Abstract
Context
Parkinson's disease is a common chronic neurodegenerative disease characterized by massive loss of dopaminergic neurons in the substantia nigra. Neuroinflammation has been shown to play an important role in the pathogenesis of neurodegenerative diseases such as Parkinson’s disease. The role of immune tolerance in neuroinflammation and neurodegenerative diseases induced by peripheral factors is unclear.
Objective
This study established a model of endotoxin tolerance to explore the protective effect of endotoxin tolerance on Parkinson-like changes induced by repeated peripheral injections of high-dose LPS, and to explore its inflammatory mechanism.
Materials and methods
In this study, mice were injected intraperitoneally with low dose (0.5 mg/kg) LPS for 4 days to induce endotoxin tolerance (ET). Then, high-dose (1 mg/kg) LPS was injected continuously intraperitoneally for 4 days to induce Parkinson-like changes. Cytokines were detected by enzyme-linked immunosorbent assay (ELISA) and quantitative real-time polymerase chain reaction (qRT-PCR). Activation of microglial cells was detected by protein expression of CD68 and ionized calcium binding adapter molecule 1(Iba-1) by Western blotting and immunofluorescence. Hematoxylin and eosin staining and expression of tyrosine hydroxylase (TH) and dopamine (DA) were used to assess dopaminergic neuronal injury. The open field test and muscle tension test were used to assess behavioral disorders.
Results
As expected, compared with non-ET animals, ET preconditioning significantly reduced the production of inflammatory cytokines in the substantia nigra, inhibited microglial activation, and alleviated the pathological changes of dopaminergic neurons.
Conclusions
ET may be a promising intervention method for neurodegenerative diseases.
ET was successfully induced by continuous low-dose intraperitoneal LPS injection in mice.
ET pretreatment inhibited neuroinflammation in the SN induced by continuous peripheral high doses of LPS.
ET pretreatment inhibited continuous peripheral high-dose LPS injection-induced microglial activation in the SN.
ET pretreatment decreased LPS-induced functional impairment of dopaminergic neurons.
ET reversed the morphological changes of dopaminergic neurons induced by peripheral high-dose LPS.
ET pretreatment improved continuous peripheral high-dose LPS injection-induced behavioral impairment.
Highlights
Introduction
Parkinson’s disease (PD) is a common chronic neurodegenerative disease with clinical manifestations that include static tremor and progressive motor dysfunction. Neuroinflammation plays an important role in the pathogenesis of PD [Citation1]. The hallmarks of neuroinflammation include the activation of microglia and the release of pro-inflammatory cytokines. These two factors have been shown to be involved in the development of neuronal degeneration [Citation2,Citation3].
The peripheral immune system is also involved in the pathogenesis of PD [Citation4]. Inflammatory proteins are overexpressed and infiltration of microglia from the periphery are detected in the vicinity of denatured neurons in PD patients [Citation5]. In rodents, a single intraperitoneal injection of lipopolysaccharide (LPS) of 5 mg/kg resulted in acute microglial activation in the brain lasting for at least 12 months and loss of dopaminergic neurons in the substantia nigra (SN) 10 months later [Citation6,Citation7]. Several days of continuously injected LPS at 1 mg/kg, or chronic LPS exposure, can cause increased neurodegeneration and has been used as a model for PD [Citation8,Citation9]. In order to better simulate the central chronic inflammatory injury caused by repeated peripheral inflammatory stimulation, the present study used intraperitoneal injection of LPS at 1 mg/kg for four consecutive days to observe neuroinflammation and dopaminergic neuronal injury after 28 days [Citation10,Citation11].
The literature has confirmed that anti-inflammatory treatment is effective in neurodegenerative diseases such as Alzheimer’s disease and PD [Citation12–14]. However, the efficacy of non-steroidal anti-inflammatory drugs is uncertain [Citation15,Citation16], and toxic effects cannot be effectively avoided [Citation17]. Hence, nonsteroidal anti-inflammatory drugs failed to achieve wide application in central nervous system brain injury. Therefore, new methods to combat neuroinflammation are needed, one of which, immune control, has shown promise. Previous studies have shown that, immunosuppressant azathioprine, plasmapheresis, and immunoglobulin treatment may halt or partially reverse PD-like changes [Citation18].
Immune tolerance refers to the reduced responsiveness of the immune system when the body is continuously exposed to the threat of antigens, which results in decreased secretion of pro-inflammatory factors, increased secretion of anti-inflammatory factors, and inhibition of T-cell activation [Citation19]. Immune tolerance is a protective mechanism of the body in the face of continuous antigen threat or continuous attack, which reduces an excessive inflammatory response and unnecessary secondary damage [Citation20]. Endotoxin tolerance (ET) induced by low-dose LPS is considered to be the most important component of immune tolerance [Citation21]. ET has been shown to alleviate the process of a variety of neurological diseases, including middle cerebral artery occlusion syndrome [Citation22], brain trauma [Citation23], and Alzheimer's disease [Citation24]. These results show that ET plays an important role in neuroprotection. Others studies indicated that ET can alleviate the intracranial inflammation induced by one-time intracranial injection of LPS and thus promote protection against dopaminergic neuronal injury [Citation4]. However, whether ET also has a protective effect on peripheral LPS-induced chronic neuroinflammation and Parkinson-like changes remains to be verified. Therefore, in this study, an inflammation-induced PD model was established by intraperitoneal injection of high-dose LPS (1 mg/kg) for four consecutive days to observe the protective effect of ET on neuroinflammation and dopaminergic neuronal injury and to explore its inflammatory mechanism to provide an experimental basis for the clinical application of ET. These results may provide a novel therapeutic strategy for neuroinflammation-related neurodegenerative diseases.
Materials and methods
Animal care and LPS treatment
Male C57BL/6J mice (8 weeks old, 18–22 g, Liaoning Changsheng Biotechnology Co., Ltd., Liaoning, China) altogether 70 animals were used in the current study. Mice were housed in Polypropylene cages (290 × 178 × 160 mm), five mice per cage. The mice were allowed free access to food (Rats and mice maintain feed, Liaoning Changsheng Biotechnology Co., Ltd., Liaoning, China) and water, and were housed at a constant temperature of 22 ± 1 °C, with 60% humidity and a light and dark cycle of 12 h/12 h. No exclusion criteria were predetermined. All experimental procedures were approved by Ethics Committee of Harbin Medical University (Harbin, China, HMUPHIRB2016006, 4 March 2016). This study was not preregistered.
Animals were acclimated for one week prior to treatment. The mice were randomly divided into groups with a random number table. In the first part of the experiment, the mice were randomly divided into the following three groups (n = 10 for each group): Group I (control): Animals received four phosphate buffered saline (PBS) injections on four consecutive days; Group II (1 × LPS): A single LPS (no. L6386, strain Salmonella enterica serotype typhimurium, Sigma, St. Louis, MO, USA) at 0.5 mg/kg injection followed by three PBS injections on the following three days; and Group III (4 × LPS): Animals received four LPS at 0.5 mg/kg injections on four consecutive days. Drug delivery mode according to the method of Wendeln [Citation24], as shown in .
Figure 1. Experimental layout of protective effects of endotoxin tolerance on peripheral lipopolysaccharide-induced dopaminergic neuronal injury.
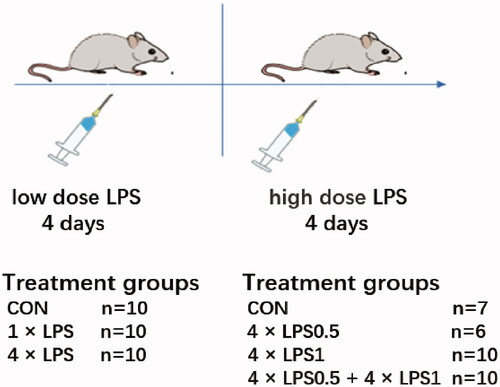
In the second part of the experiment, the mice were divided into another four groups: Group I (control, n = 7): Animals received eight PBS injections on eight consecutive days; Group II (4 × LPS0.5, n = 6): LPS at 0.5 mg/kg was injected for four consecutive days, after which PBS injection was given for four consecutive days; Group III (4 × LPS1, n = 10): PBS injection was given for four consecutive days, after which LPS at 1 mg/kg was injected for four consecutive days, dosage regimen according to the method of Beier [Citation11]; and Group IV (4 × LPS0.5 + 4 × LPS1, n = 10): LPS injection at 0.5 mg/kg was given for four consecutive days, after which LPS at 1 mg/kg was injected for four consecutive days, as shown in . No animals died during experiments. Each group of animals was sacrificed after 36 days of treatment. The weight of mice in each group was recorded at 9 am every day.
Western blotting
Mice were sacrificed after cervical vertebra was severed and the brain was taken. The midbrain tissues of mice were placed in a tube that contained lysis buffer supplemented with phenylmethanesulfonyl fluoride (Beyotime Biotechnology Co., Ltd, Shanghai, China) and allowed to lyse for 2 h at 4 °C, after which the lysed homogenate was centrifuged at 12,000 × g for 10 min at 4 °C. The resultant supernatant protein concentration was determined using a bicinchoninic acid reagent kit (BCA, cat. no. P0010S, Beyotime). Total protein (50 μg) was separated by 10% sodium dodecyl sulfate polyacrylamide gel electrophoresis and transferred to polyvinylidene fluoride membranes. The membranes were then blocked with 5% skim milk powder in Tris-buffered saline Tween (TBST) for 1.5 h at room temperature and incubated overnight at 4 °C with antibodies against tyrosine hydroxylase (TH, rabbit Monoclonal, cat. no.58844, 1:1000; CST), CD68 (rabbit Monoclonal, cat. no. 97778, 1:1000; CST), ionized calcium binding adapter molecule 1(Iba-1, goat polyclonal, cat. no. ab48004, 1: 1000; Abcam), and β-actin (rabbit polyclonal, cat. no. AC026, 1:50000; ABclonal). The next day, the membranes were washed three times (8 min/wash) in TBST and then incubated with horseradish peroxidase goat anti- rabbit IgG (cat. no. RS0002, 1:5000; ImmunoWay) or rabbit anti- goat IgG (cat. no.RS030421, 1:5000; ImmunoWay) for 1 h at room temperature. The membranes were then washed three times in TBST and high sensitive ECL luminescence reagent (Beyotime) was used to develop the protein bands. Band intensities were analyzed using Image J (v 1.51; NIH, Bethesda, MD, USA).
Enzyme-linked immunosorbent assay (ELISA)
For tumor necrosis factor-alpha (TNF-α), interleukin-1β (IL)-1β, IL-10 in the SN, and dopamine (DA) in the striatum measurements, brain homogenates were centrifuged at 5,000 × g for 10 min at 4 °C. Supernatants were analyzed using respective ELISA kits (Elabscience Biotechnology Co., Ltd., Wuhan, China) according to the manufacturer’s instructions. For analyses of brain homogenates, protein levels were normalized against total protein as measured by the bicinchoninic acid reagent kit protein assay (Beyotime).
Quantitative real-time PCR (qRT-PCR)
The TB Green I chimeric fluorescence method was used for qRT-PCR. Total RNA was extracted using Trizol (Invitrogen, Carlsbad, CA, USA) and reverse transcribed using PrimeScript RT reagent Kit with gDNA Eraser. PCR amplification was performed with TB Premix Ex Taq II Reagent Kit (TaKara Bio, Inc., Shiga, Japan) according to the manufacturers’ instructions. The following mouse-specific primer sequences were used for amplification (all from Generay Biotech, Co., Ltd., Shanghai, China): β-actin: forward GGACCTGACAGACTACCTCAT, reverse GCTCGAAGTCTAGAGCAACATAG; TNF-α: forward CGATGGGTTGTACCTTGTCTAC, reverse GCAGAGAGGAGGTTGACTTTC; IL-1β: forward ACTCCTTAGTCCTCGGCCA, reverse CCATCAGAGGCAAGGAGGAA; IL-10: forward GCTCTTACTGACTGGCATGAG, reverse CGCAGCTCTAGGAGCATGTG. Relative mRNA expression levels of the target genes were normalized to β-actin expression using the 2−ΔΔCT method (where ΔCT is the CT target gene- CT β-actin).
Immunofluorescence analysis
After 1% pentobarbital at 50 mg/kg anesthesia, brain tissue was fixed by cardiac perfusion with 4% paraformaldehyde in PBS (0.01 M, pH7.2). After fixation, the tissue was dehydrated in sucrose gradients (concentration 20% to 30%). After optimal cutting temperature compound embedding, the tissue was sliced into 10 μm thick coronal sections on a freezing microtome (CM 1900; Leica Microsystems Gmbh, Wetzlar, Germany) and mounted onto poly-L-lysine-coated slides. The sections were washed three times with PBS (5 min/wash), blocked with 2% donkey serum for 40 min at room temperature, and then incubated with primary rabbit anti-mouse CD68 antibody (cat. no. 97778, dilution, 1:200; CST) and goat anti-mouse Iba-1 antibody (cat. no. 011-27991, dilution, 1:500; Wako) or rabbit anti-mouse TH antibody (cat. no.58844, dilution, 1:200; CST) and goat anti-mouse Iba-1 antibody (cat. no. 011-27991, dilution, 1:500; Wako) overnight at 4 °C. The sections were next washed three times with PBS (5 min/wash), incubated with Alexa Fluor® 594 donkey anti-rabbit IgG (cat. no. ab150076, 1:200; Abcam) and Alexa Fluor® 488 donkey anti-goat IgG (cat. no. ab150129, 1:200; Abcam) for 30 min at 37 °C and washed three times again with PBS (5 min/wash). Finally, dried, mounted by neutral balata agent, and photographed under a fluorescence microscope (BX73; Olympus Corporation, Tokyo, Japan).
Hematoxylin and eosin (HE) staining
Neuronal morphology in the SN was observed by HE staining. The midbrain tissue was fixed with 4% paraformaldehyde in PBS and, after ethanol gradient dehydration, vitrification by dimethylbenzene, and paraffin embedding, was sectioned at a thickness of 4 μm. Next, the sections were placed in xylene for dewaxing and subsequently in ethanol, to remove xylene, after which they were incubated with hematoxylin for 15 min at room temperature to stain the cell nuclei. Finally, the sections were incubated with eosin and mounted with neutral balata.
Behavioral analysis
All behavioral experiments were conducted without interruption between 9.00 a.m. and 3.00 p.m.
Open field test (OFT)
Mice were placed individually in the center of an open-field (l × w × h: 50 × 50 × 40 cm, divided into 16 square grids; Coulbourn Instruments, Whitehall, USA) in a darkened room and were allowed to move freely. Behavioral parameters were assessed for 5 min using the SMART 3.0 Video Tracking System (SMART 3.0, Panlab, Spain). Specifically, the following parameters were measured: (1) total distance traveled in the open field zone, (2) mean speed in zone-center (cm/s), and (3) number of rearing. The distance traveled reflects the horizontal movement ability of mice, and mean speed in zone-center reflects the anxiety degree of mice, the anxious mice slowed down their mean speed in zone-center. while the number of rearing reflects the vertical movement ability of mice. After each test, the open field apparatus was cleaned using 70% ethyl alcohol and allowed to dry before the next test [Citation25].
Grip strength test
To test the muscle tension in the limbs of the mice, the grip strength test was performed. Briefly, the mouse was placed on a grip plate, and once the it had grasped the grip plate, the tail was lifted and pulled backwards at a constant speed until the mouse released the grip plate. The grip strength testing system (Bioseb, Vitrolles, France) was used to measure the maximum grip strength at the time of paw release. Each mouse was tested three times at 10-min intervals and the average grip strength was used for statistical analysis [Citation26].
Statistical analyses
The data are expressed as mean ± SEM. The Kolmogorov–Smirnov test was used to evaluate the normality of the data. No test for outliers was conducted. Where normality criteria were met, the data were compared by one-way ANOVA followed by Fisher's Least Significant Difference (LSD) or Dunn’s post hoc tests for pairwise comparisons. All statistical analyses were performed using SPSS 19.0 software (SPSS, Inc., Chicago, IL, USA). A p-value < 0.05 (two-tailed) was considered significant for all tests. Analysis no blinding was performed.
Results
Establishment and verification of ET
In the first part of the experiment, induction and validation of peripheral ET was determined. Compared with the control group, after the single dose injection of LPS (1 × LPS group), the protein and mRNA expression of the IL-1β, TNF-α and IL-10 was significantly increased in the SN (all p < 0.05), indicating a brain-specific training effect induced by the first LPS stimulus. Compared with the control group, mice in the 4 × LPS group TNF-α protein and mRNA expression in the SN was not statistically different (both p > .05), IL-1β expression were similar. Protein and mRNA expression of IL-10 remained elevated (both p < .05), thus, indicating that ET was established. These results demonstrated that ET could be induced by repeated low-dose intraperitoneal LPS injection in mice, as shown in .
Figure 2. Repeated low-dose intraperitoneal LPS injection-induced ET. The expression of TNF-α and IL-1β was suppressed, while that of IL-10 was increased by repeated 0.5 mg/kg intraperitoneal LPS injection. (a) The levels of TNF-α, IL-1β, and IL-10 in the SN were detected by ELISA. (b) The expression of TNF-α, IL-1β, and IL-10 in the SN were detected by qRT-PCR. Data are presented as mean ± SEM (n = 3 mice per group). Statistical significance was determined by ANOVA with post hoc LSD tests; *p < .05, **p < .01 versus control group, #p < .05 versus 1 × LPS group.
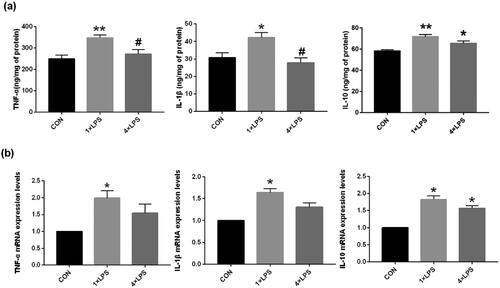
ET pretreatment decreased pro-inflammatory cytokines and increased an anti-inflammatory cytokine induced by peripheral high doses of LPS
To test whether ET preconditioning was able to prevent continuous peripheral high-dose LPS injection-induced neuroinflammation, we first investigated the expression of cytokines (TNF-α, IL-1β, and IL-10) in the SN by ELISA. Compared with the control group, continuous high-dose LPS administration (4 × LPS1 group) caused an increase in the TNF-α and IL-1β expression in the SN (p < .01 and p < .05, respectively). At the same time, the expression of the IL-10 was decreased (p < .01). The changes of cytokines in the 4 × LPS0.5 group were not statistically significant (all p > .05; ). Compared with the 4 × LPS1 group, ET pretreatment significantly decreased the expression of TNF-α (39.63%, p < .01) and IL-1β by (15.86%, p < .05) and increased the expression of IL-10 (19.78%, p < .05) at 28 days postcontinuous high-dose LPS injection ().
Figure 3. ET preconditioning inhibited neuroinflammation induced by continuous high dose LPS injection. (a) The levels of TNF-α, IL-1β, and IL-10 in the SN were detected by ELISA. (b) The levels of TNF-α, IL-1β, and IL-10 in the SN were detected by qRT-PCR. Data are presented as the mean ± SEM (n = 3 mice per group). Statistical significance was determined by ANOVA with post hoc LSD tests or Dunnett's T3 tests; *p < .05, **p < .01 versus control group, #p < .05, ##p < .01 versus 4 × LPS1 group.
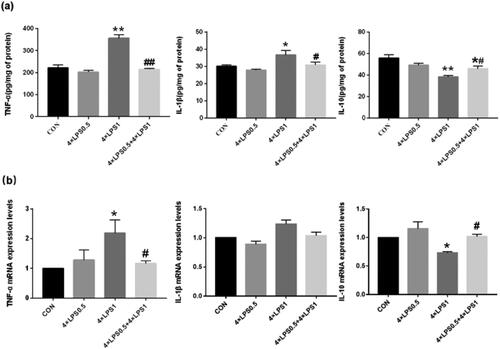
Next, we examined the expression of the cytokines TNF-α, IL-1β, and IL-10 in the SN by qRT-PCR. A significant increase in the mRNA levels of TNF-α was observed in the 4 × LPS1 group compared with the control group (p < .05), while that of IL-1β, although increased, was not statistically significant (p > .05). Expression of IL-10 mRNA was significantly decreased compared with controls (p < .05; ). Compared with the 4 × LPS1 group, the 4 × LPS0.5 + 4 × LPS1 group showed a significant decrease in TNF-α mRNA levels (p < .05), that of IL-1β mRNA was not statistically significant (p > .05), and that of IL-10 mRNA was significantly increased (p < .05; ). Interestingly, the 4 × LPS0.5 group showed no difference in the expression of TNF-α, IL-1β, or IL-10 mRNA compared with the control group (all p < .05; ).
Taken together, the results showed that ET preconditioning attenuates the inflammatory response in the SN.
ET pretreatment inhibited continuous peripheral high-dose LPS injection-induced microglial activation in the SN
To test the effect of ET pretreatment on microglial activation induced by continuous peripheral high-dose LPS injection we first detected the expression of the microglial marker, Iba-1, and the microglial activation marker, CD68, in the SN by western blot. As shown in , compared with the control group, Iba-1 and CD68 in the 4 × LPS1 group were significantly increased (both p < .05), while in the 4 × LPS0.5 group, no statistically significant changes were found (p > .05). Compared with the 4 × LPS1 group, the protein expression levels of Iba-1 and CD68 were decreased in the 4 × LPS0.5 + 4 × LPS1 group (p < .01 and compared with the control group, p > .05, respectively; ).
Figure 4. The protein expression of Iba-1 and CD68 was detected in the SN by western blotting. β-actin protein was used as an internal control. Bar graphs represent the relative expression of Iba1 and CD68 (n = 3 mice per group). (a) Relative expression level of Iba-1. (b) Relative expression level of CD68; Statistical significance was determined by ANOVA with post hoc LSD tests; *p < .05, versus control group, ##p < .01 versus 4 × LPS1 group.
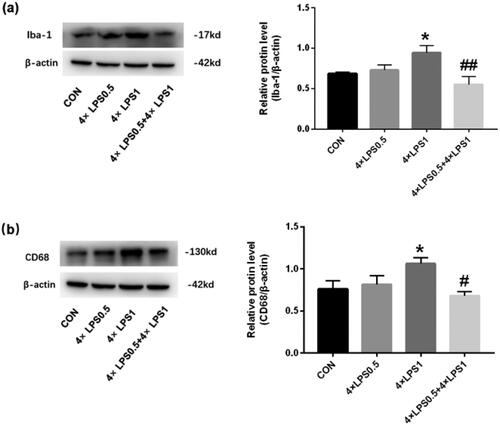
Next, we measured the expression of CD68 in the SN by immunofluorescence and targeted microglia cells with Iba-1. As shown in , compared with the control group, the 4 × LPS1 showed increased CD68 and Iba-1 double positive cell count (p < .01, ), their branches were reduced and cell bodies were enlarged (), while in the 4 × LPS0.5 group, no statistically significant changes (p > .05, ). Compared with the 4 × LPS1 group, decreased CD68 and Iba-1 double positive cell count in the 4 × LPS0.5 + 4 × LPS1 group (p < .01, ), microglia have more branches and are closer to a resting state ().
Figure 5. CD68 and Iba-1 double positive cells were examined by immunofluorescence with quantitative analysis using Image Pro Plus. red: CD68 (a marker of activation of microglia); green: Iba-1(a marker of microglia). The microscopy images were taken with 200 μm of magnification and the squares represent the approximation to 100 μm. (a) control group (b) 4 × LPS0.5 group (c) 4 × LPS1 group (d) 4 × LPS0.5 + 4 × LPS1 group (e) Quantitative results. Data are presented as the mean ± SEM (n = 3 mice per group). Statistical significance was determined by ANOVA with post hoc LSD tests; **p < .01 versus control group, ##p < .01 versus 4 × LPS1 group.
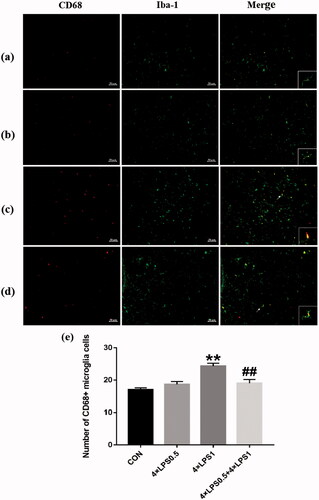
These results indicated that ET pretreatment inhibited the activation of microglia induced by continuous peripheral high-dose LPS injection.
ET pretreatment decreased LPS-induced dopaminergic neuronal injury
TH is the rate-limiting enzyme of DA secretion. The protein expression of TH in the SN was detected by western blotting and its gene expression by qRT-PCR. Results indicated that the TH protein and mRNA levels of mice in the 4 × LPS1 group showed an obvious decrease compared with mice in the control group (both p < .01; ). However, TH protein and mRNA levels in the 4 × LPS0.5 group were not statistically significant compared to the control group (both p > .05; ). Compared with the 4 × LPS1 group, the 4 × LPS0.5 + 4 × LPS1 group showed upregulated TH protein and mRNA levels (p < .05 and p < .01, respectively; ). The results suggest that ET preconditioning can alleviate the decrease of TH expression induced by continuous peripheral high-dose LPS treatment.
Figure 6. Determination of TH and DA in the SN. Data are presented as the mean ± SEM (n = 3 mice per group). (a) TH protein expression was examined by western blotting. (b) TH gene expression was examined by qRT-PCR. (c) DA protein expression was examined by ELISA; Statistical significance was determined by ANOVA with post hoc LSD tests; **p < .01, versus control group, #p < .05, ##p < .01, versus 4 × LPS1 group.
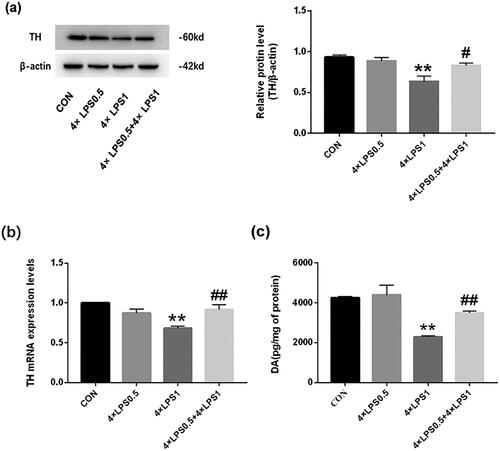
Dyspraxia in PD is caused by a decrease in DA production in the striatum [Citation18]. To test the effect ET pretreatment on the reduction of DA secretion induced by continuous peripheral high doses of LPS, the content of DA in the striatum was determined by ELISA. Results showed that, compared with the control group, DA secretion in the 4 × LPS1 group was decreased (p < .01; ), whereas there was no statistical change in the 4 × LPS0.5 group (p > .05; ). Compared with the 4 × LPS1 group, the content of DA in the 4 × LPS0.5 + 4 × LPS1 group increased (p < .01; ). The results suggest that ET preconditioning can alleviate the decrease in DA secretion induced by continuous peripheral high dose LPS treatment.
These results underlined that ET pretreatment can prevent dopaminergic neuronal injury induced by a continuous peripheral high dose of LPS.
Effect of ET on LPS-induced morphologic alterations in neurons of the SN
The death of dopaminergic neurons is a hallmark of PD. TH is the golden marker for dopaminergic neurons in the SN. The effect of ET on LPS-induced morphologic alterations in dopaminergic neurons of the SN was assessed by HE staining and immunofluorescence analysis. HE staining indicated that dopaminergic neurons were regularly arranged and clearly visible both in the control group and the 4 × LPS0.5 group, however, after a continuous peripheral high dose of LPS, degeneration became evident. Specifically, neurons of the SN of mice in the 4 × LPS1 group were disorderly and the chromatin became shallow, nucleus pyknosis, and microglial infiltration into neurons was pronounced. As shown in , the yellow arrow highlights nuclear pyknosis and microglial infiltration. Compared with the 4 × LPS1 group, in the 4 × LPS0.5 + 4 × LPS1 group cell boundaries were clear and the neurons arranged more regularly ().
Figure 7. Effect of ET on LPS-induced neuronal morphological alterations in the SN. Sections were stained with HE (magnification 400×, scale bar: 20 μm).
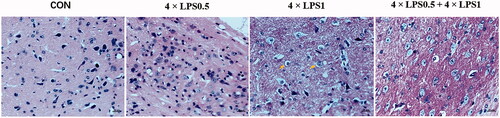
Immunofluorescence analysis showed that, compared with the control group, the number of TH positive cells decreased in 4 × LPS1 group (p < .01; ), microglia infiltrate around dopaminergic neurons, the activation of microglia was obvious (), Compared with the 4 × LPS1 group, number of TH-positive cells increased in the 4 × LPS0.5 + 4 × LPS1 group (p < .01; ), microglia activation is inhibited ().
Figure 8. ET inhibited high doses of LPS-induced dopaminergic neuronal death. immunofluorescence double staining of SN for TH and Iba-1. red - TH; green -Iba-1 (n = 3 per group). The ipsilateral sides are shown. The microscopy images were taken with 200 μm of magnification and the squares represent the approximation to 100 μm. (a) control group (b) 4 × LPS0.5 group (c) 4 × LPS1 group (d) 4 × LPS0.5 + 4 × LPS1 group (e) Representative quantification of TH. Values represent mean ± SD. Statistical significance was determined by ANOVA with post hoc LSD tests, *p < .05, **p < .01 versus control group; ##p < .01 versus 4 × LPS1 group.
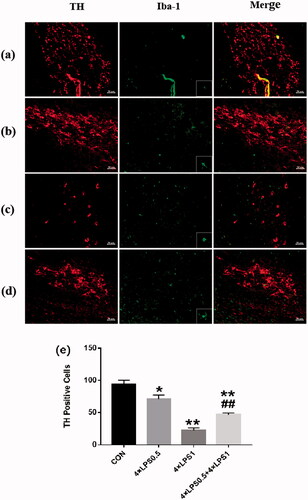
Our results suggest that high doses of LPS promote neuronal degeneration, pretreatment with ET attenuated neuronal changes in the SN.
ET pretreatment improved continuous peripheral high-dose LPS injection-induced behavioral impairment
PD is a progressive neurodegenerative disease primarily characterized by motor symptoms such as bradykinesia, rigidity, postural instability, and tremors [Citation8]. In order to test the protective effect of ET on LPS-induced behavioral disorders in mice, the OFT and muscle tension tests were conducted. OFT analysis found that, compared with the control group, the total distance traveled, rearing frequency and mean speed in zone-center by the 4 × LPS1 group was significantly decreased (all p < .05; 9a-c), whereas no significant difference was found in the 4 × LPS0.5 group (all p > .05; ). These results indicated that continuous high-dose injection of LPS could lead to horizontal dyskinesia, vertical motion disorder and anxiety in mice. Compared with the 4 × LPS1 group, the total distance traveled, rearing frequency and mean speed in zone-center by the 4 × LPS0.5 + 4 × LPS1 group was increased (p < .05, p < .05, compared with the control group, p > .05, respectively; ), There was no statistical difference compared with the control group (all p > .05; ). These results indicate that ET pretreatment improved continuous peripheral high dose LPS injection-induced behavioral impairment. Finally, there was no significant difference in grip strength among the groups (all p > .05; ).
Figure 9. Continuous peripheral high doses of LPS reduced movement in mice. There was no significant change in muscle tension. ET preconditioning can alleviate movement disorders. (a) Total distance traveled in the open field zone. (b) mean speed in zone-center (cm/s). (c) Number of rearing. (d) Grip strength. Data are presented as the mean ± SEM of 6–10 mice per group; Statistical significance was determined by ANOVA with post hoc LSD tests; *p < .05, versus control group, #p < .05, versus 4 × LPS1 group.
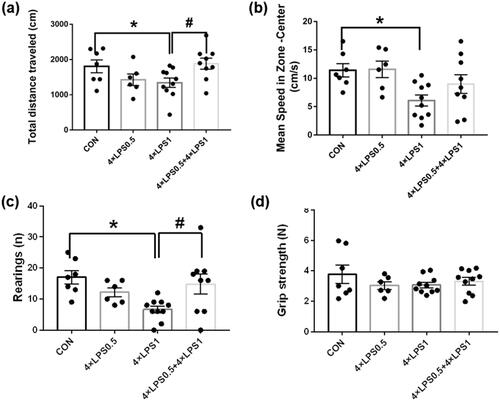
Discussion
Neuroinflammation has been established as a crucial mechanism in the development and progression of neurodegenerative diseases [Citation27]. Postmortem analyses of human PD patients and experimental animal studies indicate that activation of microglia and increases in pro-inflammatory factor levels are common features of the PD brain [Citation28,Citation29]. Targeting of inflammatory processes has been suggested as promising interventional therapies for PD and other neurodegenerative diseases [Citation5].
Immune tolerance is a protective mechanism against excessive inflammation and secondary injury. Recent studies show, when an animal is in a state of immune tolerance, it can increase the expression of genes that protect the animal from systemic inflammation. The possible molecular mechanisms mainly involve LPS signal changes, epigenetic markers, chromatin remodeling, etc. These may be the root cause of the formation of immune memory and LPS-resistant physiological changes [Citation30]. ET is induced by a low dose of LPS and deemed as the most essential component of immune tolerance when suffering from subsequent inflammatory challenge [Citation19,Citation21]. Here, we explored the establishment of ET induced by low-dose LPS. Besides the dose, the animal models used and days of pretreatment are important influencing factors for the establishment of immune tolerance, which has been confirmed by several studies [Citation31]. In the current study, an ET model in mice was established by intraperitoneal injection of 0.5 mg/kg LPS for four consecutive days according to the method of Wendeln [Citation24]. The effect of the model construction was also evaluated. Our results indicated that the above approach induced ET, as characterized by a decreased expression of inflammatory cytokines in the SN. Indicated that the peripheral immune tolerance induced a hypoactive state. This suggests that peripheral continuous low dose LPS injection can successfully induce ET in the central nervous system.
In this study, we investigated the role of peripheral ET in the occurrence and progress of subsequent continuous high dose LPS peripheral injection-induced neuroinflammation. Our results showed that ET preconditioning attenuated the continuous high-dose LPS injection-induced neuroinflammation, manifested as a decrease in pro-inflammatory cytokine expression.
Microglia are thought to be involved in the occurrence and aggravation of neuroinflammation. They are an important part of the brain immune system and play a crucial role in maintaining the dynamic balance of the brain microenvironment [Citation32]. Microglia are usually activated following neuroinflammation, and once activated, they change from a dormant state to an active state by changing morphology to produce pro-inflammatory cytokines and chemotactic factors, which further induce neurotoxic mediators such as nitric oxide, prostaglandin E2, and reactive oxygen species [Citation33]. This inflammation-induced excessive activation of microglia may also disrupt the brain's microenvironment, which further leads to neurotoxicity such as oxidative stress and neuronal apoptosis [Citation27]. In addition, microglia can also injure neurons through synaptic and neuronal phagocytosis [Citation34].
Pretreatment with fluoxetine or somatostatin has been shown to exert neuroprotective effects in rodent models that were the result of inhibition of neuroinflammation-related microglial activation [Citation35,Citation36]. Our present study showed that LPS preconditioning increased DA content and improved behaviors, which were accompanied by inhibition of microglial activation. These results highlight the neuroprotective role of microglial immunocompetent restriction in neuroinflammation -induced neurodegenerative diseases.
Taken together, our study provides evidence that peripheral immune tolerance preconditioning plays a crucial role in neuroprotection from subsequent neuroinflammation-induced neurodegeneration, and that inhibition of microglial activation may be an important factor associated with neuroprotection.
The main purpose of this study was to explore the potential value of ET as a protection strategy. Although peripheral high-dose LPS (5 mg/kg) injection induced an inflammatory effect in the SN, as demonstrated by the increase of inflammatory cytokines and microglial activation [Citation6], the dose of LPS that we used for pretreatment in the present study was considered to be a low-dose (0.5 mg/kg). The results indicated that repeated low-dose intraperitoneal LPS injection was insufficient to cause inflammation within the SN of healthy mice. Furthermore, and importantly, this dose did not decrease TH or DA secretion or pathological changes to dopaminergic neurons, and indicates that ET pretreatment is safe.
It is worth noting that LPS at low to medium doses is not able to cross the blood-brain barrier and enter brain tissue [Citation37]. Indeed, studies have indicated that, although repeated doses of LPS greatly downregulate body responses to LPS, brain responses are less evident [Citation24] and suggest that peripheral LPS may induce or inhibit neuroinflammation through peripheral pathway. Specifically, inflammatory suppression of immune tolerance may begin before neuroinflammation occurs, therefore, the protective effect against neurodegeneration may be greater than that detected in the current study. Future experiments will be needed to determine if this assumption is correct. Increasing evidence has indicated that the adaptive immune response and innate immunity are important mediators of neuroinflammation- mediated dopaminergic neurodegeneration, therefore, addressing central nervous system dysfunction through immune control aimed at downregulating the immune response is a promising future treatment option [Citation38].
Conclusions
Peripheral immune tolerance is an important regulatory mechanism for immunological activity. Our results indicated that peripheral ET attenuated neuroinflammation from the periphery and provided neuroprotection against neuroinflammation and subsequent neurodegeneration. This approach may offer a new therapeutic strategy to combat neurodegenerative diseases.
Ethical approval
All applicable international, national, and/or institutional guidelines for the care and use of animals were followed.
Abbreviations | ||
AD | = | Alzheimer's disease |
ANOVA | = | Analysis of variance |
BCA | = | bicinchoninic acid |
DA | = | Dopaminergic |
DAPI | = | 4,6-diamidino-2-phenylindole |
ELISA | = | enzyme-linked immunosorbent assay |
ET | = | Endotoxin tolerance |
HE | = | Hematoxylin and eosin |
Iba-1 | = | ionized calcium binding adapter molecule 1 |
IL-1β | = | Interleukin-1β |
LSD | = | Least Significant Difference |
LPS | = | lipopolysaccharide |
MCAO | = | middle cerebral artery occlusion syndrome |
OFT | = | Open field test |
PBS | = | phosphate buffer saline |
PD | = | Parkinson's disease |
qRT-PCR | = | quantitative real-time polymerase chain reaction |
SN | = | substantia nigra |
TBST | = | Tris buffered saline Tween |
TH | = | Tyrosine Hydroxylase |
TNF-α | = | tumor necrosis factor-alpha |
Disclosure statement
The authors declare no conflicts of interest.
Additional information
Funding
References
- Gigante AF, Bruno G, Iliceto G, et al. Action tremor in parkinson's disease: frequency and relationship to motor and non-motor signs. Eur J Neurol. 2015;22(2):223–228.
- Heneka MT, Carson MJ, Khoury JE, et al. Neuroinflammation in alzheimer's disease. Lancet Neurol. 2015;14(4):388–405.
- Wee Yong V. Inflammation in neurological disorders: a help or a hindrance? Neuroscientist. 2010;16(4):408–420.
- Liu Y, Xie X, Xia LP, et al. Peripheral immune tolerance alleviates the intracranial lipopolysaccharide injection-induced neuroinflammation and protects the dopaminergic neurons from neuroinflammation-related neurotoxicity. J Neuroinflammation. 2017;14(1):223.
- Wang Q, Liu Y, Zhou J. Neuroinflammation in parkinson's disease and its potential as therapeutic target. Transl Neurodegener. 2015;4:19.
- Qin L, Wu X, Block ML, et al. Systemic LPS causes chronic neuroinflammation and progressive neurodegeneration. Glia. 2007;55(5):453–462.
- Qin L, Liu Y, Hong JS, et al. NADPH oxidase and aging drive microglial activation, oxidative stress, and dopaminergic neurodegeneration following systemic LPS administration. Glia. 2013;61(6):855–868.
- Tufekci KU, Genc S, Genc K. The endotoxin-induced neuroinflammation model of parkinson's disease. Parkinsons Dis. 2011;2011:487450.
- Zhang FX, Xu RS. Juglanin ameliorates LPS-induced neuroinflammation in animal models of parkinson's disease and cell culture via inactivating TLR4/NF-κB pathway. Biomed Pharmacother. 2018;97:1011–1019.
- Bodea LG, Wang Y, Linnartz-Gerlach B, et al. Neurodegeneration by activation of the microglial complement-phagosome pathway. J Neurosci. 2014;34(25):8546–8556.
- Beier EE, Neal M, Alam G, et al. Alternative microglial activation is associated with cessation of progressive dopamine neuron loss in mice systemically administered lipopolysaccharide. Neurobiol Dis. 2017;108:115–127.
- Tweedie D, Luo W, Short RG, et al. A cellular model of inflammation for identifying TNF-alpha synthesis inhibitors. J Neurosci Methods. 2009;183(2):182–187.
- Yu Q, Song FJ, Chen JF, et al. Antineuroinflammatory effects of modified Wu-Zi-Yan-Zong prescription in β-amyloid-stimulated BV2 microglia via the NF-κB and ERK/p38 MAPK signaling pathways. Evid Based Complement Alternat Med. 2017;2017:8470381.
- Gao X, Chen H, Schwarzschild MA, et al. Use of ibuprofen and risk of Parkinson disease. Neurology. 2011;76(10):863–869.
- Hancock DB, Martin ER, Stajich JM, et al. Smoking, caffeine, and nonsteroidal anti-inflammatory drugs in families with Parkinson disease. Arch Neurol. 2007;64(4):576–580.
- Hernán MA, Logroscino G, García Rodríguez LA. Garcı ’a rodrı ’guez, LA. Nonsteroidal anti-inflammatory drugs and the incidence of Parkinson disease. Neurology. 2006;66(7):1097–1099.
- Woodling NS, Andreasson KI. Untangling the web: Toxic and protective effects of neuroinflammation and PGE2 signaling in Alzheimer's disease. ACS Chem Neurosci. 2016;7(4):454–463.
- Stojkovska I, Wagner BM, Morrison BE. Parkinson's disease and enhanced inflammatory response. Exp Biol Med. 2015;240(11):1387–1395.
- Biswas SK, Lopez-Collazo E. Endotoxin tolerance: new mechanisms, molecules and clinical significance. Trends Immunol. 2009;30(10):475–487.
- Kox M, de Kleijn S, Pompe JC, et al. Differential ex vivo and in vivo endotoxin tolerance kinetics following human endotoxemia. Crit Care Med. 2011;39(8):1866–1870.
- Martin M, Katz J, Vogel SN, et al. Differential induction of endotoxin tolerance by lipopolysaccharides derived from Porphyromonas gingivalis and Escherichia coli. J Immunol. 2001;167(9):5278–5285.
- Rosenzweig HL, Lessov NS, Henshall DC, et al. Endotoxin preconditioning prevents cellular inflammatory response during ischemic neuroprotection in mice. Stroke. 2004;35(11):2576–2581.
- Longhi L, Gesuete R, Perego C, et al. Long-lasting protection in brain trauma by endotoxin preconditioning. J Cereb Blood Flow Metab. 2011;31(9):1919–1929.
- Wendeln AC, Degenhardt K, Kaurani L, et al. Innate immune memory in the brain shapes neurological disease hallmarks. Nature. 2018;556(7701):332–338.
- Krishna S, Lin Z, de La Serre CB, et al. Time-dependent behavioral, neurochemical, and metabolic dysregulation in female C57BL/6 mice caused by chronic high-fat diet intake. Physiol Behav. 2016;157:196–208.
- Krishna S, Dodd CA, Hekmatyar SK, et al. Brain deposition and neurotoxicity of manganese in adult mice exposed via the drinking water. Arch Toxicol. 2014;88(1):47–64.
- Zhou J, Deng Y, Li F, et al. Icariside II attenuates lipopolysaccharide-induced neuroinflammation through inhibiting TLR4/MyD88/NF-κB pathway in rats. Biomed Pharmacother. 2019;111:315–324.
- McGeer PL, Itagaki S, Boyes BE, et al. Reactive microglia are positive for HLA-DR in the substantia nigra of Parkinson's and Alzheimer's disease brains. Neurology. 1988;38(8):1285–1291.
- Taylor JM, Main BS, Crack PJ. Neuroinflammation and oxidative stress: co-conspirators in the pathology of Parkinson's disease. Neurochem Int. 2013;62(5):803–819.
- Seeley JJ, Ghosh S. Molecular mechanisms of innate memory and tolerance to LPS. J Leukoc Biol. 2017;101(1):107–119.
- Qin XY, Zhang SP, Cao C, et al. Aberrations in peripheral inflammatory cytokine levels in Parkinson disease: a systematic review and Meta-analysis. JAMA Neurol. 2016;73(11):1316–1324.
- Colonna M, Butovsky O. Microglia function in the Central nervous system during health and neurodegeneration. Annu Rev Immunol. 2017;35:441–468.
- Luo X-G, Chen S-D. The changing phenotype of microglia from homeostasis to disease. Transl Neurodegener. 2012;1(1):9.
- Xin YR, Jiang JX, Hu Y, et al. The immune system drives synapse loss during lipopolysaccharide-induced learning and memory impairment in mice. Front Aging Neurosci. 2019;11(279):279.
- Chung ES, Chung YC, Bok E, et al. Fluoxetine prevents LPS-induced degeneration of nigral dopaminergic neurons by inhibiting microglia-mediated oxidative stress. Brain Res. 2010;1363:143–150.
- Bai L, Zhang X, Li X, et al. Somatostatin prevents lipopolysaccharide-induced neurodegeneration in the rat substantia nigra by inhibiting the activation of microglia. Mol Med Rep. 2015;12(1):1002–1008.
- Banks WA, Robinson SM. Minimal penetration of lipopolysaccharide across the murine blood-brain barrier. Brain Behav Immun. 2010;24(1):102–109.
- Brown GC. The endotoxin hypothesis of neurodegeneration. J Neuroinflammation. 2019; 16(1):180.