Abstract
Rheumatoid arthritis (RA) displays very similar characteristics to those of tumor cells, platycodin D (PD) is a triterpenoid saponin abundant in Platycodon grandiflorum (PG), plays an important role in the inhibition of tumor growth. Our previous experiments confirmed that PD inhibited MH7A cell proliferation and migration, but it’s possible mechanism remain unclear. This study aimed to reveal the mechanism of PD on RA, based on network pharmacology analysis. Rat of CIA was treated with the different doses PD. The arthritis score and paw volume were evaluated, ankle imaging changes were observed via myosseous ultrasound, all rats were anaesthetized by intraperitoneal injection of 25% urethane (1 mL/100 g), and ankle histopathology was observed using hematoxylin and eosin (HE) staining. Cell (MH7A) Counting Kit 8 (CCK8) was used to measure cell activity, and JC-1 assay kit and flow cytometry were employed to examine the cell mitochondrial membrane potential and apoptosis. The expression levels of Sonic hedgehog (Shh) signaling pathway-related proteins were observed by Western blotting. Cell inflammation levels of tumor necrosis factor alpha (TNF-α) and interleukin (IL)-6 being determined via enzyme-linked immunoassay ELISA and q-PCR. In total, the saponin PD significantly improves joint synovium inflammation and apoptosis in CIA rats. The activity of administered MH7A was significantly inhibited, the mitochondrial membrane potential decreased, the expression level of the Shh signaling pathway-related protein SuFu increased, the expression levels of SHh and Gli decreased, and cell serum levels of TNF-a and IL-6 decreased significantly. Therefore, PD exhibits therapeutic potential for synovial hyperplasia in RA.
Introduction
Rheumatoid arthritis (RA) is a systemic, chronic, and inflammatory immune disease that is characterized by systemic multi-joint involvement [Citation1]. Its progression can cause joint deformity and may result in permanent disability, leading to a tremendous economic burden on patients and their families [Citation2]. The cause of RA has not yet been fully elucidated, and there is a lack of specific treatment options [Citation3]. Moreover, the primary pathology of joints in patients with RA involves synovitis associated with hyperplasia. This is a consequence of the numerous fibroblast-like synoviocytes (FLS) abundant in mesenchymal cells that are the structural cells that define organ architecture. Abnormally activated FLS (the major subtype of synovial cells), which are the primary producers of key components of synovial fluid and synovial stroma, dominate in RA and drive inflammation, possibly determining the course of the disease. FLS act as synovial mesenchymal cells as well as effector cells; they abnormally proliferate [Citation4] and are directly involved in pannus formation [Citation5]. Nevertheless, the mechanism of the activation of the RA-FLS transition requires further study, as do suitable treatment targets. Traditional Chinese Medicine has recently attracted significant research attention owing to its remarkable efficacy and few side effects [Citation6]. Platycodin D (PD) is one of the main active ingredients of Platycodon grandiflorum; it has many pharmacological effects, such as anti-inflammatory [Citation7] and anti-tumor properties [Citation8]. Moreover, PD can improve the symptoms of collagen-induced arthritis in DBA/1J mice and reduce the level of oxidative stress index myeloperoxidase (MPO) and lipid oxidation end product malondialdehyde (MDA) [Citation9]. A previous study by our research group confirmed that PD can inhibit the proliferation and invasion and promote the apoptosis of MH7A cells and induce growth arrest in the G0/G1 phase of these cells [Citation10]. However, its specific mechanism of action remains unclear, particularly in FLS. Therefore, in this study, we explored the potential targets and action pathways of PD using animal models and bioinformatics, with RA-FLS being used in an in vitro experimental verification model. Thus, the aim of this study was to improve current understanding regarding the selection of RA treatments.
Methods
Animal model and experimental design
Wistar male rats were adaptively fed for 7 days. Subsequently, fully dissolved bovine collagen in 0.05 mol/L acetic acid (concentration = 2 mg/mL) with a complete adjuvant in a 1:1 ratio was injected subcutaneously via the tail root of each animal to induce arthritis. The PD group was administered different doses of PD (50 or 150 mg/kg) and 1.8 mg/kg leflunomide (LEF) after initial collagen immunization. The clinical arthritis indexes in the collagen-induced arthritis (CIA) animal model were estimated as follows: 0: normal; 1: mild, apparent swelling limited to individual digits; 2: moderate, redness and swelling of the ankle; 3: redness and swelling of the paw including digits; and 4: maximally inflamed limb with involvement of multiple joints. The arthritis score for each mouse was the sum of all four paws, totaling between 0 and 16 points for each mouse. The swelling volume of the left hind paw of each rat was measured before the first immunization and then repeated on days 0, 7, 14, 21, and 28. The change in paw volume for each group was calculated as follows: the volume of hind paw swelling (ΔmL) = Vt – V0, where V0 is the volume before complete Freund’s adjuvant (CFA) immunization (mL) and Vt is the volume at t day after CFA immunization (mL) [Citation11]. After 4 weeks, all rats were anesthetized by intraperitoneal injection of 25% urethane (1 mL/100 g), following which blood, synovial, and ankle joint samples were collected (with rats in a fasted state). All experimental animals were euthanized depletory after anesthesia. The ankle joint was scanned with a B-ultrasound probe and the findings were interpreted by imaging practitioners. The synovial samples were stained with hematoxylin and eosin (HE).
Library and software
PubChem database (https://pubchem.ncbi.nlm.nih.gov/) [Citation12]; the Swiss Target Prediction Database (http://www.swisstargetprediction.ch/) [Citation13]; the GeneCards database (https://www.genecards.org/) [Citation14]; TCM Integrated Pharmacology Research Platform (TCMIP,http://www.tcmip.cn/TCMIP/ index.php/Home/) [Citation15]; the DAVID database (https://david.ncifcrf.gov/) [Citation16]; GeneMANIA (http://www.genemania.org/) [Citation17] were used.
Screening of components and pharmacological biotargets of PD
Composition of PD is input into PubChem database to collect its chemical structure; import each chemical structure into Swiss TargetPrediction database to predict the action target and obtain the action target.
Screening drug-disease biotargets of RA
With “Rheumatoid arthritis” as the keyword, relevant target information was retrieved in GeneCards and TCMIP databases, integrating two databases to retrieve results and remove duplicate values to obtain disease-related targets. Map Wayne was used to get a common target of the active drug ingredient with RA.
Common biotarget enrichment analysis
Typical targets were imported through the DAVID database. Path enrichment analysis was conducted through gene ontology (GO) and Kyoto encyclopedia of genes and genomes (KEGG), and the resultant data were visualized using Cytoscape software. The common targets were imported into the String database to obtain the protein–protein interaction (PPI) network diagram, which was visualized using Cytoscape software.
Quantitative reverse-transcriptase PCR
Total RNA was extracted using TRIzol reagent. The concentration was measured, and target gene primers were added. Subsequently, each sample was reverse-transcribed using the cDNA synthesis kit according to the manufacturer’s protocol. The thermocycling conditions were as follows: 42 °C for 2 min→ 37 °C for 15 min→ 85 °C for 5 s, 30 cycles, and storage at 4 °C. For endogenous controls, β-actin was used to normalize mRNA expressions. To examine relative gene expression, qPCR was conducted using a TB Green Premix Ex Tap PCR kit (Applied Takara, Code No. RR047A). Target gene expression was evaluated via the ΔΔCt method after reverse transcription and amplification.
CCK 8 and flow cytometry measurement
Depending on cell growth, the growth area was allowed to reach 80%–90% confluence for subsequent experiments. After vaccination with a cell density of cells per well in a 96-well plate, wells were prepared with different concentrations of PD (5, 10, 15, 20, 25, and 30 mg/L); 0.1% dimethyl sulfoxide (DMSO) was added into the medium for the control group. Subsequently, 10 μL of Cell Counting Kit 8 (CCK-8) reagent was added to each well, and the plate was placed in an incubator and cultivated for 1–2 h. Enzyme markers were then used to detect the light density value (OD) of each well at 450 nm. The cell suppression rate was calculated as 1 – A drug/A control × 100%. The rate of apoptosis was measured via flow cytometry. Using FL-1 and FL-2 channels and a cross gate blank to compensate for single-dye regulation, 3000 to 10,000 cells per cell population were collected per tube, and the detection data were analyzed using BD AccuriC6 Software.
Western blot assays
A cell suspension was routinely prepared and cultured at a cell density of 5 × 105 mL−1 and a volume of 1 mL per well. After cell adhesion, for the administration group, the treated cells were added at different concentrations (5, 10, and 15 µmol/L); GANT-61 was then added to the positive drug group (10 µmol/L). After being cultured for 4 h in 0.1% DMSO, cells were collected, and 200 µL of precooled radio immuno precipitation assay (RIPA) lysis buffer was added. The protein concentration was determined using the bicinchoninic acid (BCA) assay, following which 5× protein sample buffer was added at a ratio of 4:1. Electrophoresis was then conducted for 90 min, and samples were incubated overnight at 4 °C. The following day, the samples were placed into a chemiluminescence imaging system (Bio-Rad) for 90 min, and Image J software was used for protein strip analysis.
JC-1 assay kit and ELISA
The cells were treated using the “2.2” method and inoculated into 24-well culture plates with coverslips at a cell density of 10 × 105 cells/mL and volume of 500 L/well. PD (5, 10 and 15 mg/L) were then added after wall attachment. For the positive drug group, medium containing GANT-61 (10 µmo1/L) was added, and samples were left for 24 h. Changes in the mitochondrial membrane potential and apoptosis were observed using a microplate imaging system according to the manufacturer’s instructions of mitochondrial membrane potential (JC-1) assay kit. The superior inflammatory level of RA primary and MH7A cells was detected via the ELISA method strictly following the manufacturer’s instructions for determining IL-6 and TNF-α. Finally, the OD of each sample was calculated for statistical analysis.
Statistical analysis
Statistical analysis using GraphPad Prism8.0 (Graphpad Software, Inc) software, the measurement data by mean ± standard deviation (x ± s), when meeting the normal distribution, do not meet the normal distribution using the Man–Whitney test. All experiments underwent single factor variance analysis (one-way ANOVA).
Results
Arthritis score and paw volume
The flowchart shows all essential procedures in our study (). Compared with those in the normal group, the paw volume was significantly increased (p < 0.05) and the arthritis score and paw volume were significantly reduced in the PD group (p < 0.05) ().
Figure 2. Arthritis score and paw volume. A–E: Normal; Model; PD (5 mg/kg); PD (15 mg/kg); LEF (1.8 mg/kg) treatments. F and G: the effects of each group at arthritis score and paw volume.
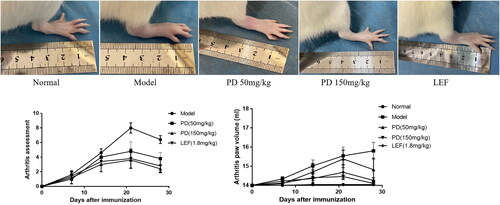
Figure 3. The effects of each group treatments on rat (A) iconography of synovial thickness, (B) change of histopathology, (C) the ankle was evaluated in each group using a scoring system including cellular infiltration, synovial proliferation, cartilage erosion, and pannus formation. Data are expressed as the mean ± S.D, n = 3,*p<0.05, **p<0.01 vs. model.
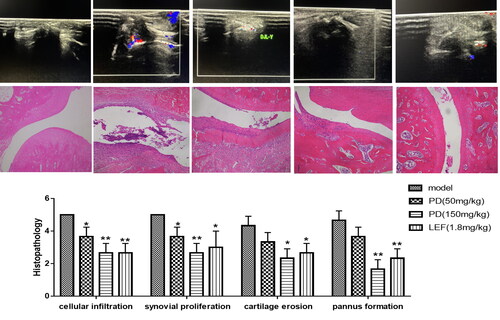
HE staining and iconography
Musculoskeletal ultrasound revealed that the ankle synovial thickness of healthy rats was normal with no blood flow signal. Model rats had thickened ankles that were not smooth and serrated, with dot and bar blood flow signals. Furthermore, less smooth thickening and punctate blood flow signal were observed in low-dose PD rats than in the high-dose group. The slight synovial thickening in the high-dose PD group showed no blood flow signal (). The pathological changes in the ankle joints were evaluated in each group using a scoring system by inflammatory cell infiltration, synovial proliferation, cartilage erosion, pannus formation, and the results showed the PD (50 mg/kg) group had a slight improvement of cell infiltration and synovial proliferation, whereas the PD (150 mg/kg) groups and the LEF (1.8 mg/kg) group showed the significantly enhanced therapeutic effect ( and ).
Network pharmacology analysis
Drug anti-RA target prediction of 20 drug major component-related action targets were obtained by the Swiss Target Prediction database, and 2855 disease-related action targets were obtained by GeneCards and TCMIP and obtained 9 drug-disease common targets (). Based on the GeneMANIA database, we analyzed the co-expression network and related pathways of these genes. These genes were mainly involved in regulating mitochondrial apoptosis, RNA polymerase II, and mitochondrial membrane permeability during apoptosis. Co-expression was 34.39%, physical interaction was 22.26%, co-localization was 7.38%, genetic interaction was 23.47%, prediction was 11.62%, and pathway was 0.87% (). The GO and KEGG pathway enrichment analysis results were obtained through the DAVID database, including RNA polymerase II promoter, cell proliferation and transcription (), with 37 pathways enriched, target-pathway, and the drugs mainly treated RA by participating in signaling pathways such as apoptosis, Hedgehog, p53, and EGFR ().
Effect of PD on RA-FLS function
The effect of plasticine D on the proliferation and activity of RA-FLS cells was studied. With increased cytosine D concentration compared with control (0 mg/L), half of the inhibition concentration in RA-FLS cells was 8.892 mg/L (p <0.01) and 7.696 mg/L (p<0.01)(). Compared with the control group (0 mg/L), after 24 h of MH7A cells treated with PD (5 mg/L, 10 mg/L, 15 mg/L) the apoptosis rate was 8.03%, 0.09%, 35.95%, 1.08% (p < 0.01), respectively (p < 0.01). This result indicated that PD can induce apoptosis in MH7A cells ().
Figure 5. A: The rate of 24-h inhibition by PD (EC50 = 0.949); (B) the rate of 48-h inhibition by PD (EC50 = 0.8863); (C) flow cytometric analysis diagram, apoptosis is divided into four quadrants: Q1-UL (necrotic cells and debris), Q1-UR (late apoptotic cells), Q1-LL (normal cells), Q1-LR (early apoptotic cells); the sum of Q1-UR and Q1-LR; FL1-A: Annein-V staining, FL2-A: PI staining and effect of platycodin D was measured by flow cytometry on apoptosis of MH7A cells.
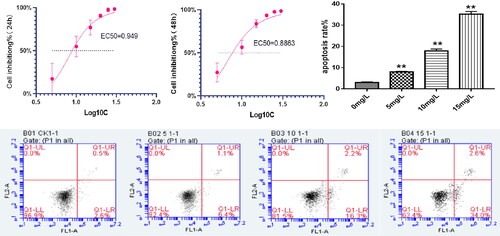
Table 1. Effect of platycodin D on proliferation of MH7A cells (x ± s, n = 5).
Validation of the effect of PD on the RA-FLS mechanism
PD significantly reduced SHh, G1i1, and BCL-2 protein expression (p < 0.05) and substantially increased SuFu expression (p < 0.05) (). JC-1 staining revealed that both PD (15 mg/mL) and GANT-1 significantly promoted apoptosis, red fluorescence was diminished, and green fluorescence was enhanced, indicating that PD can inhibit the activation of the Hedgehog signaling pathway and apoptosis (). The expression of inflammatory factors in RA-FLS cells in each group was examined by ELISA and QPCR. PD significantly decreased the expression of TNF-α compared with that of the control group (p < 0.05) (), and the concentration of cellular IL-6 expression was significantly reduced (p < 0.05) (). These findings indicate that PD inhibits the RA-FLS inflammatory response.
Figure 6. A: The expression levels of SHH, Gli, SuFu, and Bcl-2 in different groups (means ± SD, n = 3, p < 0.05), as compared with the control group; (B) changes in mitochondrial membrane potential in cells from different groups; (C and D) the Effects of PD on changes in TNF-a and IL-6 inflammatory factors of RA-FLS.
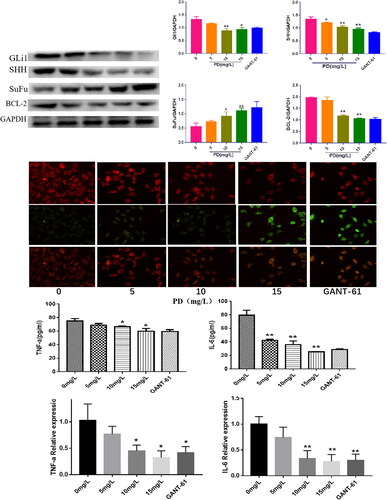
Discussion
In RA, synovial fibroblasts contribute to joint damage by stimulating both pro-inflammatory and tissue destructive pathways. Currently, various conventional anti-rheumatic drugs are available for the treatment of RA [Citation18]. Nonetheless, disease-modifying anti-rheumatic drugs and biologicals do not address all the clinical issues, including efficacy and toxicity [Citation19]. Therefore, seeking safer and more effective preparations from traditional Chinese medicines is important. The major chemical components of P. grandiflorum include saponins, flavonoids, and polysaccharides. Among them, the saponin PD is a pentacyclic triterpenoid saponin monomer [Citation20]. PD can inhibit nitric oxide production and inflammation. Its anticancer activity has also been extensively studied; however, its mechanism in relation to RA remains unclear. In this study, we assessed the therapeutic effects of PD in rats with CIA, which is a well-established in vivo model that reflects the common immunological and pathological features of RA. The paw volume and arthritis global assessment were used as the indicators of disease progression. Our results demonstrate that PD improved joint inflammation and synovial hyperplasia in rats with CIA. Histopathologic and ultrasound examinations of the joints further demonstrated the protective and anti-inflammatory effects of PD via reducing inflammatory cell infiltration and synovial hyperplasia, as well as joint cartilage and bone erosion. PD could be a promising anti-rheumatic drug as it has been shown to exert anti-inflammatory, antioxidative, and immunomodulatory effects in DBA/1J mice [Citation9]
Because RA-FLS are similar to tumor cells, we demonstrated that PD had a significant cytotoxic effect through cell growth and migration-induced apoptosis within a specific dose range. They are linked to vital processes in RA such as chronic synovitis and joint destruction. Therefore, many studies have investigated the application of apoptosis in the treatment of RA, as this could have great significance for future targeted therapy. Perlman et al. revealed that Bcl-2 is more expressed in FLS of RA than in synovial tissue of osteoarthritis. Moreover, they found that knocking out the Bcl-2 gene in relation to RA led to a reduction in Bcl-2 expression in vitro [Citation21]. Decreased cell viability in FLS has been shown to alter mitochondrial permeability and induce the release of Cyt c, resulting in the activation of Caspase-9 and Caspase-3, as well as DNA fractures.
Based on these findings, the present study used network pharmacology to study the potential mechanism of action of PD in the treatment of RA. The disease targets of PD as an RA drug included BCL2L1, F2, GLI1, Jun, and STAT3. In particular, BCL2L1 has been identified as an important apoptotic member [Citation22].The GLI1 gene is localized in humans as one member of the encoding zinc finger protein family; it activates Gli to initiate Bcl-2 expression [Citation23]. The c-Jun terminal kinase (JNK) is an important contributor in the pathology of RA because its phosphorylation initiates the expression of matrix metalloproteinases (MMPs) to promote bone destruction. Transcriptional activation factor 3 (STAT3) is involved in cell proliferation and differentiation under JAK signaling [Citation24]. Based on this, several of the major biological functions enriched by these disease targets were identified using the KEGG signaling pathway and co-expression network. In detail, these were p53, Shh, EGFR, the regulation of mitochondrial membrane permeability, apoptosis, intracellular receptor signaling, and other biological processes. These findings show that PD can treat RA through multi-target and multi-pathway cooperation. Mechanistically, this may have occurred by inhibiting the activation of the Shh signaling pathway. RA-FLS exist in a synchronic inflammatory microenvironment [Citation25]. The Shh signaling pathway is conservative. When signaling is activated, Shh binds to Ptch1, thereby inhibiting Smo by releasing the activated form of Gli-A. In this way, it participates in target gene transcription and expression [Citation26]. This in turn results in the activation of Caspase-9 and Caspase-3, causing DNA fractures [Citation27]. Furthermore, the Shh signaling pathway is known to be involved in the circumstances, development, and metastasis of multiple tumors [Citation28]. Activated Shh signals and the increased proliferation and migration of FLS have been reported in synovial tissue obtained from patients with RA [Citation29]. The aggressiveness of overexpressed Shh on cartilage during the inhibition of GLI1 can significantly reduce FLS proliferation [Citation30]. Moreover, the activation of Shh signaling promotes the expression of pro-inflammatory cytokines, such as IL-6 and TNF-α, in FLS. This behavior is similar to the intervention effect of GANT-61 [Citation31], which is a molecular inhibitor of Shh signaling. This suggests that the activation of Shh signaling occurs in patients with RA, and that Shh signaling is involved in the activation of RA-FLS. In this study, the results of our in vivo experiments have shown that PD was effective in alleviating disease progression in rats with CIA, including regulating apoptosis of FLS and reducing the production of pro-inflammatory factors (TNF-α and IL-6). Our findings further suggest that PD might regulate mitochondrial apoptosis through the Hedgehog signaling pathway. Further studies should explore the mechanisms of cellular types of apoptosis associated with PD in vitro.
Conclusion
In summary, apoptosis is one of the most effective therapeutic targets for PD, a saponin that can be isolated from P. grandiflorum, for the treatment of RA. This therapeutic effect functions through the Shh signaling pathway, as confirmed herein by experiments performed with RA-FLS. Further studies are needed to reveal the multiple pathways for a range of predicted targets. It is expected that such results could provide a theoretical basis and reveal a therapeutic strategy for targeted RA-FLS therapy.
Ethics approval and consent to participate
The studies involving animal participants were reviewed and approved by animal Ethical Committee of The Affiliated Hospital of Guizhou Medical University [No: 2101153] .
Disclosure statement
No potential conflict of interest was reported by the authors.
Data availability statement
All data generated or analyzed during this study are included in this published article.
Additional information
Funding
References
- Aletaha D, Smolen JS. Diagnosis and management of rheumatoid arthritis: a review. JAMA. 2018;320(13):1–9.
- Onuora S. Rheumatoid arthritis: higher disease risk in children of women with RA. Nat Rev Rheumatol. 2018;14(2):62.
- Zhou L, WangLi T, Chen Y, et al. 1,25-Dihydroxyvitamin D3 ameliorates collagen-induced arthritis via suppression of Th17 cells through miR-124 mediated inhibition of IL-6 signaling. Front Immunol. 2019;10:178.
- Komatsu N, Takayanagi H. Mechanisms of joint destruction in rheumatoid arthritis – immune cell-fibroblast-bone interactions. Nat Rev Rheumatol. 2022;18(7):415–429.
- Lin J, Sun AR, Li J, et al. A three-dimensional co-culture model for rheumatoid arthritis pannus tissue. Front Bioeng Biotechnol. 2021;9:764212.
- Zhang Q, Liu J, Zhang M, et al. Apoptosis induction of fibroblast-like synoviocytes is an important molecular-mechanism for herbal medicine along with its active components in treating rheumatoid arthritis. Biomolecules. 2019;9(12):795.
- Ji MY, Bo A, Yang M, et al. The pharmacological effects and health benefits of Platycodon grandiflorus – a medicine food homology species. Foods. 2020;9(2):142.
- Lee SJ, Choi Y-J, Kim HI, et al. Platycodin D inhibits autophagy and increases glioblastoma cell death via LDLR upregulation. Mol Oncol. 2022;16(1):250–268.
- Kwon OG, Ku SK, An HD, et al. The effects of platycodin D, a saponin purified from platycodi radix, on collagen-induced DBA/1J mouse rheumatoid arthritis. Evid Based Complement Alternat Med. 2014;2014:954508.
- Wang J, Zeng JS. Effects and mechanism of platycodin D on proliferation and apoptosis of MH7A cells. J Guizhou Medical Univ. 2020;45(10):1149–1155.
- Zhou J, Yu Y, Yang X, et al. Berberine attenuates arthritis in adjuvant-induced arthritic rats associated with regulating polarization of macrophages through AMPK/NF-кB pathway. Eur J Pharmacol. 2019;852(2019):179–188.
- Wang Y, Xiao J, Suzek TO, et al. PubChem’s BioAssay database. Nucleic Acids Res. 2012;40(Database issue):D400–D12.
- Daina A, Michielin O, Zoete V. SwissTargetPrediction: updated data and new features for efficient prediction of protein targets of small molecules. Nucleic Acids Res. 2019;47(W1):W357–W364.
- Rappaport N, Fishilevich S, Nudel R, et al. Rational confederation of genes and diseases: NGS interpretation via GeneCards, MalaCards and VarElect. Biomed Eng Online. 2017;16(Suppl 1):72.
- Wan Y, Xu L, Liu Z, et al. Utilising network pharmacology to explore the underlying mechanism of wumei pill in treating pancreatic neoplasms. BMC Complement Altern. 2019;19:158.
- Jiao X, Sherman BT, Stephens R, et al. DAVID-WS: a stateful web service to facilitate gene/protein list analysis. Bioinformatics. 2012;28(13):1805–1806.
- Warde-Farley D, Spranger S, Lorenz-Depiereux B, et al. The GeneMANIA prediction server: biological network integration for gene prioritization and predicting gene function. Nucleic Acids Res. 2010;1:38–45.
- Woude D, Ml AH. Update on the epidemiology, risk factors, and disease outcomes of rheumatoid arthritis. Best Pract Res Clin Rheumatol. 2018;32(2):174–187.
- Ulku U, Betul C, Talip YD, et al. Drug repurposing for rheumatoid arthritis: identification of new drug candidates via bioinformatics and text mining analysis. Autoimmunity. 2022;55(3):147–156.
- Li Q, Yang T, Zhao S, et al. Distribution, biotransformation, pharmacological effects, metabolic mechanism and safety evaluation of platycodin D: a comprehensive review. Curr Drug Metab. 2022;23(1):21–29.
- Yang J, Zhao SL, Yang XL, et al. Inhibition of B-cell apoptosis is mediated through increased expression of bcl-2 in patients with rheumatoid arthritis. Int J Rheum Dis. 2016;19(2):134–140.
- Avi A, Wayne JF, Joel DL, et al. From basic apoptosis discoveries to advanced selective BCL-2 family inhibitors. Nat Rev Drug Discov. 2017;16(4):273–284.
- Doheny D, Manore SG, Wong GL, et al. Hedgehog signaling and truncated GLI1 in cancer. Cells. 2020;9(9):2114.
- Zhu SL, Ye YM, Shi YM, et al. Sonic hedgehog regulates proliferation, migration and invasion of synoviocytes in rheumatoid arthritis via JNK signaling. Front Immunol. 2020;11(11):1300.
- De Cauwer A, Mariotte A, Sibilia J, et al. DICER1: a key player in rheumatoid arthritis, at the crossroads of cellular stress, innate immunity, and chronic inflammation in aging. Front Immunol. 2018;9:1647.
- Hu Z, Chen Y, Zhu S, et al. Sonic hedgehog promotes proliferation and migration of fibroblast-like synoviocytes in rheumatoid arthritis via rho/ROCK signaling. J Immunol Res. 2022;2022:3423692.
- Palle K, Mani C, Tripathi K, et al. Aberrant GLI1 activation in DNA damage response, carcinogenesis and chemoresistance. Cancers (Basel). 2015;7(4):2330–2351.
- Ding J, Li HY, Zhang L, et al. Hedgehog signaling, a critical pathway governing the development and progression of hepatocellular carcinoma. Cells. 2021;10(1):123. 11
- Zhu SL, Huang JL, Peng WX, et al. Inhibition of smoothened decreases proliferation of synoviocytes in rheumatoid arthritis. Cell Mol Immunol. 2017;14(2):214–222.
- Nishimura R, Hata K, Takahata Y, et al. Role of signal transduction pathways and transcription factors in cartilage and joint diseases. Int J Mol Sci. 2020;21(4):1340.
- Qin S, Sun D, Li X, et al. GANT61 alleviates arthritic symptoms by targeting fibroblast-like synoviocytes in CIA rats. J Orthop Sci. 2019;24(2):353–360.