Abstract
Introduction: As it is generally known, regulatory B cells (Bregs) control inflammation and autoimmunity. The significance of Bregs in the population of children with autoimmune thyroid diseases (AITD) still offers plenty of potential to explore. The aim of this study was to estimate the expression of Bregs (phenotype CD19+CD24+CD27+IL-10+, CD19+IL-10+, CD1d+CD5+CD19+IL-10+ and CD1d+CD5+CD19+CD24+CD27+) in a paediatric cohort with AITD and in health controls.
Materials and methods: A total of 100 blood samples were obtained from 53 paediatric patients with Graves’ disease (GD) (N = 12 newly diagnosed, mean age 12.5 ± 3.5 and N = 17 during methimazole therapy, mean age 12.7 ± 4.4), Hashimoto’s thyroiditis (HT) (N = 10 newly diagnosed, mean age 13.3 ± 2.9 and N = 10 during L-thyroxine therapy, mean age 13.7 ± 3.4) and compared with healthy controls (C) (N = 15, mean age 13.1 ± 3.1). The expressions of the immune cell populations were analysed by four-color flow cytometry using a FASC Canto II cytometer (BD Biosciences).
Results: There was a decreasing tendency in the number of lymphocytes B producing IL-10 (B10) cells among all B lymphocytes and more widely, also among all lymphocytes, in each study group, as compared to C. We reported a reduction in IL-10 production in Bregs with the expression of CD19+CD24+CD27+IL-10 and CD1d+CD5+CD19+IL-10+ in both untreated and treated AITD.
Conclusions: Our data demonstrate that the reduction in the number of Bregs with CD19+CD24+CD27+IL-10+ and CD19+IL-10+ expression could be responsible for breaking immune tolerance and for AITD development in children.
Introduction
Autoimmune thyroid diseases (AITD) which include Graves’ disease (GD) and chronic lymphocytic thyroiditis, called Hashimoto’s thyroiditis (HT), account for approximately 30% of all autoimmune diseases [Citation1]. Despite a relatively low prevalence rate, they are observed to become increasingly common in the paediatric population. The most frequent cause of paediatric hypothyroidism is HT, whereas GD is responsible for the majority of hyperthyroidism entities in childhood, cognately [Citation2–4]. AITD is assumed to occur due to coexistence of genetic predisposition and environmental triggers that yield a breakdown in immune tolerance [Citation5]. Though autoimmunity still remains an unexplored topic, the reason why the thyroid gland is so susceptible to AITD may be the fact that thyroid cells produce many immunological factors, such as cytokines, inflammatory mediators or adhesion molecules, which actively interact with the immune system [Citation6].
The severity of the antigen-adaptive immune system may be regulated in the human body in a variety of ways [Citation7–9]. According to the classical paradigm, the helper subset of T lymphocytes, Th1 and Th2, produce cytokines supporting cellular immunity that predominates in HT, and are responsible for humoral regulations, characteristic of GD, respectively. More recently, in more detail, it is considered that Th1, Th17 and effector B lymhocytes (Beff) are responsible for the upregulation of immune responses. Adversely, T regulatory lymphocytes (Tregs) and B regulatory lymphocytes (Bregs) cooperate against spreading of inflammation. Abnormalities in the Th17/Treg proportion or expression of cytokines towards Beff as compared to Bregs may contribute to AITD development [Citation8,Citation10–12].
According to the available literature, Tregs, which generally express CD4 and CD25, play a critical role in preventing autoimmunization, which was first proved in mice and then in humans. The absence or defects of Tregs concur to autoimmune gastritis, thyroiditis, diabetes and inflammatory bowel disease. Surprisingly, the number of Tregs may not be decreased restrictively in some tissues or may even occur in excess, like in synovial fluid in patients with rheumatoid arthritis. Thus, not only dysfunction or depletion but also balance between Tregs and effector T cells contribute to autoimmunity [Citation8]. Clonal deletion of autoreactive lymphocytes takes place in thymus, whereas in peripheral lymphoid organs it is obtained via anergy or active suppression with involvement of T and B regulatory lymphocytes, all of which maintain immunological homeostasis [Citation9,Citation13].
Focussing our attention on the family of B lymphocytes, the variety of their functions includes production of antibodies, antigen-presenting capacity and release of cytokines [Citation14]. Promotion of inflammatory response constitutes the primary role of B-cells in humans. Traditionally, B-cells are responsible for humoral immunity via antigen-specific antibody production. Their other positive features include the regulation of CD8+ T cell responses, functioning of dendritic cells, inducing T cell activation or organization of lymphoid tissue. In the last decades intensified studies have brought a completely opposite view on their additional role. There are well supported proofs that B-cells are able to regulate immune responses in a negative way through the secretion of anti-inflammatory cytokines. Thus, B cells are considered to have both positive and negative regulatory roles in immunity [Citation15–17].
In this work, we would like to focus on Bregs, since the subject has been rarely undertaken.
Bregs represent 5–10% of the whole population of B lymphocytes (CD19) and are marked by a peculiar production of interleukin 10 (IL-10) and transforming growth factor beta (TGF-β), responsible for suppressing excessive immune responses. Additionally, these cytokines inhibit the expression of pro-inflammatory cytokines involved in the activation of Th1 and Th17 lymphocytes [Citation18]. Particularly IL-10 remains pivotal in immunity and every deregulation of its expression or signalling may result in autoimmune diseases [Citation19]. However, performing IL-10 intracellularly entails prolonged lab procedure (permeabilization) and prevents the use of these cells for further tests e.g. functional ones. Researches have recently detected a strong correlation between intracellular expression of IL-10 and superficial expression of antigen CD24 and CD27. Therefore, it can be assumed that B10 regulatory cells may present phenotype CD19+CD24hiCD27 + [Citation20]. Intensive studies have been performed on the role of Bregs in the pathogenesis and development of many systemic autoimmune disorders (diabetes mellitus, lupus, psoriasis), but not in the field of AITD [Citation21,Citation22].
CD19+CD24hiCD27+ producing IL-10 have the ability to inhibit the immune response dependent on Th1 and dendritic cells (presenting antigen). According to Zha B at al., this can reduce their number and/or impair their functionality in AITD in adults, thus limiting the mechanisms of inhibiting cytokine synthesis by Th1 [Citation23].
Little is known about functionality of B10 cells in the paediatric population. In one study, Bregs producing IL-10 were analysed and researches demonstrated that the B10 population seems to enhance during childhood as compared to newborns and adults. Moreover, they provided evidence for a decreased number of B10 in children with autoimmune disorders [Citation24]. Other authors aimed to investigate the linkage between Bregs and immune thrombocytopenic purpura (ITP) in children. They revealed a decreased number of Bregs in the peripheral blood in patients with ITP [Citation25].
Functional abnormalities of regulatory lymphocytes lead to a decline in tolerability of the body’s own tissues as a result, among others, of the production of antibodies reacting with particular structures of cells and tissues [Citation26]. It has been explicitly demonstrated that there is a strong relationship between loss of Bregs’ function and occurrence of autoimmune processes in animals [Citation27]. Their functional deficiency and impairment may promote autoimmune diseases. Studies performed on mice deprived of Bregs showed vulnerability to the development of organ-specific autoimmune diseases including encephalomyelitis, diabetes mellitus or arthritis. On the contrary, the bandwidth of lymphocytes from healthy adult individuals prevents these pathologies [Citation27–29]. The administration of lymphocytes lacking B10 conduced autoimmunization and the presence of B10 cells suppressed the process [Citation29].
Materials and methods
A total of 100 blood samples were obtained from 53 paediatric patients aged 5–19 years. The study groups demonstrated different autoimmune diseases: GD (N = 12 newly diagnosed, mean age 12.5 ± 3.5 and N = 17 during methimazole therapy, mean age 12.7 ± 4.4), HT (N = 10 newly diagnosed, mean age 13.3 ± 2.9 and N = 10 during L-thyroxine therapy, mean age 13.7 ± 3.4). All patient groups were compared to healthy controls (C, N = 15, mean age 13.1 ± 3.1). Informed consent was obtained from patients or their parents. The qualifying criteria for patients with AITD were based on clinical, laboratory and ultrasound investigations. GD was diagnosed in patients with large goitre, and hyperthyroidism was biochemically confirmed in a large number of samples with positive titres of Ab against the thyroid stimulating hormone receptor TSHR >1.7 (U/L), and positive titres of anti-TPO and/or anti-TG antibodies. Patients with HT demonstrated anti-TPO and/or anti-TG Ab, and had clinical and biochemical features of hypothyroidism requiring thyroid hormone replacement therapy or were euthyroid during therapy [Citation8]. Some of the patients were tested a few times during the follow-up period and were subsumed into both: untreated and treated groups. Most samples were checked for the following laboratory parameters: free thyroxine (fT4), free triiodothyronine (fT3), TSHR-Abs, anti-TG, and anti-TPO. All patients with GD were treated with methimazole as a basic therapy. L-Thyroxine was initiated in HT cases. All healthy C were euthyroid, had no personal or family history of any AITD and were negative for thyroid autoantibodies.
Assessment of the thyroid hormone concentration and antithyroid antibody titres
Blood was drawn and centrifuged for 10 min at 2000 rotations/min. Sera were stored at minus 85 C. Serum levels of fT4, fT3, and TSH were determined on electrochemiluminescence “ECLIA” with a Cobas e 411 analyser (Roche Diagnostics, Warszawa, Poland). Normal values for fT4 ranged between 0.71 and 1.55 ng/dl, for fT3 between 2.6 and 5.4 pg/ml and for TSH between 0.32 and 5.0 (mIU/ml). Anti-TPO, anti-TG antibodies and TSHR-Abs were measured in all samples using electrochemiluminescence “ECLIA” with a Modular Analytics E170 analyser (Roche Diagnostics, Warszawa, Poland). The negative values for antithyroid antibodies were: 1.7 U/l for TSHR-Ab, between 0 and 34 IU/ml for anti-TPO-Abs and between 0 and 115 IU/ml for anti-TG-Abs [Citation8].
Cell culture
EDTA-anticoagulated peripheral blood has been collected from children with AITD, and from healthy patients. Peripheral blood mononuclear cells (PBMC) were isolated by density-gradient centrifugation using Ficoll (Histopaque-1077, Sigma-Aldrich). Following isolation, around 1 x 106 PBMC were suspended in RPMI medium (RPMI-1640 Medium, Sigma-Aldrich, Missouri) supplemented with 10% Foetal Bovine Serum (Foetal Bovine Serum, Gibco) and 1% Penicillin/Streptomycin (Penicillin-Streptomycin, Sigma-Aldrich, Missouri). Subsequently, cells were incubated for 5 h at 37 °C in 5% CO2 in presence of 5 μg/ml TLR9 agonist (CpG ODN, InvivoGen) and 2 μl/ml of Leukocyte Activation Coctail with GolgiPlug (BD Bioscience) which contains: Phorbol 12-Myristate 13-Acetate (PMA), calcium ionophore (Ionomycin), the protein transport inhibitor (Brefeldin A). Stimulation procedure, with slight modifications, was established on the basis of previously described protocols for identification of B10 cells in peripheral blood [Citation15]. CpG induced B10 cells to be competent for IL-10 production, which was enhanced with PMA/ionomycin. Addition of Brefeldin A prevented from IL-10 secretion and improved detection efficacy. PBMC cultured in enriched RPMI medium alone served as a negative control – unstimulated leukocytes, to unambiguously define IL-10-negative B cells.
Immunostaining and flow cytometric analysis
Following incubation PBMC were collected and subjected to extracellular staining with fluorochrome conjugated monoclonal antibodies including: anti-CD1d APC (clone CD1d42), anti-CD5 PerCP-Cy5.5 (clone L17F12), anti-CD19 FITC (clone HIB19), anti-CD24 BV421 (clone ML5) and anti-CD27 APC-H7 (clone M-T271) (BD Biosciences). After incubation for 30 min. at room temperature, in the dark cells were washed and permeabilized prior to intracellular staining (BD FACS Permabilization Solution 2, BD Bioscience). For intracellular staining, anti-IL-10 PE (clone JES3-19F1) monoclonal antibodies were used. Next incubation for 30 min. (room temperature, in the dark cells) and washing step was followed by acquisition of data on FACSCanto II flow cytometer (BD Biosciences) and analysed with the use of FlowJo software (Tree Star Inc.) Regulatory B10 cells were distinguished within pool of CD19+ B on the basis of stimulation induced production of IL-10 (protocol for stimulation described in previous subsection regarding cell culture). CD1d, CD5, CD24 and CD27 expression markers were used to determine the full phenotype of B10 cells. Gating strategy of Bregs and IL-10+ assessment is demonstrated in .
Figure 1. Gating strategy of Bregs and IL-10+ assessment. (A) Gating strategy of particular lineage-specific surface markers. First of all, lymphocytes were distinguished on the basis of FSC and SSC properties within singlets of the PBMC population analysed, and then CD19+ cells were gated (upper row). Subsequently, IL-10 production was assessed within CD19+ lymphocytes or additional gating of CD1d + CD5+ and CD24 + CD27+ cells was performed (middle row) to allow for evaluation of IL-10-producing B cells within CD19 + CD1d + CD5+, CD19 + CD24 + CD27+ and CD19 + CD1d + CD5 + CD24 + CD27+ population subsequently (lower row). (B) IL-10+ assessment in control group. (C) IL-10+ assessment in untreated GD. (D) IL-10+ assessment in untreated HT.
Statistical analysis
Statistical analysis of the acquired data was performed with the use of GraphPad Prism 5.01 software (GraphPad Software Inc., San Diego, CA). The differences between unrelated groups were analysed using non-parametric Mann-Whitney test, and Wilcoxon matched pairs test in reference to data from patients before and after treatment. The results were presented on the graphs as mean values with standard error of the mean (SEM). Pearson and Spearman correlation analyses were applied to verify the correlation. All probability values were two-tailed, and a value of <.05 was considered statistically significant. The levels of significance were indicated with asterisks: *p < .05, **p < .01.
Results
The demographic and serological data of 53 paediatric patients aged 5 year to 19 years (mean: 12.9 ± 1) are shown in .
Table 1. Demographic and serological data of all 53 investigated patients.
No relevant differences were noted in mean age distribution between all study groups. All C patients were negative for thyroid-related autoantibodies and had baseline levels of thyroid hormones within the normal range.
Untreated GD patients revealed high free triiodothyronine (fT3), mean: 8.4 ± 5.8 pg/ml, free thyroxine (fT4), mean: 2.9 ± 1.7 levels and elevated levels of anti-TSH receptor antibodies (TRAb), mean 13.9 ± 12.5, anti-thyroid peroxidase antibodies (anti-TPO-Ab), mean: 235.0 ± 198.2 and anti-thyroglobulin antibodies (anti-TG-b), mean: 941.6 ± 1408.0.
Patients with untreated HT were in hypothyroidism, with the following mean hormone levels: fT3 mean: 2.3 ± 1.1, fT4 mean: 0.6 ± 0.5, TSH mean: 11.9 ± 11.1 and with elevated concentration of anti-thyroid antibodies: anti-TPO-Ab mean: 438.3 ± 172.5, anti-TG-Ab mean:943.4 ± 1253.8. Positivity and mean values of anti-thyroid antibodies are demonstrated in .
Primarily, we assessed the percent of B10 in the whole lymphocyte group. We detected a significant depletion in the number of CD19+IL-10+ in untreated GD (p < .05), treated GD (<.01), untreated HT (p < .05) and treated HT (<.05) in comparison with C. The frequency of CD19+CD24+CD27+IL-10+ was significantly decreased in GD untreated and HT treated groups (p < .05), CD1d+CD5+CD19+IL-10+ in treated GD (p < .01), and the number of CD1d+CD5+CD19+CD24+CD27+ IL-10+ was not significantly decreased in all groups ().
Figure 2. Presentation of alterations in frequencies of different IL-10-producing B and regulatory B cells within total lymphocytes (A-D) and CD19+ B cells (E-H) in study groups including: CD19+IL-10+ (A, E), CD19+CD24+CD27+IL-10+ (B, F), CD1d+CD5+CD19+IL-10+ (C, G), CD1d+CD5+CD24+CD27+IL-10+ (D, H).
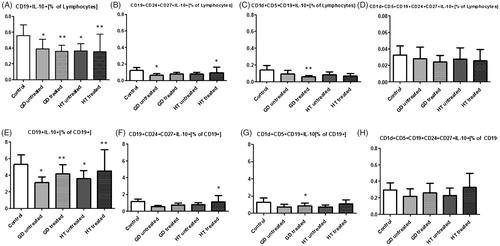
Restricting the issue, among all B lymphocytes (antigen CD19+) we similarly reported a significant decrease in the number of B lymphocytes producing IL-10 in GD untreated group (p < .05), GD treated group (p < .01), HT untreated group (p < .05) and in HT treated group (p < .01), as compared to C. CD19+CD24+CD27+IL-10+ in the whole CD19+ group was notably decreased only in treated HT (p < .05). CD1d+CD5+CD19+IL-10+ was significantly decreased in treated GD (p < .05). No positive correlation was detected between number of CD1d+CD5+CD19+CD24+CD27+IL-10+ subset in all patient groups and healthy individuals ().
When concentrating on the functionality of Bregs, which can be estimated by investigating the quantity of IL-10, there was a notable depletion in the production of IL-10 in CD19 + IL-10+ in untreated GD and HT (p < .05), treated GD and HT (p < .01) and in CD19+CD24+CD27+ and CD1d+CD5+CD19+ in all the study groups (p < .05). In the case of CD1d+CD5+CD19+CD24+CD27+ a significant decrease was noted in treated GD and treated HT groups (p < .01) ().
Figure 3. Graphical presentation of changes in IL-10 production within total CD19+ B cells (A) and 3 different phenotypes of regulatory B cells: CD24+CD27+CD19+ (B); CD1d+CD5+CD19+ (C); CD1d+CD5+CD19+CD24+CD27+ (D).
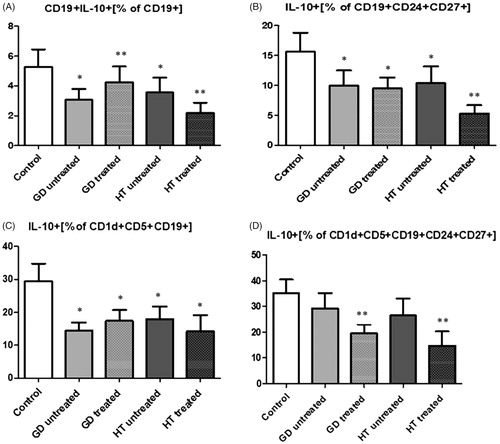
The levels of CD19+CD24+CD27+, CD1d+CD5+CD19+, CD1d+CD5+CD19+CD24+CD27+ relative to all lymphocytes and to all B lymphocytes (CD19+) were not significantly decreased ().
Figure 4. Changes in percentage of putative phenotypes of regulatory B cells within lymphocytes (row above) and CD19+ B cells (row below) in study groups: CD19+CD24+CD27+ (A, D), CD1d+CD5+CD19+ (B, E), CD1d+CD5+CD24+CD27+CD19+ (C, F).
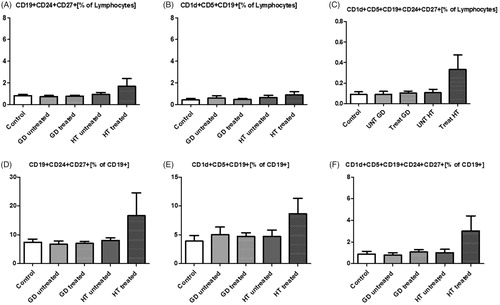
The relationship between thyroid homones and anti-thyroid antibodies and the proportions of Breg cells was evaluated (Supplementary Table 2). In patients with untreated GD, a statistically significant correlation was found between the proportions of the production of IL-10+ by CD19+CD24+CD27+ (p < .05), CD1d+CD5+CD19+CD24+CD27+IL-10+ (p < .05) and CD1d+CD5+CD19+CD24+CD27+ (p < .01) in the whole lymphocyte group and the level of anti-TG. Such relationship in this group was observed only for TSH, in the case of IL-10+ production by CD1d+CD5+CD19+CD24+CD27+ (p < .01). In patients with untreated HT, a correlation was detected between the concentration of TSH and the proportion of CD19+CD24+CD27+ (p < .05). Further, the correlation was found between the levels of fT4 and the ratio of IL-10+ production by CD19+CD24+CD27+ (p < .05), CD1d+CD5+CD19+ (p < .05) and the amount of B10 (CD19+) in the whole lymphocyte group (p < .05), CD19+CD24+CD27+IL-10+ (p < .05), CD19+CD24+CD27+. Patients with treated GD demonstrated a positive correlation between the level of anti-TG and anti-TPO and the quantity of CD1d+CD5+CD19+CD24+CD27+ (p < .05). In this group, a correlation was also noted between the levels of fT3 and fT4 and almost all the investigated Bregs phenotypes: CD19+IL-10+, CD19+CD24+CD27+IL-10+, CD1d+CD5+CD19+IL-10+, CD1d+CD5+CD19+CD24+CD27+IL-10+, as well as with the production of IL-10+ by CD19+CD24+CD27+ (p < .05). No significant correlation was found between the thyroid hormones and anti-thyroid antibodies and the proportion of Bregs in patients with treated HT.
Discussion
The undisturbed process of inflammation is the foundation of satisfactory functioning of the immune system. Its self-limiting nature, which is due to the presence of special mediators and cytokines [Citation30] is the major characteristics of the inflammatory response. Maintaining balance between pro-inflammatory and anti-inflammatory mechanisms depends on contrary regulations provided by different types of cells. The role of cytokines and chemokines in AITD is strongly emphasized in the latest research [Citation1,Citation31].
As mentioned above, B cells that display regulatory functions and produce immunomodulatory cytokines are called Bregs [Citation32]. Apart from human blood, they have been also detected in the spleen, tonsils and newborn cord blood. In spite of the fact that Bregs constitute a small percent of the whole family of B cells, there is currently no doubt that they play an essential role in immune homeostasis [Citation33–35].
The first study to develop the subject of B regulatory cells appeared in 1974 when the capacity of B-cells to suppress delayed-type hypersensitivity in guinea pigs was discovered [Citation36]. Among one of the further reports was the investigation of Janeway’s group in 1996, in which authors demonstrated a suppressive role of B lymphocytes in experimental autoimmune encephalomyelitis (EAE). Shortly thereafter similar conclusions were presented regarding chronic colitis. Subsequently, immunoregulatory capacity was shown in the murine model of collagen-induced arthritis (CIA) [Citation29,Citation36–38]. Lately, scientists have been trying to understand the role of Bregs in transplantation procedure. Until now the presence of Bregs has been confirmed to be associated with refined graft persistence [Citation39,Citation40]. The dual role of Bregs function is related to carcinogenesis. On the one hand, Bregs support tumour progression through modulation of immune responses. On the other, extensive studies have been devoted to the Breg-based immunotherapies in cancer treatment [Citation34,Citation35,Citation41,Citation42].
By contrast, Iwata et al. [Citation20] basing on their own research formulated a thesis that patients with specific autoimmune diseases such as lupus, rheumatoid arthritis, Sjögren syndrome, autoimmune skin disease or multiple sclerosis do not differ from healthy controls in the number of B10 cells. Further, they postulated that the production of IL-10 by B cells is even higher in patients with rheumatoid arthritis, lupus and systemic sclerosis.
In turn, when restricting the issue to the phenotype CD19+CD24hiCD27+ in accessible literature, an entirely different conclusion seems to appear. Jin et al. [Citation20] supported the suggestion that in patients with untreated systemic lupus erythematosus, the frequency and number of CD19+CD24hiCD27+ B cells significantly decrease and correlate with the activity and response to therapy in that particular disease.
As mentioned above, the term B10 cells refers strictly to Bregs that produce IL-10. This cytokine has a significant immunoregulatory potential for both innate and acquired immunity. It is involved in the resolution of inflammation during acute infections at the local and systemic level. It can inhibit the activity of Th1, natural killer cells and macrophages, so it may have equal capability to detract pathogen clearance as well as to prevent tissue damage. Thus, deregulation of the number or function of IL-10 may contribute to severe immunopathology [Citation19,Citation43,Citation44].
Presently, we can differentiate B- cells by many lineage-specific surface markers. According to literature, CD19+CD24hiCD38hi, CD19+CD24hiCD27+, CD19+CD5+CD1dhi and CD19+CD73negCD25+CD71+ phenotypes seem to be responsible just for the regulatory function [Citation45]. The issue we would like to particularly highlight in this work is the suspicion that an impaired function or a change in B10 cell count may influence the development of autoimmune disease in children. In more detail, we tried to assess specific subtypes of CD19+CD24+CD27+ and CD19+IL-10+ Bregs. Additionally, we also investigated other lineage-specific surface markers, such as CD1d+CD5+CD19+IL-10+ and CD1d+CD5+CD19+CD24+CD27+. To the best of our knowledge, it is the first study to present such results.
In the current study we demonstrate that the number of CD19+IL-10+ in the whole lymphocytes group and more precisely, in the B lymphocytes group, is decreased. In more detail, the count of CD19+CD24+CD27+IL-10+ was significantly reduced in untreated GD and treated HT what relates to the whole lymphocytes group, and in treated HT, regarding B cells group. In case of CD1d + CD5 + CD19 + IL-10+ we evidenced significant depletion only in treated GD both in all lymphocytes and B lymphocytes groups. Interestingly, we did not evaluated difference in the count of these phenotypes without IL-10+ expression. Apart from the fact of being treated or not, patients with AITD manifest lower frequency of Bregs comparing to healthy individuals.
Evaluation of Bregs functionality may be registered by their ability to express IL-10. Generally, our data demonstrate a decreasing tendency in the production of IL-10 among all B lymphocytes (CD19+) and more widely, also of all lymphocytes, in each study group, as compared to C. Moreover, a reduction was noted in the production of IL-10 in Bregs with the expression of CD19+CD24+CD27+ and CD1d+CD5+CD19+ in both untreated and treated AITD. In the case of CD1d+CD5+CD19+CD24+CD27+, an impairment was reported in IL-10 production in the treated GD and HT groups. This in itself allows us to confirm that the process of IL-10 release is disturbed in AITD.
Our findings can be compared to those reported by Zha et al. [Citation23], who concentrated on adult GD patients only, but showed likewise functional reduction in CD19+CD24hiCD27+ in new-onset GD. Possible reasons should be considered. One of the explanations may be a hypothesis that Bregs undergo exhaustion during autoimmune processes. As shown in Bossowski et al., on an example of T regulatory lymphocytes, they circulate and migrate to a specific tissue zone to perform effector function. We detected lymphocytes in the peripheral blood samples, not in the thyroid tissue being the target of lymphocytic infiltration and ultimate accumulation in AITD. We suggest that a change in the proportions of Bregs could be a result of lymphocytes’ inflow to the thyroid cells [Citation8,Citation46]. Even though we did not reveal any deficiency in the number of some specific surface markers in patients with AITD as compared to healthy individuals, functionality of Bregs appears to be a more valuable indicator of inflammatory process spread. Adequate or even elevated B10 count with a concomitant functional loss may result from the development of thyroid cell resistance to Bregs.
Untreated patients with GD had positive correlation between number of some subtypes of Bregs and the level of anti-TG antibodies. Higher anti-TG were accompanied by lower count of CD1d+CD5+CD19+CD24+CD27+IL-10+ and lower production of IL-10+ by CD19+CD24+CD27+. In turn, untreated HT group demonstrated correlation between concentration of fT4 and Bregs. Lower count of CD19 + IL-10+, CD19 + CD24 + CD27 + IL-10+ and production of IL-10+ by CD19 + CD24 + CD27+ and CD1d + CD5 + CD19+ was observed in the linkage with lower fT4. Thus, thyroid metabolic status may influence on the proportions of immune cells. It is still source of debate how treatment may impact the frequency of Bregs. Zha et al. [Citation23] demonstrated tendency to reversion to the status of a healthy individual after treatment with anti-thyroid drugs. In our case we cannot exclude that methimazole does not affect thyroid gland in the range of IL-10, but also via other mediators. What is more, our patients with GD, despite the applied therapy, experienced several recurrences and in many cases did not achieve stable euthyreosis. The disease exacerbation depends on many circumstances among which some are not modifiable. In the publication mentioned above, GD patients recovered and were treated with methimazole for at least 12 months. [Citation23,Citation47].
Despite a small number of patients involved, which is a limitation of our study, we revealed for the first time in literature that the number of Bregs and even more significantly, their functionality in the paediatric profile of AITD are disturbed. Further research with a larger group of samples is required to confirm the results published in this paper.
Our data demonstrate that the reduction in the number of Bregs with CD19+CD24+CD27+ IL-10+ and CD19+IL-10+ expression could be responsible for breaking the immune tolerance and AITD development in children. Details of regulation of immunological processes at the intracellular level may contribute not only to better recognition of AITD pathogenesis, but also to novel diagnostic, prognostic and therapeutic possibilities. We consider our results to be an open field for further investigations.
Correction Statement
This article has been republished with minor changes. These changes do not impact the academic content of the article.
Supplementary_table_2.docx
Download MS Word (14 KB)Acknowledgments
The authors thank Mrs Ewa Fiedorczuk for her valuable laboratory assistance.
Disclosure statement
No potential conflict of interest was reported by the authors.
Additional information
Funding
References
- Antonelli A, Ferrari SM, Corrado A, et al. Autoimmune thyroid disorders. Autoimmun Rev. 2015;14:174–180.
- Committee on Pharmaceutical Affairs, Japanese Society for Pediatric Endocrinology, and the Pediatric Thyroid Disease Committee, Japan Thyroid Association (Taskforce for the Revision of the Guidelines for the Treatment of Childhood-Onset Graves’ Disease); Minamitani K, Sato H, Ohye H, et al. Guidelines for the treatment of childhood-onset Graves' disease in Japan 2016. Clin Pediatr Endocrinol. 2017;26:29–62.
- De Luca F, Santucci S, Corica D, et al. Hashimoto's thyroiditis in childhood: presentation modes and evolution over time. Ital J Pediatr. 2013;39:8.
- Bossowski AT, Reddy V, Perry LA, et al. Clinical and endocrine features and long-term outcome of Graves' disease in early childhood. J Endocrinol Invest. 2007;5:388–392.
- Lee HJ, Li CW, Hammerstad SS, et al. Immunogenetics of autoimmune thyroid diseases: A comprehensive review. J Autoimmun. 2015;64:82–90.
- Saranac L, Zivanovic S, Bjelakovic B, et al. Why is the thyroid so prone to autoimmune disease? Horm Res Paediatr. 2011;75:157–165.
- Bossowski A, Moniuszko M, Dąbrowska M, et al. Lower proportions of CD4 + CD25(high) and CD4 + FoxP3, but not CD4 + CD25 + CD127(low) FoxP3+ T cell levels in children with autoimmune thyroid diseases. Autoimmunity. 2013;46:222–230.
- Bossowski A, Moniuszko M, Idźkowska E, et al. Decreased proportions of CD4 + IL17+/CD4 + CD25 + CD127- and CD4 + IL17+/CD4 + CD25 + CD127 - FoxP3+ T cells in children with autoimmune thyroid diseases. Autoimmunity. 2016;49:320–328.
- Ramos-Leví AM, Marazuela M. Pathogenesis of thyroid autoimmune disease: the role of cellular mechanisms. Endocrinol Nutr. 2016;63:421–429.
- Bliddal S, Nielsen CH, Feldt-Rasmussen U. Recent advances in understanding autoimmune thyroid disease: the tallest tree in the forest of polyautoimmunity. F1000Res. 2017;6:1776.
- Wang B, Shao X, Song R, et al. The emerging role of epigenetics in autoimmune thyroid diseases. Front Immunol. 2017;8:396.
- Phenekos C, Vryonidou A, Gritzapis AD, et al. Th1 and Th2 serum cytokine profiles characterize patients with Hashimoto’s thyroiditis (Th1) and Graves' disease (Th2). Neuroimmunomodulation. 2004;11:209–213.
- Mauri C, Blair PA. Regulatory B cells in autoimmunity: developments and controversies. Nat Rev Rheumatol. 2010;6:636–643.
- Wang P, Zheng SG. Regulatory T cells and B cells: implication on autoimmune diseases. Int J Clin Exp Pathol. 2013;6:2668–2674.
- Kalampokis I, Yoshizaki A, Tedder TF. IL-10-producing regulatory B cells (B10 cells) in autoimmune disease. Arthritis Res Ther. 2013;15:S1.
- Lykken JM, Candando KM, Tedder TF. Regulatory B10 cell development and function. Int Immunol. 2015;27:471–477.
- Mann MK, Ray A, Basu S, et al. Pathogenic and regulatory roles for B cells in experimental autoimmune encephalomyelitis. Autoimmunity. 2012;45:388–399.
- Pillai S, Mattoo H, Cariappa A. B cells and autoimmunity. Curr Opin Immunol. 2011;23:721–731.
- Iyer SS, Cheng G. Role of interleukin 10 transcriptional regulation in inflammation and autoimmune disease. Crit Rev Immunol. 2012;32:23–63.
- Iwata Y, Matsushita T, Horikawa M, et al. Characterization of a rare IL-10-competent B-cell subset in humans that parallels mouse regulatory B10 cells. Blood. 2011;117:530–541.
- Blair PA, Noreña LY, Flores-Borja F, et al. CD19(+)CD24(hi)CD38(hi) B cells exhibit regulatory capacity in healthy individuals but are functionally impaired in systemic Lupus Erythematosus patients. Immunity. 2010;32:129–140.
- Kristensen B. Regulatory B and T cell responses in patients with autoimmune thyroid disease and healthy controls. Dan Med J. 2016;63.
- Zha B, Wang L, Liu X, et al. Decrease in proportion of CD19+ CD24(hi) CD27+ B cells and impairment of their suppressive function in Graves' disease. PLoS One. 2012;7:e49835.
- Kalampokis I, Venturi GM, Poe JC, et al. The regulatory B cell compartment expands transiently during childhood and is contracted in children with autoimmunity. Arthritis Rheumatol. 2017;69:225–238.
- Zahran A, Aly S, Elabd A, et al. Regulatory and memory B lymphocytes in children with newly diagnosed immune thrombocytopenia. J Hematol. 2017;6:81–86.
- Mason D, Powrie F. Control of immune pathology by regulatory T cells. Curr Opin Immunol. 1998;6:649–655.
- Hussain S, Delovitch TL. Intravenous transfusion of BCR-activated B cells protects NOD mice from type 1 diabetes in an IL-10-dependent manner. J Immunol. 2007;179:7225–7232.
- Matsushita T, Yanaba K, Bouaziz JD, et al. Regulatory B cells inhibit EAE initiation in mice while other B cells promote disease progression. J Clin Invest. 2008;118:3420–3430.
- Mauri C, Gray D, Mushtaq N, et al. Prevention of arthritis by interleukin 10-producing B cells. J Exp Med. 2003;197:489–501.
- Rosser EC, Mauri C. Regulatory B cells: origin, phenotype, and function. Immunity. 2015;42:607–612.
- Bossowski A, Harasymczuk J, Moniuszko A, et al. Cytometric evaluation of intracellular IFN-γ and IL-4 levels in thyroid follicular cells from patients with autoimmune thyroid diseases. Thyroid Res. 2011;4:13.
- Mizoguchi A, Bhan AK. A case for regulatory B cells. J Immunol. 2006;176:705–710.
- Mauri C, Menon M. Human regulatory B cells in health and disease: therapeutic potential. J Clin Invest. 2017;127:772–779.
- He Y, Qian H, Liu Y, et al. The roles of regulatory B cells in cancer. J Immunol Res. 2014;2014:215471.
- Zhang Y, Gallastegui N, Rosenblatt JD. Regulatory B cells in anti-tumor immunity. Int ImmUNOL. 2015;27:521–530.
- Katz SI, Parker D, Turk JL. B-cell suppression of delayed hypersensitivity reactions. Nature. 1974;251:550–551.
- Wolf SD, Dittel BN, Hardardottir F, et al. Experimental Autoimmune Encephalomyelitis Induction in Genetically B Cell–deficient Mice. J Exp Med. 1996;184:2271–2278.
- Yang M, Sun L, Wang S, et al. Cutting edge: novel function of B cell-activating factor in the induction of IL-10–producing regulatory B cells. J Immunol. 2010;184:3321–3325.
- Peng B, Ming Y, Yang C. Regulatory B cells: the cutting edge of immune tolerance in kidney transplantation. Cell Death Dis. 2018;9:109.
- Wortel CM, Heidt S. Regulatory B cells: phenotype, function and role in transplantation. Transpl Immunol. 2017;41:1–9.
- Sarvaria A, Madrigal JA, Saudemont A. B cell regulation in cancer and anti-tumor immunity. Cell Mol Immunol. 2017;14:662–674.
- Schwartz M, Zhang Y, Rosenblatt JD. B cell regulation of the anti-tumor response and role in carcinogenesis. J Immunother Cancer. 2016;4:40
- Jin L, Weiqian C, Lihuan Y. Peripheral CD24hiCD27+CD19+B cells subset as a potential biomarker in naïve systemic lupus erythematosus. Int J Rheum Dis. 2013;16:698–708.
- Couper KN, Blount DG, Riley EM. IL-10: the master regulator of immunity to infection. J Immunol. 2008;180:5771–5777.
- Gallego-Valle J, Pérez-Fernández VA, Correa-Rocha R, et al. Generation of human Breg-like phenotype with regulatory function in vitro with bacteria-derived oligodeoxynucleotides. Int J Mol Sci. 2018;19:1737.
- Kawashima A, Tanigawa K, Akama T, et al. Innate immune activation and thyroid autoimmunity. J Clin Endocrinol Metab. 2011;96:3661–3367.
- Liu J, Fu J, Xu Y, et al. Antithyroid drug therapy for Graves' disease and implications for recurrence. Int J Endocrinol. 2017;2017:1.