Abstract
Dual-specificity phosphatase 12 (DUSP12) is abnormally expressed under various pathological conditions and plays a crucial role in the pathological progression of disorders. However, the role of DUSP12 in cerebral ischaemia/reperfusion injury has not yet been investigated. This study explored the possible link between DUSP12 and cerebral ischaemia/reperfusion injury using an oxygen-glucose deprivation/reoxygenation (OGD/R) model. Marked decreases in DUSP12 levels have been observed in cultured neurons exposed to OGD/R. DUSP12-overexpressed neurons were resistant to OGD/R-induced apoptosis and inflammation, whereas DUSP12-deficient neurons were vulnerable to OGD/R-evoked injuries. Further investigation revealed that DUSP12 overexpression or deficiency affects the phosphorylation of apoptosis signal-regulating kinase 1 (ASK1), c-Jun NH2-terminal kinase (JNK), and p38 mitogen-activated protein kinase (MAPK) in neurons under OGD/R conditions. Moreover, blockade of ASK1 diminished the regulatory effect of DUSP12 deficiency on JNK and p38 MAPK activation. In addition, DUSP12-deficiency-elicited effects exacerbating neuronal OGD/R injury were reversed by ASK1 blockade. In summary, DUSP12 protects against neuronal OGD/R injury by reducing apoptosis and inflammation through inactivation of the ASK1-JNK/p38 MAPK pathway. These findings imply a neuroprotective function for DUSP12 in cerebral ischaemia/reperfusion injury.
1. Introduction
Ischaemic stroke is a common neurological disorder mainly caused by occlusion of the cerebral arteries [Citation1]. The reduction in cerebral blood flow leads to an insufficient supply of oxygen and glucose to the brain, causing neuronal damage and neurological deficits [Citation2]. Rapid blood flow recovery is the main clinical approach for ischaemic stroke; however, it can exacerbate ischaemic brain injury through a phenomenon known as cerebral ischaemia/reperfusion injury [Citation3]. The main pathophysiological events in cerebral ischaemia/reperfusion injury include neuronal apoptosis, inflammatory response, and an imbalance of oxidants and ions, attributed to the dysregulation of genes and signal transduction [Citation4–7]. Therefore, it is imperative to address the underlying pathophysiology of cerebral ischaemia/reperfusion injury and identify the novel and key genes responsible for this disease, which can offer therapeutic candidates for translating into effective therapies.
Dual-specificity phosphatase 12 (DUSP12) is a member of the DUSP family that is capable of dephosphorylating substrates at threonine and tyrosine residues, thus participating in numerous signaling cascades [Citation8]. DUSP12 was originally identified as an anti-apoptotic survival phosphatase via RNA interference [Citation9]. To date, there have been few reports on DUSP12, but its crucial relevance in pathophysiology has attracted particular attention. DUSP12 has oncogenic potential, and its overexpression enhances cell transformation by increasing cell migration and resistance to apoptosis [Citation10]. Increasing DUSP12 expression in cardiac cells ameliorates cardiac hypertrophy and fibrosis in mouse models [Citation11]. DUSP12 can retard the progression of inflammatory reactions in macrophages in response to microbial infections [Citation12]. DUSP12 also protects against lipopolysaccharide-induced lung inflammation and injury [Citation13]. Moreover, DUSP212 serves as a crucial regulator of hepatic lipid metabolism by regulating hepatic steatosis, insulin resistance and inflammation [Citation14].
Apoptosis signal-regulating kinase 1 (ASK1) serves as an upstream kinase in the mitogen-activated protein kinase (MAPK) signaling cascade, which plays key roles in apoptotic cell death and inflammatory responses under numerous pathological conditions [Citation15,Citation16]. Through phosphorylation of MAP2K, ASK1 can activate c-Jun NH2-terminal kinase (JNK) and p38 MAPK, which govern cellular fate [Citation17]. ASK1 is a pivotal mediator of ischaemic stroke [Citation18], is activated after cerebral ischaemia, which is associated with neuronal death and the development of infarct lesions [Citation19]. Inhibition of ASK1 can block the activation of JNK and p38 MAPK, which have protective effects against cerebral ischaemia/reperfusion injury [Citation20]. Therefore, ASK1-JNK/p38MAPK is an attractive research focus owing to its crucial action in cerebral ischaemia/reperfusion injury. However, the regulatory mechanism of ASK1-JNK/p38MAPK remains unclear. Exploring novel upstream regulators of ASK1-JNK/p38MAPK is important for understanding the molecular mechanisms underlying cerebral ischaemia/reperfusion injury.
Recent findings have demonstrated a crucial action of DUSP12 on hepatic and myocardial ischaemia/reperfusion injury [Citation21–23]; however, whether DUSP12 acts on cerebral ischaemia/reperfusion injury remains unknown. This study explored the possible link between DUSP12 and cerebral ischaemia/reperfusion injury using an oxygen-glucose deprivation/reoxygenation (OGD/R) model.
2. Materials and methods
2.1. Neuronal culture and OGD/R model
HT22, a commercial mouse hippocampal neuron cell line, was kindly provided by Procell Life Science and Technology Co., Ltd. (Wuhan, China). HT22 neurons were seeded in culture flasks containing Dulbecco’s modified Eagle’s medium in the presence of 10% foetal bovine serum and 1% penicillin/streptomycin solution. The culture flasks were placed in a carbon dioxide incubator supplied with a humidified atmosphere of 5% CO2 and 95% air for cell growth at 37 °C. To mimic ischaemia/reperfusion in vitro, the OGD/R model was established according to previously described protocols [Citation24]. Briefly, OGD media (glucose- and serum-free Dulbecco’s modified Eagle’s medium) was added to HT22 neurons after washing with PBS, and the cell culture was placed into an anaerobic chamber containing 1% O2, 5% CO2 and 94% N2 at 37 °C for 3 h. Afterwards, the old media was discarded, full-culture media was added to HT22 neurons, and the cell culture was placed in a normoxic incubator for reoxygenation for 24 h to establish the OGD/R model.
2.2. Adenovirus infection
Adenoviral vectors carrying DUSP12 cDNA (Ad-DUSP12) or DUSP12 shRNA (Ad-sh-DUSP12: CCGACCAGCUUACCUUUGAAA), and control adenoviral vectors were purchased from GenePharma (Shanghai, China). HT22 neurons were seeded into a six-well plate and cultured until the cell confluence reached 70%. Before infection, the culture medium was refreshed, and adenoviruses were added to the cells at an MOI of 50. After culturing for 48 h, infected neurons were collected for cellular functional experiments.
2.3. Quantitative real-time PCR (qRT-PCR)
Total RNA was extracted using TRIzol reagent, and reverse transcription reactions for synthesizing cDNA were performed using the cDNA Synthesis Kit (Beyotime, Shanghai, China). To detect the level of target genes, cDNA was mixed with Top Green qPCR SuperMix (TransGen, Beijing, China) and forward/reverse primers for qRT-PCR in a Real-Time PCR System. Relative levels of target genes were assessed using the delta Ct method and plotted as 2−ddCt using β-actin for normalization. The primer sequences for qRT-PCR were as follows: DUSP12, forward: 5′-ACTGAAACTGTATGAGGCAATGG-3′ and reverse: 5′-CCTGTGAAATGGTAGTTGGGTC-3′; β-actin, forward: 5′-GGCTGTATTCCCCTCCATCG-3′ and reverse: 5′-CCAGTTGGTAACAATGCCATGT-3′.
2.4. Western blotting
Proteins were extracted from the treated cell samples and loaded onto sodium dodecyl sulfate-polyacrylamide gels for fractionation. The proteins on the gels were then transferred onto polyvinylidene difluoride membranes. After membrane blocking, immunoblotting was performed using primary and secondary antibodies, following routine protocols. The membranes were treated with ECL luminescence agents and the protein signals were detected using a chemiluminescence detector. The following primary antibodies were used: DUSP12 (#orb539257, 1:1000; Biorbyt, Wuhan, China), Bcl2 (#68103-1-Ig, 1:5000; Sanying Biotech, Wuhan, China), Bax (#50599-2-Ig, 1:2000; Sanying Biotech), BDNF (#25699-1-AP, 1:500; Sanying Biotech), NF-κB p65 (#8242, 1:1000; CST, Shanghai, China), phospho-NF-κB p65 (Ser536, #3033, 1:1000; CST), ASK1 (#8662, 1:1000; CST), phospho-ASK1 (Thr845, orb6390, 1:1000; Biorbyt), JNK (#9252, 1:1000; CST), phospho-JNK (Thr183/Tyr185, #4668, 1:1000; CST), p38 MAPK (#8690, 1:1000; CST), phospho-p38 MAPK (#4511, 1:1000; Thr180/Tyr182; CST), and β-actin (81115-1-RR, 1:5000; Sanying Biotech).
2.5. Cell viability assay
The effect of DUSP12 overexpression or deficiency on the viability of OGD/R-exposed HT22 neurons was assessed using the Calcein AM Cell Viability Assay Kit (Beyotime) according to the manufacturer’s instructions. Briefly, Ad-DUSP12- or Ad-sh-DUSP12-infected cells were seeded in a 96-well black opaque plate at 5000 cells/well. After culturing overnight in a normoxic incubator, the culture medium was replaced with OGD medium and the plate was transferred to an anaerobic chamber for 3 h at 37 °C. Thereafter, the culture medium was replaced with full-culture media and the plate was placed into a standard incubator for reoxygenation for 24 h. Cells were then washed in PBS and Calcein AM solution was added to the plate at 100 μl/well, followed by incubation for 24 h at 37 °C in the dark. A fluorescence microplate reader was used to measure the fluorescence intensity at an excitation wavelength of 494 nm and an emission wavelength of 517 nm.
2.6. TUNEL assay
Ad-DUSP12- or Ad-sh-DUSP12-infected cells were seeded on chamber slides. After OGD/R treatment, cells were incubated with tissue fixation solution for 15 min at room temperature. After washing with PBS, cells were incubated with 0.2% Triton X-100 in PBS for 10 min at 37 °C. The cells were washed with PBS and then treated with TdT Equilibration Buffer for 15 min at 37 °C. The TdT Equilibration Buffer was absorbed using absorbent paper, and the TdT Labelling solution (Elabscience, Wuhan, China) was added to the cells, followed by incubation for 1 h at 37 °C in a dark humidified box. After washing with PBS, the cells were counterstained with DAPI solution for 5 min at room temperature in the dark. After washing with PBS, the slides were sealed with an antifade mounting medium and observed under a fluorescence microscope.
2.7. Flow cytometry assay
Ad-DUSP12- and Ad-sh-DUSP12-infected cells were seeded in 24-well plates. After OGD/R treatment, the cells were dissociated using a Trypsin Solution without EDTA. The cells were washed with PBS and collected by centrifugation. A total of 5 × 105 cells were collected and suspended in 100 μl binding buffer, to which 5 μl Annexin V-FITC and 10 μl PI Staining Solution (Elabscience) were added. After incubation for 10 min at room temperature in the dark, 400 μl binding buffer was added to the suspension. The samples were analyzed by flow cytometry. Cells residing in the quadrants of lower right (LR) (Annexin V+/PI-) and upper right (UR) (Annexin V+/PI+) were identified as apoptotic cells.
2.8. Enzyme-linked immunosorbent assay (ELISA)
The culture medium was collected by centrifugation at 2,000 × g for 10 min. The concentrations of tumor necrosis factor-α (TNF-α), interleukin (IL)-6, and IL-1β in the culture media were determined using the corresponding ELISA kits (Elabscience, Wuhan, China), according to the manufacturer’s instructions. Briefly, cell culture supernatants were harvested through centrifugation and added to the ELISA pate at 100 μl/well. After incubation for 90 min at 37 °C, the liquid was discarded and biotinylated detection Ab working solution was added to the plate at 100 μl/well. After incubation for 60 min at 37 °C, the liquid was discarded and the wells were washed with wash buffer for three times. Thereafter, HRP conjugate working solution was added to the plate at 100 μl/well. After incubation for 30 min at 37 °C, the liquid was discarded and the wells were washed with wash buffer for three times. Substrate reagent was added to the plate at 90 μl/well, followed by incubation for 15 min at 37 °C. Then, stop solution was added to the plate at 50 μl/well and the optical density (OD value) of each well at 450 nm was measured with a micro-plate reader.
2.9. Chemical inhibitor treatment
The highly selective and potent ASK1 inhibitor GS-4997 (Selleck Chemicals, Shanghai, China) was utilized in this work. GS-4997 was dissolved in the vehicle solvent dimethyl sulfoxide for utilization. HT22 neurons were pre-treated with GS-4997 at 2 μM for 1 h before OGD/R treatment.
2.10. Statistical analysis
Data were obtained from three repeated experiments and are expressed as the mean ± standard deviation. GraphPad Prism software (version 8.0) was used to perform all the statistical analyses. Statistical differences were determined using Student’s t-test (two groups only) or one-way analysis of variance (ANOVA) (more than two groups) followed by Tukey’s post-hoc test. Differences were considered statistically significant at p < 0.05.
3. Results
3.1. OGD/R decreases DUSP12 levels in cultured neurons
To analyse the link between DUSP12 and neuronal OGD/R injury, changes in DUSP12 expression upon OGD/R were investigated in HT22 neurons. DUSP12 mRNA levels decreased in HT22 neurons after OGD/R (). Consistently, DUSP12 protein levels also decreased in HT22 neurons after OGD/R ().
Figure 1. DUSP12 expression in OGD/R-exposed neurons and overexpression of DUSP12 by adenovirus. The expression of DUSP12 in HT22 neurons post-OGD/R was examined by (A) qRT-PCR and (B, C) Western blotting. (D, E) HT22 neurons were infected with Ad-DUSP12 or Ad-Ctrl for 48 h and DUSP12 expression was detected by Western blotting. n = 3. **p < 0.01. Statistical differences were determined using Student’s t-test.
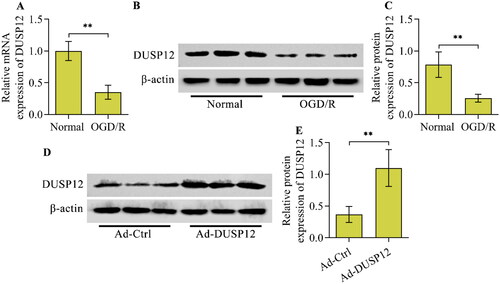
3.2. DUSP12 overexpression alleviates neuronal OGD/R injury
The reduction in DUSP12 expression in OGD/R-exposed neurons prompted us to explore the functional role of DUSP12 in OGD/R injury. Therefore, we generated DUSP12-overexpressed neurons using adenovirus-mediated gene transfer to evaluate the function of DUSP12 in OGD/R injury. Western blotting validated the overexpression of DUSP12 in Ad-DUSP12-infected neurons (). DUSP12-overexpressed neurons were used to establish an OGD/R model (). DUSP12 overexpression had no obvious effect on the viability of neurons cultured under normal conditions (). OGD/R markedly reduced the viability of Ad-Ctrl-infected neurons, whereas the adverse effect of OGD/R on neuronal viability was significantly reduced in Ad-DUSP12-infected neurons (). OGD/R-exposed neurons showed an increased number of TUNEL-positive cells, which were remarkably decreased by DUSP12 overexpression (). The Annexin V-FITC/PI staining assay also demonstrated that the percentage of apoptotic neurons increased by OGD/R was markedly reduced in DUSP12-overexpressed neurons (). The level of the anti-apoptotic protein Bcl2 was decreased and the level of the pro-apoptotic protein Bax was increased in Ad-Ctrl-infected neurons after OGD/R exposure, which were all remarkably reversed in Ad-DUSP12-infected neurons ( and ). In addition, DUSP12 overexpression in neurons increased the expression of the neuroprotective factor BDNF under OGD/R conditions ( and ).
Figure 2. The effect of DUSP12 overexpression on neuronal OGD/R injury. Ad-Ctrl- or Ad-DUSP12-infected HT22 neurons were used to establish the OGD/R model and (A, B) DUSP12 expression was examined by Western blotting. (C) The viability of Ad-Ctrl- or Ad-DUSP12-infected neurons with or without OGD/R was measured by the Calcein AM cell viability assay. The apoptosis of Ad-Ctrl- or Ad-DUSP12-infected neurons with or without OGD/R was assessed by (D, E) TUNEL (scale bar = 100 μm) and (F, G) Annexin V-FITC/PI staining assays. (H, I) Levels of Bcl2, Bax and BDNF in Ad-Ctrl- or Ad-DUSP12-infected neurons with or without OGD/R were detected by Western blotting. n = 3. *p < 0.05, **p < 0.01, and ***p < 0.001. Statistical differences were determined using one-way ANOVA followed by Tukey’s post-hoc test.
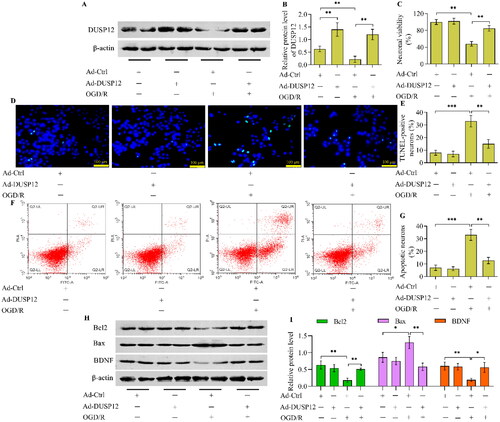
3.3. DUSP12 overexpression represses OGD/R-evoked inflammatory response
OGD/R provoked an inflammatory response in cultured neurons, as evidenced by the increased concentrations of TNF-α, IL-6, and IL-1β (). Critically, OGD/R-evoked production of inflammatory cytokines was markedly blocked in DUSP12-overexpressed neurons (). Moreover, the activation of the classical inflammatory transcription factor NF-κB, evoked by OGD/R, was also repressed by DUSP12 overexpression ().
Figure 3. The effect of DUSP12 overexpression OGD/R-evoked inflammatory response. (A-C) Concentrations of TNF-α, IL-6 and IL-1β in Ad-Ctrl- or Ad-DUSP12-infected neurons with or without OGD/R were quantified by ELISA. (D, E) Levels of phosphorylated NF-κB p65 in Ad-Ctrl- or Ad-DUSP12-infected neurons with or without OGD/R were examined by Western blotting. n = 3. **p < 0.01, and ***p < 0.001. Statistical differences were determined using one-way ANOVA followed by Tukey’s post-hoc test.
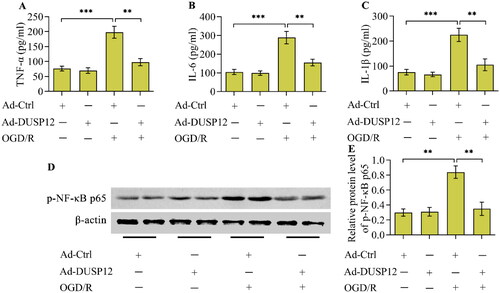
3.4. DUSP12 deficiency renders neurons more susceptible to OGD/R
DUSP12-deficient neurons were used to validate the function of DUSP12 in OGD/R injury. DUSP12 expression was depleted by the infection of Ad-sh-DUSP12 (). Under normoxic conditions, DUSP12 showed no obvious effects on the viability (), apoptosis (), and morphology (Supplementary Figure S1) of HT22 neurons. Notably, the adverse effects of OGD/R on neuronal viability were aggravated in DUSP12-deficient neurons (). OGD/R-induced neuronal apoptosis was also exacerbated by DUSP12 depletion (). DUSP12 depletion decreased the levels of Bcl2 and BDNF while increasing the levels of Bax in OGD/R-exposed neurons ( and ). Moreover, the OGD/R-provoked inflammatory response was enhanced in DUSP12-deficient neurons ().
Figure 4. The effect of DUSP12 deficiency on neuronal OGD/R injury. (A, B) DUSP12 expression in Ad-sh-Scrambled- or Ad-sh-DUSP12-infected HT22 neurons with or without OGD/R was examined by Western blotting. (C) The viability of Ad-sh-Scrambled- or Ad-sh-DUSP12-infected neurons with or without OGD/R was assessed by the Calcein AM cell viability assay. The apoptosis of Ad-sh-Scrambled- or Ad-sh-DUSP12-infected neurons with or without OGD/R was evaluated by the (D, E) TUNEL (scale bar = 100 μm) and (F, G) Annexin V-FITC/PI staining assays. (H, I) Levels of Bcl2, Bax and BDNF in Ad-sh-Scrambled- or Ad-sh-DUSP12-infected neurons with or without OGD/R were examined by Western blotting. n = 3. *p < 0.05, **p < 0.01, and ***p < 0.001. Statistical differences were determined using one-way ANOVA followed by Tukey’s post-hoc test.
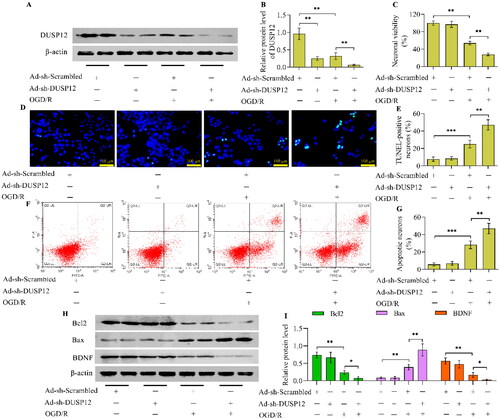
Figure 5. The effect of DUSP12 deficiency on the OGD/R-evoked inflammatory response. (A-C) Concentrations of TNF-α, IL-6 and IL-1β in Ad-sh-Scrambled- or Ad-sh-DUSP12-infected neurons with or without OGD/R were quantified by ELISA. (D, E) Levels of phosphorylated NF-κB p65 in Ad-sh-Scrambled- or Ad-sh-DUSP12-infected neurons with or without OGD/R were detected by Western blotting. n = 3. **p < 0.01, and ***p < 0.001. Statistical differences were determined using one-way ANOVA followed by Tukey’s post-hoc test.
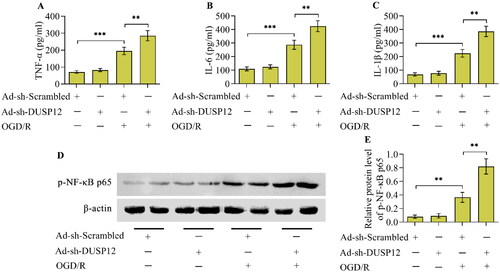
3.5. DUSP12 affects the activation of ASK1-JNK/p38 MAPK stimulated by OGD/R
To date, the well-known function of DUSP12 is to regulate the ASK1-JNK/p38 MAPK signaling axis. Considering that this signaling axis plays a crucial role in neuronal OGD/R injury, this study further investigated whether DUSP12 adjusts the ASK1-JNK/p38 MAPK signaling axis under OGD/R conditions. The levels of phosphorylated ASK1, JNK, and p38, the activated forms of these kinases, increased in OGD/R-exposed neurons (). As expected, the levels of phosphorylated ASK1, JNK, and p38 increased by OGD/R were downregulated by DUSP12 overexpression () or upregulated by DUSP12 deficiency ().
Figure 6. The effect of DUSP12 overexpression on ASK1-JNK/p38 MAPK under OGD/R conditions. (A-D) The levels of phosphorylated ASK1, JNK and p38 in Ad-Ctrl- or Ad-DUSP12-infected neurons with or without OGD/R were determined by Western blotting and their protein quantification was shown. n = 3. **p < 0.01. Statistical differences were determined using one-way ANOVA followed by Tukey’s post-hoc test.
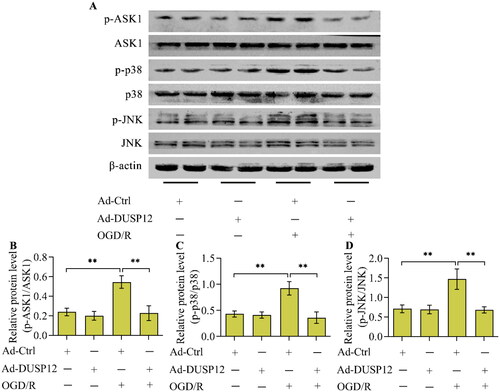
Figure 7. The effect of DUSP12 deficiency on ASK1-JNK/p38 MAPK under OGD/R conditions. (A-D) The levels of phosphorylated ASK1, JNK and p38 in Ad-sh-Scrambled- or Ad-sh-DUSP12-infected neurons with or without OGD/R were determined by Western blotting and their protein quantification was shown. n = 3. **p < 0.01. Statistical differences were determined using one-way ANOVA followed by Tukey’s post-hoc test.
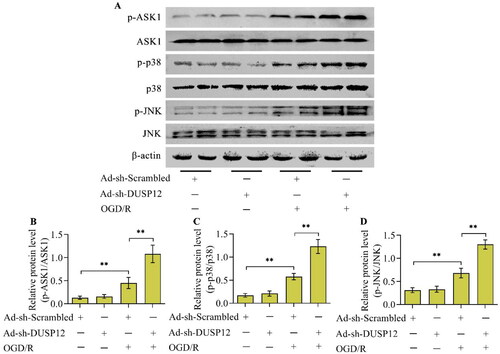
3.6. ASK1 is required for the regulatory effect of DUSP12 on JNK/p38 MAPK
To validate whether DUSP12 affects the activation of JNK/p38 MAPK via ASK1, the ASK1 inhibitor, GS-4997, was used to inactivate ASK1 in DUSP12-deficient neurons. Adding GS-4997 to Ad-sh-DUSP12-infected neurons effectively activated ASK1 under OGD/R conditions (). The effect of DUSP12 deficiency on the levels of phosphorylated JNK and p38 in OGD/R-exposed neurons was reversed by ASK1 inhibition (, and D).
Figure 8. The effect of ASK1 inhibition on DUSP12-mediated JNK/p38 MAPK. Ad-sh-DUSP12-infected neurons were treated with the ASK1 inhibitor GS-4997 and subjected to OGD/R. (A, D) Levels of DUSP12 and phosphorylated ASK1, JNK and p38 were examined by Western blotting and their protein quantification was shown. n = 3. **p < 0.01 and #p > 0.05. Statistical differences were determined using one-way ANOVA followed by Tukey’s post-hoc test.
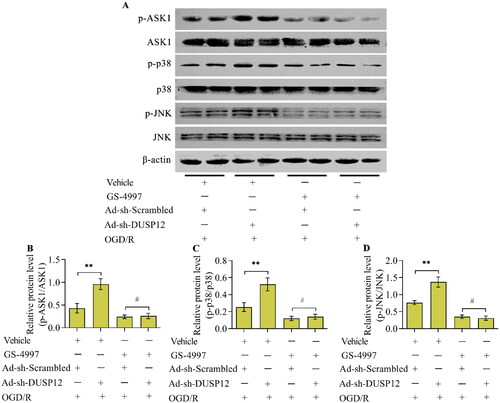
3.7. The inhibition of ASK1 diminishes the effect of DUSP12 deficiency on neuronal OGD/R injury
To verify that ASK1 is essential for the function of DUSP12 in OGD/R injury, the effect of ASK1 inhibition on DUSP12-deficiency-induced OGD/R injury was investigated. The results demonstrated that the inhibition of ASK1 significantly decreased OGD/R-induced apoptosis () and the inflammatory response (). However, the exacerbating effect of DUSP12 deficiency on OGD/R-induced apoptosis () and the inflammatory response () was diminished in neurons treated with ASK1 inhibitors.
Figure 9. The effect of ASK1 inhibition on DUSP12-mediated OGD/R injury. Neuronal apoptosis in different groups was monitored by the (A, B) TUNEL (scale bar = 100 μm) and (C, D) Annexin V-FITC/PI staining assays. (E, F) Concentrations of TNF-α, IL-6 and IL-1β in different groups were quantified by ELISA. n = 3. **p < 0.01 and #p > 0.05. Statistical differences were determined using one-way ANOVA followed by Tukey’s post-hoc test.
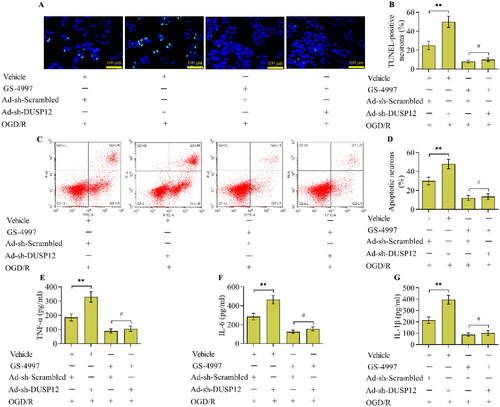
4. Discussion
DUSP12 has attracted considerable attention in pathology research because of its critical actions in numerous pathological conditions, including cardiac hypertrophy, microbial infection, acute lung injury, and hepatic steatosis [Citation11–14]. Notably, recent findings have documented a crucial action of DUSP12 in hepatic ischaemia/reperfusion injury [Citation21,Citation22]. DUSP12 was found to be a novel protective regulator against ischaemia/reperfusion-induced injury in the liver [Citation21]. Moreover, DUSP12 protects hepatocytes from hypoxia/reoxygenation-evoked injuries in vitro [Citation21]. A recent study indicates that the overexpression of DUSP12 also safeguards the heart from injuries induced by ischaemia/reperfusion [Citation23]. To date, the effect of DUSP12 on ischaemia/reperfusion-evoked brain injury has not been studied. Here, we explored the possible link between DUSP12 and ischaemia/reperfusion-evoked injury in the brain using an OGD/R model. DUSP12 levels decreased in OGD/R-exposed neurons. DUSP12-overexpressed neurons are resistant to OGD/R injury, whereas DUSP12-deficient neurons are more susceptible to OGD/R injury. Therefore, DUSP12 may have a neuroprotective function in neurons under OGD/R conditions. DUSP12 may serve as a protective molecule against ischaemia/reperfusion-evoked injury of organs, including the brain.
DUSP12 is a pro-survival phosphatase that enables cell survival and represses apoptosis under various apoptotic stimuli [Citation25]. Overexpression of DUSP12 suppresses lipopolysaccharide-induced apoptosis in lung vascular endothelial cells [Citation13]. In hypoxia/reoxygenation-stimulated hepatocytes, increasing DUSP12 expression suppressed cell apoptosis associated with the up-regulation of Bcl2 and down-regulation of Bax [Citation21]. Therefore, DUSP12 is a pro-survival gene with an anti-apoptotic function in cells under adverse conditions. Consistently, our results demonstrated that overexpression of DUSP12 increased the viability of cultured neurons under OGD/R conditions and alleviated OGD/R-induced neuronal apoptosis associated with the upregulation of Bcl2 and downregulation of Bax. Our observations confirmed that DUSP12 has a pro-survival function in neurons under OGD/R conditions.
The critical role of DUSP12 in the progression of the inflammatory response has also been highlighted. DUSP12 protects against hepatic inflammation caused by a high-fat diet or ischaemia/reperfusion [Citation14,Citation21]. DUSP12 inhibits the expression of pro-inflammatory cytokines and chemokines through the inactivation of NF-κB [Citation14,Citation21]. Overexpression of DUSP12 in macrophages reduces the production of pro-inflammatory cytokines and chemokines in response to mycobacterial infection or toll-like receptor 4 activation [Citation12]. Overexpression of DUSP12 in mice alleviated lipopolysaccharide-induced inflammatory cell infiltration in the lung [Citation13]. In addition to microglia and astrocytes, neurons also generate inflammatory factors in response to harmful stimuli [Citation26–30]. Considering that OGD/R induces an inflammatory response in neurons [Citation31–33], we determined the relevance of DUSP12 in OGD/R-induced inflammation. In accordance with these findings, our observations showed that overexpression of DUSP12 reduced the generation of TNF-α, IL-6, and IL-1β in OGD/R-evoked neurons associated with the inactivation of NF-κB. Therefore, our results suggest that DUSP12 is an anti-inflammatory molecule that may play a critical role in inflammation-related pathological conditions.
ASK1-JNK/p38MAPK has been proposed to be a pivotal signaling cascade in ischaemia/reperfusion-evoked injury of organs, including the brain [Citation34–37]. ASK1-JNK/p38 MAPK is a well-characterized downstream target of DUSP12 [Citation11–14]. It has been reported to DUSP12 directly interacts with ASK1 to inactivate ASK1 [Citation14]. Through the inactivation of the ASK1-JNK/p38 MAPK signaling cascade, DUSP12 has regulatory effects on cell apoptosis and inflammation [Citation11–14]. Considering that ASK1-JNK/p38 MAPK activated by OGD/R also contributes to neuronal injury, we determined whether DUSP12 affects the activation of ASK1-JNK/p38 MAPK under OGD/R conditions. As expected, the OGD/R-induced phosphorylation of ASK1, JNK, and p38 was effectively reduced in DUSP12-overexpressed neurons or enhanced in DUSP12-deficient neurons, confirming that DUSP12 adjusts ASK1-JNK/p38 MAPK in neurons under OGD/R conditions. To confirm that ASK1 acts as a downstream effector of DUSP12, we measured the effect of ASK1 inhibition on DUSP12-deficiency-mediated effects. In neurons treated with ASK1 inhibitors, the enhancing effect of DUSP12 deficiency on JNK and p38 activation was abolished. Moreover, the effect of DUSP12 deficiency on exacerbating neuronal OGD/R injury was diminished in neurons with ASK1 repression. Collectively, DUSP12 protects against neuronal OGD/R injury via inactivation of the ASK1-JNK/p38 MAPK signaling cascade.
In conclusion, the present study shows that DUSP12 serves as a novel mediator of neuronal OGD/R injury and that DUSP12-mediated neuroprotective effects against OGD/R injury depend on the ASK1- JNK/p38 MAPK pathway. These findings provide a novel molecular mechanism for OGD/R-related neuronal apoptosis and inflammation, and suggest a neuroprotective function for DUSP12 in cerebral ischaemia/reperfusion injury. However, further investigation using animal models is required to determine the precise function and mechanism of DUSP12 in cerebral ischaemia/reperfusion injury.
Authors’ contributions
Jiaxuan He designed the study, performed the experiments, and wrote the manuscript. Siyuan Li, Yunpeng Teng, Hongfei Xiong, and Zhuang Wang performed experiments. Xiaoyao Han and Wei Gong performed data analysis and provided technical support. Ya Gao contributed to the conceptualization and reviewed the manuscript. All authors have read and approved the final version of the manuscript.
Acknowledgments
Not applicable.
Disclosure statement
The authors report there are no competing interests to declare.
Data availability statement
The datasets used in this study are available from the corresponding author upon reasonable request.
Additional information
Funding
References
- Mosconi MG, Paciaroni M. Treatments in ischemic stroke: current and future. Eur Neurol. 2022;85(5):349–366. doi:10.1159/000525822
- Balch MHH, Nimjee SM, Rink C, et al. Beyond the brain: the systemic pathophysiological response to acute ischemic stroke. J Stroke. 2020;22(2):159–172. doi:10.5853/jos.2019.02978
- Liang TY, Peng SY, Ma M, et al. Protective effects of sevoflurane in cerebral ischemia reperfusion injury: a narrative review. Med Gas Res. 2021;11(4):152–154.
- Zhang Q, Jia M, Wang Y, et al. Cell death mechanisms in cerebral Ischemia-Reperfusion injury. Neurochem Res. 2022;47(12):3525–3542. doi:10.1007/s11064-022-03697-8
- Jurcau A, Simion A. Neuroinflammation in cerebral ischemia and ischemia/reperfusion injuries: from pathophysiology to therapeutic strategies. Int J Mol Sci. 2021;23(1):14. doi:10.3390/ijms23010014
- Mo Z, Zeng Z, Liu Y, et al. Activation of wnt/Beta-Catenin signaling pathway as a promising therapeutic candidate for cerebral ischemia/reperfusion injury. Front Pharmacol. 2022;13:914537. doi:10.3389/fphar.2022.914537
- Wang L, Liu Y, Zhang X, et al. Endoplasmic reticulum stress and the unfolded protein response in cerebral ischemia/reperfusion injury. Front Cell Neurosci. 2022;16:864426. doi:10.3389/fncel.2022.864426
- Huang CY, Tan TH. DUSPs, to MAP kinases and beyond. Cell Biosci. 2012;2(1):24. doi:10.1186/2045-3701-2-24
- MacKeigan JP, Murphy LO, Blenis J. Sensitized RNAi screen of human kinases and phosphatases identifies new regulators of apoptosis and chemoresistance. Nat Cell Biol. 2005;7(6):591–600. doi:10.1038/ncb1258
- Cain EL, Braun SE, Beeser A. Characterization of a human cell line stably over-expressing the candidate oncogene, dual specificity phosphatase 12. PLoS One. 2011;6(4):e18677. doi:10.1371/journal.pone.0018677
- Li WM, Zhao YF, Zhu GF, et al. Dual specific phosphatase 12 ameliorates cardiac hypertrophy in response to pressure overload. Clin Sci (Lond). 2017;131(2):141–154. doi:10.1042/CS20160664
- Cho SSL, Han J, James SJ, et al. Dual-Specificity phosphatase 12 targets p38 MAP kinase to regulate macrophage response to intracellular bacterial infection. Front Immunol. 2017;8:1259. doi:10.3389/fimmu.2017.01259
- Hui Z, Jie H, Fan GH. Expression of DUSP12 reduces lung vascular endothelial cell damage in a murine model of lipopolysaccharide-induced acute lung injury via the apoptosis signal-regulating kinase 1 (ASK1)-jun N-Terminal kinase activation (JNK) pathway. Med Sci Monit. 2021;27:e930429.
- Huang Z, Wu LM, Zhang JL, et al. Dual specificity phosphatase 12 regulates hepatic lipid metabolism through inhibition of the lipogenesis and apoptosis signal-regulating kinase 1 pathways. Hepatology. 2019;70(4):1099–1118. doi:10.1002/hep.30597
- Ogier JM, Nayagam BA, Lockhart PJ. ASK1 inhibition: a therapeutic strategy with multi-system benefits. J Mol Med (Berl). 2020;98(3):335–348. doi:10.1007/s00109-020-01878-y
- Obsilova V, Honzejkova K, Obsil T. Structural insights support targeting ASK1 kinase for therapeutic interventions. Int J Mol Sci. 2021;22(24):13395. doi:10.3390/ijms222413395
- Rusnak L, Fu H. Regulation of ASK1 signaling by scaffold and adaptor proteins. Adv Biol Regul. 2017;66:23–30. doi:10.1016/j.jbior.2017.10.003
- Cheon SY, Kim EJ, Kim JM, et al. Cell Type-Specific mechanisms in the pathogenesis of ischemic stroke: the role of apoptosis signal-regulating kinase 1. Oxid Med Cell Longev. 2018;2018:2596043–2596049.
- Shiizaki S, Naguro I, Ichijo H. Activation mechanisms of ASK1 in response to various stresses and its significance in intracellular signaling. Adv Biol Regul. 2013;53(1):135–144. doi:10.1016/j.jbior.2012.09.006
- Li F, Mao Q, Wang J, et al. Salidroside inhibited cerebral ischemia/reperfusion-induced oxidative stress and apoptosis via Nrf2/Trx1 signaling pathway. Metab Brain Dis. 2022;37(8):2965–2978. doi:10.1007/s11011-022-01061-x
- Qiu T, Wang T, Zhou J, et al. DUSP12 protects against hepatic ischemia-reperfusion injury dependent on ASK1-JNK/p38 pathway in vitro and in vivo. Clin Sci (Lond). 2020;134(17):2279–2294. doi:10.1042/CS20191272
- Boldorini R, Clemente N, Alchera E, et al. DUSP12 acts as a novel endogenous protective signal against hepatic ischemia-reperfusion damage by inhibiting ASK1 pathway. Clin Sci (Lond). 2021;135(1):161–166. doi:10.1042/CS20201091
- Cheng J, Ji M, Jing H, et al. DUSP12 ameliorates myocardial ischemia-reperfusion injury through HSPB8-induced mitophagy. J Biochem Mol Toxicol. 2023;37(5):e23310. doi:10.1002/jbt.23310
- Wang J, Ren B, Yang Y, et al. C1q/tumor necrosis factor-related protein-9 exerts antioxidant and anti-inflammatory effects on oxygen-glucose deprivation/reoxygenation-stimulated neurons by modulating the Akt-GSK-3beta-Nrf2 Cascade via AdipoR1. Int Immunopharmacol. 2023;118:110045. doi:10.1016/j.intimp.2023.110045
- Sharda PR, Bonham CA, Mucaki EJ, et al. The dual-specificity phosphatase hYVH1 interacts with Hsp70 and prevents heat-shock-induced cell death. Biochem J. 2009;418(2):391–401. doi:10.1042/BJ20081484
- Zhang H, Feng Y, Si Y, et al. Shank3 ameliorates neuronal injury after cerebral ischemia/reperfusion via inhibiting oxidative stress and inflammation. Redox Biol. 2024;69:102983. doi:10.1016/j.redox.2023.102983
- Li X, Fu J, Guan M, et al. Biochanin a attenuates spinal cord injury in rats during early stages by inhibiting oxidative stress and inflammasome activation. Neural Regen Res. 2024;19(9):2050–2056. doi:10.4103/1673-5374.390953
- Su L, Lu H, Zhang D, et al. Total paeony glycoside relieves neuroinflammation to exert antidepressant effect via the interplay between NLRP3 inflammasome, pyroptosis and autophagy. Phytomedicine. 2024;128:155519. doi:10.1016/j.phymed.2024.155519
- Luo L, Wang S, Liu W, et al. Narirutin attenuates cerebral ischemia-reperfusion injury by suppressing the TXNIP/NLRP3 pathway. Neurochem Res. 2024;49(3):692–705. doi:10.1007/s11064-023-04062-z
- Tang S, Lai N, Xu L. Neuronal pyroptosis mediated by STAT3 in early brain injury after subarachnoid hemorrhage. Brain Res. 2024;1822:148666. doi:10.1016/j.brainres.2023.148666
- Zhang Y, Ye P, Zhu H, et al. Neutral polysaccharide from gastrodia elata alleviates cerebral ischemia-reperfusion injury by inhibiting ferroptosis-mediated neuroinflammation via the NRF2/HO-1 signaling pathway. CNS Neurosci Ther. 2024;30(3):e14456. doi:10.1111/cns.14456
- Yang F, Lian Q, Zhang X, et al. Aucubin provides protection against cerebral ischaemia-reperfusion injury by suppressing neuronal apoptosis, oxidative stress, and inflammation through the modulation of the AKT-GSK-3beta-Nrf2 signal cascade. Toxicol Appl Pharmacol. 2024;483:116829. doi:10.1016/j.taap.2024.116829
- Liu C, Chen H, Tao X, et al. ALKBH5 protects against stroke by reducing endoplasmic reticulum stress-dependent inflammation injury via the STAT5/PERK/EIF2alpha/CHOP signaling pathway in an m(6)A-YTHDF1-dependent manner. Exp Neurol. 2024;372:114629. doi:10.1016/j.expneurol.2023.114629
- Xu W, Zhang L, Zhang Y, et al. TRAF1 exacerbates myocardial ischemia reperfusion injury via ASK1-JNK/p38 signaling. J Am Heart Assoc. 2019;8(21):e012575. doi:10.1161/JAHA.119.012575
- Qin JJ, Mao W, Wang X, et al. Caspase recruitment domain 6 protects against hepatic ischemia/reperfusion injury by suppressing ASK1. J Hepatol. 2018;69(5):1110–1122. doi:10.1016/j.jhep.2018.06.014
- Chen J, Wang Q, Zhou W, et al. GPCR kinase 2-interacting protein-1 protects against ischemia-reperfusion injury of the spinal cord by modulating ASK1/JNK/p38 signaling. Faseb J. 2018;18:fj201800548.
- Tesch GH, Ma FY, Nikolic-Paterson DJ. ASK1: a new therapeutic target for kidney disease. Am J Physiol Renal Physiol. 2016;311(2):F373–81. doi:10.1152/ajprenal.00208.2016