Pandemrix®. It could be argued that the low levels of autoantibodies in blood serum are associated with late time of analysis. It is noted in the current study that autoantibody levels diverse for different antigens among healthy subjects. First it should be recognized that arbitrary units for each autoantibody are determined individually, direct comparison between different assays is, therefore, not exact. Second existence of these natural autoantibodies is previously established, although there putative contribution to autoimmunity is not clarified (for review see [Citation48]).
Unfortunately, there have been many different suggestions of autoantigen in the field of narcolepsy, either with negative replication studies or lack of such studies. Equally important as to search for new autoantigens is to follow up and try to replicate previous ones. In the case of the current study, we are reporting negative outcome to possibly exclude previously proposed candidates. It is argued that NT1 is a T cell-mediated disease [Citation9,Citation10]. Involvement of B cells and autoantibodies in the disease pathogenesis might be limit or secondary to T cell initiated hypocretin degeneration. Such scenario would be in agreement with the current findings.
In conclusion, the autoimmune mechanisms behind Pandemrix®-induced NT1 are still unexplained as this study failed to reproduce NT1-specific autoantibody reactivity to nine putative antigenic targets.
Suppl_FIG1.jpg
Download JPEG Image (406.9 KB)Acknowledgement
The authors would like to acknowledge all NT1 patients and their family members that have donated their blood to make this study possible. The Swedish Narcolepsy Association (Narkolepsiföreningen Sverige) is thanked for inviting us to one of their meetings to present the study and to inform their members about the opportunity to participate in the study. Thanks to Ida Jönsson and Falastin Salami for expert technical assistance.
Disclosure statement
The authors report no conflict of interest.
Additional information
Funding
References
- Szakacs A, Darin N, Hallbook T. Increased childhood incidence of narcolepsy in western Sweden after H1N1 influenza vaccination. Neurology. 2013;80:1315–1321.
- Persson I, Granath F, Askling J, et al. Risks of neurological and immune-related diseases, including narcolepsy, after vaccination with Pandemrix: a population- and registry-based cohort study with over 2 years of follow-up. J Intern Med. 2014;275:172–190.
- Nohynek H, Jokinen J, Partinen M, et al. AS03 adjuvanted AH1N1 vaccine associated with an abrupt increase in the incidence of childhood narcolepsy in Finland. PLoS One. 2012;7:e33536.
- Tafti M, Hor H, Dauvilliers Y, et al. DQB1 locus alone explains most of the risk and protection in narcolepsy with cataplexy in. Europe Sleep. 2014;37:19–25.
- Mignot E, Lin L, Rogers W, et al. Complex HLA-DR and -DQ interactions confer risk of narcolepsy-cataplexy in three ethnic groups. Am J Hum Genet. 2001;68:686–699.
- Kornum BRS, Knudsen HM, Ollila F, et al. Narcolepsy. Nat Rev Dis Primers. 2017;3:16100.
- Thannickal TC, Moore RY, Nienhuis R, et al. Reduced number of hypocretin neurons in human narcolepsy. Neuron. 2000;27:469–474.
- Wang C, Wang Q, Ji B, et al. The Orexin/Receptor System: molecular mechanism and therapeutic potential for neurological diseases. Front Mol Neurosci. 2018;11:220.
- Luo G, Ambati A, Lin L, et al. Autoimmunity to hypocretin and molecular mimicry to flu in type 1 narcolepsy. Proc Natl Acad Sci USA. 2018;115:E12323–e12332.
- Latorre D, Kallweit U, Armentani E, et al. T cells in patients with narcolepsy target self-antigens of hypocretin neurons. Nature. 2018;562:63–68.
- Tanaka S, Honda Y, Inoue Y, et al. Detection of autoantibodies against hypocretin, hcrtrl, and hcrtr2 in narcolepsy: anti-Hcrt system antibody in narcolepsy. Sleep. 2006;29:633–638.
- Knudsen S, Mikkelsen JD, Jennum P. Antibodies in narcolepsy-cataplexy patient serum bind to rat hypocretin neurons. Neuroreport. 2007;18:77–79.
- Martinez-Rodriguez JE, Sabater L, Graus F, et al. Evaluation of hypothalamic-specific autoimmunity in patients with narcolepsy. Sleep. 2007;30:27–28.
- Luo G, Lin L, Jacob L, et al. Absence of anti-hypocretin receptor 2 autoantibodies in post pandemrix narcolepsy cases. PLoS One. 2017;12:e0187305.
- Giannoccaro MP, Waters P, Pizza F, et al. Antibodies against hypocretin receptor 2 are rare in narcolepsy. Sleep. 2017;40.
- Ahmed SS, Volkmuth W, Duca J, et al. Antibodies to influenza nucleoprotein cross-react with human hypocretin receptor 2. Sci Transl Med. 2015;7:294ra105.
- Toyoda H, Tanaka S, Miyagawa T, et al. Anti-Tribbles homolog 2 autoantibodies in Japanese patients with narcolepsy. Sleep. 2010;33:875–878.
- Kawashima M, Lin L, Tanaka S, et al. Anti-Tribbles homolog 2 (TRIB2) autoantibodies in narcolepsy are associated with recent onset of cataplexy. Sleep. 2010;33:869–874.
- Cvetkovic-Lopes V, Bayer L, Dorsaz S, et al. Elevated Tribbles homolog 2-specific antibody levels in narcolepsy patients. J Clin Invest. 2010;120:713–719.
- Lind A, Ramelius A, Olsson T, et al. A/H1N1 antibodies and TRIB2 autoantibodies in narcolepsy patients diagnosed in conjunction with the Pandemrix vaccination campaign in Sweden 2009–2010. J Autoimmun. 2014;50:99–106.
- Bergman P, Adori C, Vas S, et al. Narcolepsy patients have antibodies that stain distinct cell populations in rat brain and influence sleep patterns. Proc Natl Acad Sci USA. 2014;111:E3735–E3744.
- Sadam H, Pihlak A, Kivil A, et al. Prostaglandin D2 receptor DP1 antibodies predict vaccine-induced and spontaneous narcolepsy type 1: large-scale study of antibody profiling. EBioMedicine. 2018;29:47–59.
- Häggmark-Månberg A, Zandian A, Forsström B, et al. Autoantibody targets in vaccine-associated narcolepsy. Autoimmunity. 2016;49:421–433.
- Swaab DF. Neuropeptides in hypothalamic neuronal disorders. Int Rev Cytol. 2004;240:305–375.
- Nixon JP, Mavanji V, Butterick TA, et al. Sleep disorders, obesity, and aging: the role of orexin. Ageing Res Rev. 2015;20:63–73.
- Juvodden HT, Alnaes D, Lund MJ, et al. Widespread white matter changes in post-H1N1 narcolepsy type 1 patients and 1st degree relatives. Sleep. 2018;41.
- Park YK, Kwon OH, Joo EY, et al. White matter alterations in narcolepsy patients with cataplexy: tract-based spatial statistics. J Sleep Res. 2016;25:181–189.
- Srivastava R, Aslam M, Kalluri SR, et al. Potassium channel KIR4.1 as an immune target in multiple sclerosis. N Engl J Med. 2012;367:115–123.
- Zhong R, Liang J, Tao A, et al. Anti-KIR4.1 antibodies in Chinese patients with central nervous system inflammatory demyelinating disorders. Neuroimmunomodulation. 2016;23:295–300.
- Navas-Madronal M, Valero-Mut A, Martinez-Zapata MJ, et al. Absence of antibodies against KIR4.1 in multiple sclerosis: a three-technique approach and systematic review. PLoS One. 2017;12:e0175538.
- Higuchi O, Nakane S, Sakai W, et al. Lack of KIR4.1 autoantibodies in Japanese patients with MS and NMO. Neurol Neuroimmunol Neuroinflamm. 2016;3:e263.
- Brill L, Goldberg L, Karni A, et al. Increased anti-KIR4.1 antibodies in multiple sclerosis: could it be a marker of disease relapse? Mult Scler. 2015;21:572–579.
- Marino M, Frisullo G, Di Sante G, et al. Low reliability of anti-KIR4.183-120 peptide auto-antibodies in multiple sclerosis patients. Mult Scler. 2018;24:910–918.
- Marnetto F, Valentino P, Caldano M, et al. Detection of potassium channel KIR4.1 antibodies in multiple sclerosis patients. J Immunol Methods. 2017;445:53–58.
- Nerrant E, Salsac C, Charif M, et al. Lack of confirmation of anti-inward rectifying potassium channel 4.1 antibodies as reliable markers of multiple sclerosis. Mult Scler. 2014;20:1699–1703.
- Schirmer L, Srivastava R, Kalluri SR, et al. Differential loss of KIR4.1 immunoreactivity in multiple sclerosis lesions. Ann Neurol. 2014;75:810–828.
- Ayoglu B, Mitsios N, Kockum I, et al. Anoctamin 2 identified as an autoimmune target in multiple sclerosis. Proc Natl Acad Sci USA. 2016;113:2188–2193.
- Zlegler B, Strebelow M, Rjasanowski I, et al. A monoclonal antibody-based characterization of autoantibodies against glutamic acid decarboxylase in adults with latent autoimmune diabetes. Autoimmunity. 1998;28:61–68.
- Jastrzebska-Bohaterewicz E, Gardas A. Proportion of antibodies to the A and B immunodominant regions of thyroid peroxidase in Graves and Hashimoto disease. Autoimmunity. 2004;37:211–216.
- Grennan Jones F, Ziemnicka K, Sanders J, et al. Analysis of autoantibody epitopes on human thyroid peroxidase. Autoimmunity. 1999;30:157–169.
- Liu E, Eisenbarth GS. Accepting clocks that tell time poorly: fluid-phase versus standard ELISA autoantibody assays. Clin Immunol. 2007;125:120–126.
- Winqvist O, Karlsson FA, Kampe O. 21-Hydroxylase, a major autoantigen in idiopathic Addison's disease. Lancet. 1992;339:1559–1562.
- Pihoker C, Gilliam LK, Hampe CS, et al. Autoantibodies in diabetes. Diabetes. 2005;54(Suppl 2):S52–S61.
- Johansson J, Becker C, Persson NG, et al. C-peptide in dried blood spots. Scand J Clin Lab Invest. 2010;70:404–409.
- Grubin CE, Daniels T, Toivola B, et al. A novel radioligand binding assay to determine diagnostic accuracy of isoform-specific glutamic acid decarboxylase antibodies in childhood IDDM. Diabetologia. 1994;37:344–350.
- Jonsdottir B, Larsson C, Lundgren M, et al. Childhood thyroid autoimmunity and relation to islet autoantibodies in children at risk for type 1 diabetes in the diabetes prediction in skåne (DiPiS) study. Autoimmunity. 2018;51:228–237.
- Doyle HA, Yang M-L, Raycroft MT, et al. Autoantigens: novel forms and presentation to the immune system. Autoimmunity. 2014;47:220–233.
- Mannoor K, Xu Y, Chen C. Natural autoantibodies and associated B cells in immunity and autoimmunity. Autoimmunity. 2013;46:138–147.