Abstract
Rheumatoid arthritis (RA) is a common type of chronic inflammatory disease. Elucidating the mechanism of fibroblast-like synovial (FLS) as a pathologic factor in RA may address the urgent medical requirement for the treatment of RA. Isorhynchophylline (IRN) is a tetracyclic hydroxyindole alkaloid isolated from uncinaria, which has multiple biological activities and affects the progression of osteoarthritis. However, the role of IRN in rheumatoid arthritis remains unclear. Herein, our study aimed to elucidate the potential effect of IRN on RA and reveal its mechanism. Human FLS cell line MH7A cells were stimulated with TNF-α for 24 h to construct a cell model. CCK-8, Edu, wound healing, as well as transwell assays were conducted to detect the effects of IRN on cell proliferation and motility. ELISA and Immunoblot assays were further performed to detect the production of pro-inflammatory factors and the expression levels of MMPs. Immunoblot and Immunostaining assays were conducted to uncover the mechanism. ELISA, H&E staining, and Immunoblot assays were used to confirm the effects of IRN on RA in a CIA rat model. We revealed that IRN restrained TNF-α-stimulated MH7A cell proliferation and motility. In addition, IRN blocked the production of pro-inflammatory factors and MMPs in TNF-α-stimulated-MH7A cells. We further found that IRN restrained FOXC1/β-catenin axis, and improved MH7A cell proliferation as well as migration via the FOXC1/β-catenin axis. IRN restores CIA by inhibiting pro-inflammatory cytokines in synovial tissues. In summary, IRN attenuates proliferation and migration of FLS in RA via the FOXC1 mediated β-catenin axis.
Introduction
Rheumatoid arthritis (RA) is a common chronic inflammatory disease featured by persistent synovial hyperplasia [Citation1]. The main pathologic feature of confirmed RA is the proliferative tissue, which contains a large number of activated fibroblast-like synovial (FLS) cells (FLS) [Citation2]. FLS is crucial in the pathogenesis of the disease through interactions with T cells, B cells, macrophages, as well as multiple pro-inflammatory cytokines [Citation3]. As a major source of inflammatory cytokines and catabolic enzymes that promote joint degradation, RA-FLSs also exhibit several tumor-like behaviours when activated in a chronic inflammatory setting [Citation4]. This shifting behaviour stimulates RA progression and leads to joint destruction [Citation5]. Therefore, elucidating the underlying mechanism of FLS as a pathologic factor in RA may address the urgent medical requirement for RA treatment.
Isorhynchophylline (IRN) is a tetracyclic hydroxyindole alkaloid isolated from uncinaria [Citation6]. Isorhynchophylline (IRP) can regulate lipid metabolism [Citation6,Citation7]. IRN mitigated paraquat-induced acute kidney injury, oxidative stress, and inflammatory damage in LPS-induced macrophages [Citation7]. IRN inhibits inflammatory responses in endothelial cells and macrophages through the NF-κB/NLRP3 signalling pathway [Citation6]. Isorhynchophylline alleviates cartilage degeneration in osteoarthritis by activating autophagy of chondrocytes [Citation8]. Isorhynchophylline improves the progression of osteoarthritis by inhibiting the NF-κB signalling pathway [Citation8]. The administration of IRN inhibits the activation of FOXC1/NF-κB axis. However, the role of IRN in rheumatoid arthritis remains unclear.
Forkhead box c1 (FOXC1) is a key member of the Fox transcription factor family and is crucial in multiple biological processes, including metabolism, differentiation, proliferation, apoptosis, migration, invasion, and longevity [Citation9]. Studies have shown that FOXC1 expression is higher in fibroblast-like synovial cells (FLS) of RA, and FOXC1 promotes cell proliferation by upregulating inflammation-dependent PI3K/AKT axis, providing new therapeutic benefits for the treatment of RA patients [Citation10]. FOXC1 has been reported to promote gastric cancer metastasis by activating the Wnt/β-catenin axis [Citation11]. The Wnt/β-catenin axis is known to be activated in the synovium of RA patients and experimental RA animals, as well as in cultured FLS [Citation12]. Increasing evidence show that the activation of Wnt/β-catenin pathway is involved in the pathogenesis of RA, and is related to the regulation of proliferation and motility of RA FLS, and suppression of this axis might be an effective strategy for RA treatment [Citation13,Citation14].
Here, we aimed to elucidate the potential effect of IRN on RA and reveal its mechanism. Our results suggested that IRN attenuated proliferation and migration of synovial fibroblasts in RA.
Materials and methods
Cell culture and treatment
Human MH7A cells were obtained from Jennio Biological Technology (Guangzhou, China) and maintained with DMEM supplied with 10% FBS at a sterile hood with 37 °C, 5% CO2. The cultured cells were treated with 10 ng/mL TNF-α for 24 h. IRN was used to treat cells at the dose of 10, 20 and 40 μM. For cell transfection, cells at 80% confluency were transfected with control or FOXC1 vectors with Lipofectamine 2000 (Invitrogen, USA).
CCK-8 assay
103 cells per well of MH7A cells were seeded into 96-well plates, and incubated as indicated in the figure legend. Then cells were maintained for 2 days and subsequently were incubated with CCK-8 solution for 4 h. Then the absorbance at 450 nm was measured by a multifunction measuring instrument (SpectraMax M5, Molecular Devices, USA).
EdU staining
The growth of MH7A cells was determined by EdU staining. Cells were treated as indicated. Then, cells were incubated with 50 μM EdU for 2 h. Afterwards, cells were fixed with 4% PFA and treated with 2 mg/ml glycine for 5 min. Cell were permeabilized and treated with Apollo reaction for 30 min and stained with Hoechst. The images were captured. The number of EdU-positive cells were counted per field, and 8 fields were used for the analysis of percentage of Edu-positive cells.
Transwell assay
Cells were plated into the upper chamber of transwell with no serum cell culturing medium. Subsequently, the bottom chamber was filled with complete medium to stimulate motility. After culturing for 24 h, cell medium in upper chamber was discarded as well as the cells. The remaining cells were fixed, and stained with the 0.2% crystal violet. The number of cells were counted per field, and 8 fields were used for the analysis of invasion difference.
Wound-healing assay
Scratch was made with a 10-µl pipette tip. Afterwards, MH7A cells were washed twice to remove debris. The wound closure was measured at 0 and 24 h to evaluate the capability of wound closure.
ELISA
The level of IL-1β, TNF-α, IL-8, MMP-2/9 as well as IL-6 in the culturing supernatant and tissues were assessed by ELISA kit in agree with the suggestions of manufacturer. Briefly, samples were added. Then, primary antibodies were aspirated and subsequently the Horseradish Peroxidase (HRP) was added into wells after washing for several times. Then enzyme substrate was used for colour development. The intensity of each well was evaluated by a multifunction measuring instrument (SpectraMax M5, Molecular Devices, USA).
Western blotting
MH7A cells were lysed for protein extraction buffer. The extracted proteins were quantitated and then separated by SDS-PAGE, and further transferred to PVDF membrane. The membranes were blocked with 5% BSA for 1 h. Then the membranes were incubated with primary antibodies at 4 °C overnight. Primary antibodies targeting IL- 1β (Abcam, ab216995, 1:1000), IL-6 (Abcam, ab233706, 1:500), IL-8 (Abcam, ab235584, 1:1000), MMP-2 (Abcam, ab92536, 1:500), MMP-9 (Abcam, ab76003, 1:1000), FOXC1 (Abcam, ab227977, 1:1000), β-catenin (Abcam, ab32572, 1:1000), and β-actin (Abcam, ab8226, 1:3000) were used, and then secondary antibodies were added to the membranes for 1 h and blots were imaged after chemiluminescence and the expression was measured by Image J 9.0 (NIH, USA).
Immunofluorescent staining
MH7A cells were fixed with 4% PFA and then blocked with 5% BSA in PBST. And then cells were incubated with primary antibody targeting β-catenin (1:300, Abcam). After washing in PBST for three times, cells were incubated with secondary antibodies (Invitrogen, CA). Cell nuclei was labelled with DAPI. Images were acquired with a fluorescent microscope.
Rat CIA model construction and evaluation
Male SD rats (n = 6 in each group) were obtained from the SLAC company (Shanghai, China). The rats were maintained under sterile conditions. The animal experiments was approved by Ethical Committee on Experimental Animals of Nanjing Medical University. First, CCII was dissolved in 0.01 M acetic acid. Then the solution was injected into the left hind paws of rats at the first day. The boost injection was made in the tails at the 7th day. Control rats received equal amount of saline. Rast with no joint swelling at 12th day were discarded. Rats with signs of CIA were treated with or without IRN at varying dose from day 15 to 32.
HE staining
Freshly excised lung tissues were fixed and cut into 5 μm sections. Then the sliced sections were deparaffinized, and stained with haematoxylin as well as eosin (Sigma-Aldrich).
Statistics
GraphPad software was used and performed for statistical analysis. Data were represented as mean ± SD. The student’s t test was performed between two groups. The one-way ANOVA followed by Tukey’s post hoc test was performed among multiple groups. And the p < 0.05 was considered a significant difference.
Results
IRN inhibits MH7A cell proliferation induced by TNF-α
To evaluate the effects of IRN on MH7A cell proliferation, we stimulated MH7A cells with different doses of IRN (0, 10, 20, 40 as well as 80 μM) for 24 h and detected cell proliferation via CCK-8 assay. The chemical structure formula of IRN is shown in . We noticed that IRN treatment had no obvious impact on cell proliferation at low dose (10, 20, 40 μM, ). MH7A cell proliferation was significantly enhanced by TNF-α, and inhibited by IRN treatment at 40 μM, as detected by EdU staining (). Therefore, IRN treatment suppressed MH7A cell proliferation.
Figure 1. IRN inhibits MH7A cell proliferation induced by TNF-α. a. The chemical structure of IRN. b. The effect of IRN on cell viability of MH7A cell by CCK8 assay. c. The proliferation of MH7A cells induced by TNF-α with or without IRN. #p < 0.05, ##p < 0.01, ###p < 0.01 vs TNF-α group. *p < 0.05, **p < 0.01, ***p < 0.001 vs control group.
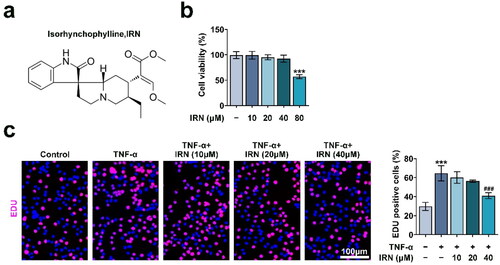
IRN blocks TNF-α-induced migration and invasion in MH7A cells
Cell migration and invasion were detected by wound-healing and transwell assay. As shown in , TNF-α-induced significant cell invasion, which was abrogated by IRN treatment. Similarly, the transwell assay showed that TNF-α-induced obvious enhanced migration, which was weakened by IRN treatment (). Therefore, IRN treatment significantly restrained the TNF-α-induced motility in MH7A cells.
Figure 2. IRN blocks TNF-α-induced migration and invasion in MH7A cells. a. Transwell assay detected the cell invasion of MH7A cells induced by TNF-α with or without IRN. b. The ability of cell migration in MH7A cells induced by TNF-α with or without IRN. #p < 0.05, ##p < 0.01, ###p < 0.01 vs TNF-α group. *p < 0.05, **p < 0.01, ***p < 0.001 vs control group.
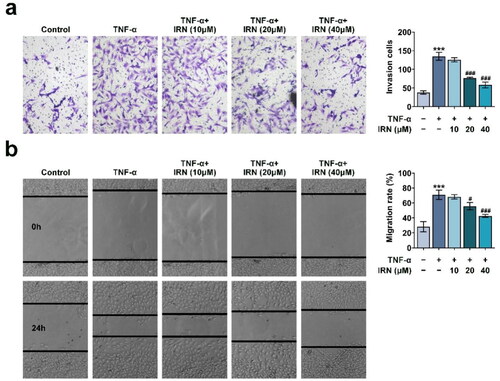
IRN inhibits Proinflammatory Cytokines and MMPs in TNF-α-induced MH7A cells
The release of pro-inflammatory cytokines and the levels of MMPs in MH7A cells were detected by ELISA and Immunoblot, respectively. We performed the ELISA and found that IRN treatment (40 uM) had modest effects on the secretion of IL- 1β, IL-6, IL-8, MMP-2, MMP-9, suggesting that IRN treatment alone had no effects on the inflammation of cells (Figure S1). However, the levels of IL-1β, IL-6, IL-8, MMP-2, and MMP-9 were enhanced in the TNF-α-induced cells. IRN treatment significantly restricted enhancement of these cytokines and MMPs (). Consistently, the protein levels of these cytokines and MMPs underwent the similar alterations as detected in the ELISA assay (). Collectively, IRN inhibits Proinflammatory Cytokines and MMPs in TNF-α-treated MH7A cells.
Figure 3. IRN inhibits Proinflammatory Cytokines and MMPs in TNF-α-induced MH7A cells. a. IRN reduced pro-inflammatory cytokines and MMPs in MH7A cell as detected by ELISA. b. The levels of pro-inflammatory cytokines and MMPs in MH7A cell as detected by western blot. #p < 0.05, ##p < 0.01, ###p < 0.01 vs TNF-α group. *p < 0.05, **p < 0.01, ***p < 0.001 vs control group.
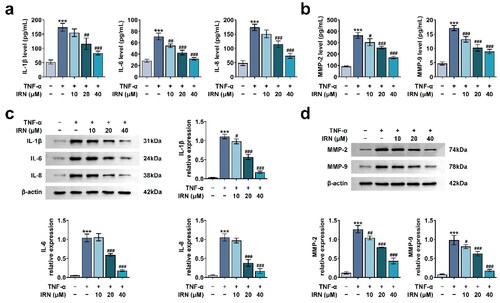
IRN inhibits FOXC1/β-catenin pathway in MH7A cells
To further examine the potential mechanism in IRN mediated cell proliferation and migration in MH7A cells, the FOXC1/β-Catenin was detected in TNF-α-treated MH7A cells. TNF-α stimulation promoted the levels of both FOXC1 and β-catenin, which were partially inhibited by IRN (). Moreover, the cellular localisation of β-catenin was investigated in TNF-α-treated MH7A cells and TNF-α-induced MH7A cells treated with IRN. TNF-α treatment induced significant nuclear translocation of β-catenin, which was abrogated by IRN treatment (). Therefore, IRN inhibits FOXC1/β-Catenin axis in MH7A cells.
Figure 4. IRN inhibits FOXC1/β-catenin pathway in MH7A cells. a. The levels of FOXC1 and β-catenin in MH7A cell treated as labelled as detected by western blot. b. The cellular location of β-catenin in MH7A cell induced by TNF-α with or without IRN. #p < 0.05, ##p < 0.01, ###p < 0.01 vs TNF-α group. *p < 0.05, **p < 0.01, ***p < 0.001 vs control group.
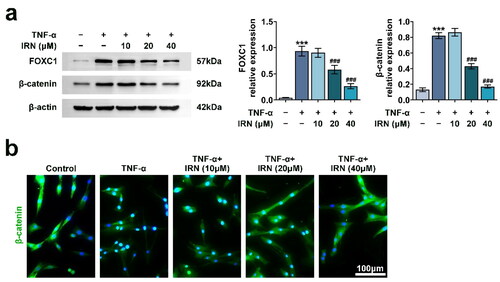
IRN inhibits cell proliferation as well as migration by restraining FOXC1/β-Catenin axis in MH7A cells
To verify the involvement of FOXC1/β-Catenin axis in IRN mediated cell proliferation and migration, FOXC1 vector was transfected into MH7A cells. IRNA significantly inhibited cell proliferation, and FOXC1 transfection attenuated the effect of IRN on proliferation (). In addition, the effect of IRN on the motility were reversed by FOXC1 transfection, suggesting the involvement of FOXC1/β-Catenin axis in IRN mediated cell proliferation and migration (). These results suggested that IRN inhibits cell proliferation and motility by restraining FOXC1/β-Catenin axis in MH7A cells.
Figure 5. IRN inhibits cell proliferation and migration by restraining FOXC1/β-catenin pathway in MH7A cells. a. The proliferation of MH7A cells induced by TNF-α and IRN with or without FOXC1 transfection. b. Transwell assay detected the cell invasion of MH7A cells induced by TNF-α and IRN with or without FOXC1 transfection. c. The ability of cell migration in MH7A cells induced by TNF-α and IRN with or without FOXC1 transfection. #p < 0.05, ##p < 0.01, ###p < 0.01 vs TNF-α group. *p < 0.05, **p < 0.01, ***p < 0.001 vs control group.
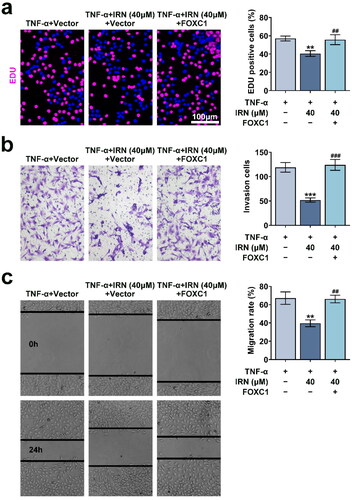
IRN restores CIA by inhibiting pro-inflammatory cytokines in synovial tissues
The therapeutic effects of IRN in CIA was further detected in vivo. CIA rats showed obvious clinical features like human RA, including synovial pannus formation, hyperplasia, as well as cartilage damage. IRN treatment significantly relieved these alterations in CIA rat ankle (). Additionally, ELISA results showed that CIA induced levels of TNF-α, IL-1β, IL-6, IL-8, MMP-2, and MMP-9, which were diminished by IRN treatment in a dose-dependent manner (). Immunoblot assays further confirmed that IRN restores CIA by inhibiting pro-inflammatory cytokines in synovial tissues via the FOXC1/beta-catenin axis (). These findings suggested that IRN restores rat CIA by suppressing the generation of inflammatory cytokines and MMPs in synovial tissues.
Figure 6. IRN restores CIA by inhibiting pro-inflammatory cytokines in synovial tissues. a. Representive images of HE staining in each group. b. The levels of proinflammatory cytokines (TNF-α, IL-1β, IL-6, IL-8) in various groups, detected by ELISA. c. The levels of MMPs (MMP-2, MMP-9) in various groups, detected by ELISA. d. The expression of FOXC1 and beta-catenin was detected in various groups via Immunoblot. #p < 0.05, ##p < 0.01, ###p < 0.01 vs TNF-α group. *p < 0.05, **p < 0.01, ***p < 0.001 vs control group.
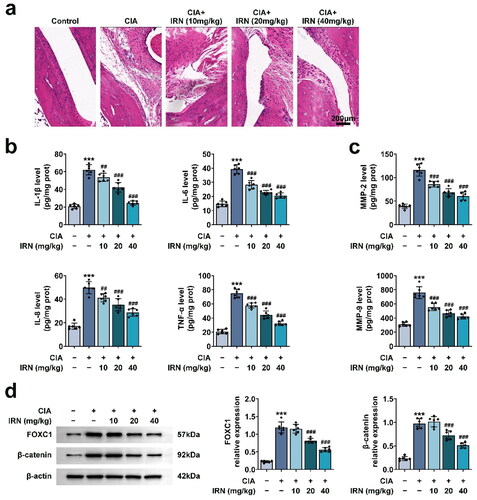
Discussion
Rheumatoid arthritis (RA) is a chronic disease of unknown aetiology [Citation15]. It is characterised by multi-joint, symmetrical and invasive joint inflammation of the small joints of the hands and feet, which can lead to joint deformity and loss of function, seriously jeopardising human health [Citation16]. FLS are the main effectors of proliferation and inflammation in synovial tissue of RA. They have biological features of abnormal proliferation, motility, adhesion, invasion as well as secretion, and play an important role in the pathology of RA. Therefore, inhibition of synovial cell dysfunction can effectively control the synovial inflammation and improve the symptoms of RA. In this study, human FLS cell line MH7A cells were stimulated with TNF-α for 24 h to construct a cell model. IRN inhibits cell proliferation and migration of TNF-α-induced MH7A cells, suggesting that IRN has the potential to serve as a drug of RA.
IRN is extracted from Uncaria rhynchophylla [Citation6]. IRN suppressed the inflammatory responses in endothelial cells and macrophages via mediating NF-κB/NLRP3 pathway [Citation17]. IRN could alleviate cartilage degeneration in osteoarthritis via stimulating autophagy of chondrocytes. IRN ameliorated stress-stimulated emotional disorder and cognitive impairment by modulation of NMDA receptors [Citation18]. In addition, it could mediate the circadian rhythm of the hypothalamus in spontaneously hypertensive animal models [Citation9]. Our data confirmed that IRN suppressed the TNF-α-induced MH7A cell proliferation, motility, and production of pro-inflammatory factors and MMPs. IRN improves the progression of osteoarthritis by inhibiting the NF-κB pathway and the activation of FOXC1/NF-κB pathway. We hypothesised that its effect on RA is through FOXC1-mediated signalling pathway. Our study further confirms this hypothesis. However, more in-depth molecular mechanisms and in vivo studies are still needed.
As a member of the fork head box transcription factor family, FOXC1 transcription factor, like other members, can bind to the target gene segment through its own unique fork-head domain (FHD) DNA binding domain, thereby initiating transcription and promoting the expression of specific proteins [Citation19]. FOXC1 mutation is closely associated with abnormal embryonic development. FOXC1 regulates several cellular processes, such as metabolism and differentiation [Citation20]. Interestingly, we here revealed its effects on the proliferation and migration of TNF-α-induced MH7A cells. Similarly, previous study indicated that FOXC1-mediated TRIM22 could mediate the proliferation and inflammation of FLS via NF‑κB pathway, thereby suppressing the progression of RA. We here also confirmed that FOXC1 suppressed the progression of RA via the β-catenin pathway. Notably, another study also indicated that FOXC1 contributed to the proliferation of FLS in RA via PI3K/AKT pathway [Citation21]. These studies suggested that FOXC1 affects the progression of RA via different manners, and the precise mechanism needs further study.
β-catenin pathway not only regulates osteocyte maturation, differentiation and apoptosis, but also directly promotes bone formation [Citation22]. At present, it has been confirmed that the β-catenin pathway is a key factor in the development of rheumatoid arthritis [Citation23]. On the one hand, activation of Wnt/β-catenin pathway promotes osteoblast growth and differentiation, and promotes bone tissue repair. On the other hand, the up-regulation of β-catenin in fibroblast synoviocytes promotes the proliferation of fibroblast synoviocytes, aggravates soft tissue hyperplasia, and destroys bone joints. Our study further confirmed that IRN affected the RA progression and functions of FLS via this axis. We believe that this pathway could serve as a promising target for RA treatment.
In summary, we revealed that IRN attenuates proliferation and migration of FLS in RA via the FOXC1 mediated β-catenin pathway.
Ethical approval
Ethical approval was obtained from the Ethics Committee of Experimental Animals of Nanjing Medical University.
Author’s contribution
All authors contributed to the study conception and design. Material preparation and the experiments were performed by Yingyi Wu. Data collection and analysis were performed by Yan Bian and Jing Fei. The first draft of the manuscript was written by Yang Huang and all authors commented on previous versions of the manuscript. All authors read and approved the final manuscript.
Supplemental Material
Download MS Word (96.5 KB)Acknowledgements
Not applicable.
Disclosure statement
No potential conflict of interest was reported by the author(s).
Data availability statement
All data generated or analysed during this study are included in this published article.
The datasets used and/or analysed during the present study are available from the corresponding author on reasonable request.
Additional information
Funding
References
- Anchi P, Swamy V, Godugu C. Nimbolide exerts protective effects in complete Freund’s adjuvant induced inflammatory arthritis via abrogation of STAT-3/NF-kappaB/notch-1 signaling. Life Sci. 2021;266:1. doi:10.1016/j.lfs.2020.118911
- Anchi P, Panda B, Mahajan RB, et al. Co-treatment of nimbolide augmented the anti-arthritic effects of methotrexate while protecting against organ toxicities. Life Sci. 2022;295:120372. doi:10.1016/j.lfs.2022.120372
- Cui X, Wang R, Bian P, et al. Evaluation of antiarthritic activity of nimbolide against freund’s adjuvant induced arthritis in rats. Artif Cells Nanomed Biotechnol. 2019;47(1):3391–8. doi:10.1080/21691401.2019.1649269
- Israr M, Naseem N, Akhtar T, et al. Nimbolide attenuates complete Freund’s adjuvant induced arthritis through expression regulation of toll-like receptors signaling pathway. Phytother Res. 2023;37(3):903–912. doi:10.1002/ptr.7672
- Ju DT, Tsai BC, Sitorus MA, et al. Curcumin-pretreated adipose-derived stem cells enhance the neuroprotective ability to repair rheumatoid arthritis-induced damage in the rat brain. Am J Chin Med. 2022;50(5):1299–1314. doi:10.1142/S0192415X22500549
- Wu ZF, Wang YQ, Wan N, et al. Structural stabilities and transformation mechanism of rhynchophylline and isorhynchophylline by ultra performance liquid chromatography/time-of-Flight mass spectrometry (UPLC/Q-TOF-MS). Molecules. 2015;20(8):14849–14859. doi:10.3390/molecules200814849
- Yuan D, Ma B, Yang JY, et al. Anti-inflammatory effects of rhynchophylline and isorhynchophylline in mouse N9 microglial cells and the molecular mechanism. Int Immunopharmacol. 2009;9(13–14):1549–1554. doi:10.1016/j.intimp.2009.09.010
- Wang XH, Li X, Qiang W, et al. Comparative transcriptome analysis revealed the molecular mechanism of the effect of light intensity on the accumulation of rhynchophylline and isorhynchophylline in uncaria rhynchophylla. Physiol Mol Biol Plants. 2022;28(2):315–331. doi:10.1007/s12298-022-01142-2
- Wang X, Zheng M, Liu J, et al. Differences of first-pass effect in the liver and intestine contribute to the stereoselective pharmacokinetics of rhynchophylline and isorhynchophylline epimers in rats. J Ethnopharmacol. 2017;209:175–183. doi:10.1016/j.jep.2017.07.039
- Luan Y, Luan Y, Feng Q, et al. Emerging role of mitophagy in the heart: therapeutic potentials to modulate mitophagy in cardiac diseases. Oxid Med Cell Longev. 2021;2021:3259963–3259913.
- Wende AR, O’Neill BT, Bugger H, et al. Enhanced cardiac akt/protein kinase B signaling contributes to pathological cardiac hypertrophy in part by impairing mitochondrial function via transcriptional repression of mitochondrion-targeted nuclear genes. Mol Cell Biol. 2015;35(5):831–846. doi:10.1128/MCB.01109-14
- Liu W, Yan F, Xu Z, et al. Urolithin a protects human dermal fibroblasts from UVA-induced photoaging through NRF2 activation and mitophagy. J Photochem Photobiol B. 2022;232:112462. doi:10.1016/j.jphotobiol.2022.112462
- Hao Y, Ou Y, Zhang C, et al. Seratrodast, a thromboxane A2 receptor antagonist, inhibits neuronal ferroptosis by promoting GPX4 expression and suppressing JNK phosphorylation. Brain Res. 2022;1795:148073. doi:10.1016/j.brainres.2022.148073
- Albasher G, Alkahtani S, Al-Harbi LN. Urolithin a prevents streptozotocin-induced diabetic cardiomyopathy in rats by activating SIRT1. Saudi J Biol Sci. 2022;29(2):1210–1220. doi:10.1016/j.sjbs.2021.09.045
- Li T, Zhou Y, Li D, et al. The role of genome-scale leukocyte long noncoding RNA in identifying acute aortic dissection. SV. 2022;18(3):101–110.
- Wan Q-Y, Liu R-F, Zou Y, et al. A rare variant in the MARVELD2 gene is associated with Chinese samples with ovarian endometriosis. EJGO. 2022;43(1):42–47.
- Zhang C, Wu X, Xian Y, et al. Evidence on integrating pharmacokinetics to find truly therapeutic agent for alzheimer’s disease: comparative pharmacokinetics and disposition kinetics profiles of stereoisomers isorhynchophylline and rhynchophylline in rats. Evid Based Complement Alternat Med. 2019;2019:4016323.
- Chen X, Xu J, Mu X, et al. Effects of rhynchophylline and isorhynchophylline on nitric oxide and endothelin-1 secretion from RIMECs induced by listeriolysin O in vitro. Vet Microbiol. 2010;143(2–4):262–269. doi:10.1016/j.vetmic.2009.11.008
- Liu CL, Zhao D, Li JJ, et al. Inhibition of glioblastoma progression by urolithin a in vitro and in vivo by regulating Sirt1-FOXO1 axis via ERK/AKT signaling pathways. Neoplasma. 2022;69(1):80–94. doi:10.4149/neo_2021_210623N834
- Kim HM, Kang B, Park S, et al. Forkhead box protein D2 suppresses colorectal cancer by reprogramming enhancer interactions. Nucleic Acids Res. 2023;51(12):6143–6155. doi:10.1093/nar/gkad361
- Lin Y, Wang W, Wang Y, et al. MiR-223-3p increases resistance of colorectal cancer cells to 5-fluorouracil via targeting SORBS1. Zhong Nan Da Xue Xue Bao Yi Xue Ban. 2023;48(3):356–368.
- Luan Y, Ren KD, Luan Y, et al. Mitochondrial dynamics: pathogenesis and therapeutic targets of vascular diseases. Front Cardiovasc Med. 2021;8:770574. doi:10.3389/fcvm.2021.770574
- Li K, Yu H, Zhao C, et al. Down-regulation of PRR11 affects the proliferation, migration and invasion of osteosarcoma by inhibiting the wnt/beta-catenin pathway. J Cancer. 2021;12(22):6656–6664. doi:10.7150/jca.62491