Abstract
Objective
Sepsis is one of major reasons of cardiorenal syndrome type 5 (CRS-5), resulting in irreversible tissue damage and organ dysfunction. Macrophage has been demonstrated to play key role in the pathophysiology of sepsis, highlighting the need to identify therapeutic targets for modulating macrophage phenotype in sepsis.
Methods and Results
In this study, a rapid-releasing hydrogen sulfide (H2S) donor NaSH, and a slow-releasing H2S compound S-propargyl-cysteine (SPRC) which is derived from garlic, have been studied for the immune-regulatory effects on macrophages. The NaSH and SPRC showed the potential to protect the heart and kidney from tissue injury induced by LPS. The immunohistochemistry of F4/80+ revealed that the infiltration of macrophages in the heart and kidney tissues of LPS-treated mice was reduced by NaSH and SPRC. In addition, in the LPS-triggered inflammatory cascade of RAW264.7 macrophage cells, NaSH and SPRC exhibited significantly inhibitory effects on the secretion of inflammatory cytokines, production of reactive oxygen species (ROS), and regulation of the macrophage phenotype from M1-like to M2-like. Moreover, autophagy, a crucial process involved in the elimination of impaired proteins and organelles during oxidative stress and immune response, was induced by NaSH and SPRC in the presence of LPS stimulation. Consequently, there was an increase in the number of mitochondria and an improvement in mitochondrial membrane potential. This process was mainly mediated by PINK1/Parkin pathway mediated mitophagy.
Discussion
These results demonstrated that the immunoregulatory effects of H2S donors were through the PINK1/Parkin-mediated mitophagy pathway. Overall, our study provided a new therapeutic direction in LPS-induced cardiorenal injury.
Introduction
Cardiorenal syndrome type 5 (CRS-5) is one of the multi-organ dysfunctions caused by septic injury. Sepsis is one of the most common causes of acute cardiac dysfunction and acute kidney injury (AKI), which causes high mortality worldwide [Citation1]. The onset of sepsis is triggered by pathogens accompanying a dysregulated inflammatory response, subsequently resulting in immune disorder and immunosuppression [Citation2]. The microbial component lipopolysaccharide (LPS) is the main cause of sepsis, which recognizes Toll-like receptor 4 (TLR4) to activate the transcription of inflammatory and immune response genes. Sustained activation of neutrophils, macrophages, and lymphocytes leads to the generation of pro-inflammatory mediators, including tumor necrosis factor-α (TNF-α), interleukin (IL)-6, IL-1β, and IL-8, as well as reactive oxygen species (ROS), thereby intensifying the inflammatory response. Consequently, the persistent inflammation would cause tissue damage and organ dysfunction [Citation3].
Macrophages exhibit diverse functions by altering phenotype in response to different immune situation and inflammatory disorders [Citation4]. The main two distinct subsets of macrophages are the classically activated M1 phenotype and alternatively activated M2 phenotype, depending on the surrounding microenvironment. Macrophages responding to mediators such as interferon γ (IFNγ), TNF-α, or sub-stimulatory concentrations of LPS is termed as M1 phenotypic state, would effectively in killing bacteria but associated with the generation of pro-inflammatory cytokines, organ injury, and tissue damage. The M2 phenotype is programmed by IL-4 or IL-13, which is associated with tissue repair and secretion of anti-inflammatory cytokines, such as IL-10 and transforming growth factor (TGF)-β [Citation5]. The dysregulated polarization of macrophages in sepsis leads to a pro-inflammatory disorder, resulting in organ injuries such as AKI and cardiac dysfunction. Balance the macrophage phenotypic change could be an effective approach in the treatment of sepsis-induced tissue damage [Citation6].
Autophagy maintains the cellular homeostasis by recycling the misfolded proteins, protein aggregates, and dysfunctional or senescent organelles [Citation7]. Under pathological stress, such as inflammation induced by sepsis, the overproduction of ROS depolarizes the mitochondria membrane potential, leading to mitochondrial injury and the initiation of subsequent cell death signaling pathways [Citation8]. Mitophagy, a selective form of autophagy to eliminate dysfunctional or damaged mitochondria, plays an important role in mitochondrial quality control [Citation9,Citation10]. These damaged mitochondria are recognized and engulfed by autophagosomes, and targeted for degradation by lysosomes. The PTEN-induced kinase 1 (PINK1)-parkin RBR E3 ubiquitin protein ligase (PARK2) pathway has been recognized as one of the most important way involved in mitophagy [Citation11]. Other pathways, such as BCL2-interacting protein 3 (BNIP3) [Citation12] and FUN14 Domain Containing 1 (FUNDC1) [Citation13], are also being reported.
Hydrogen sulfide (H2S) is a gaseous signaling molecule in regulating cellular physiology and pathophysiology. The immune-regulatory role of H2S has been reported, especially in relation to inflammatory responses [Citation14]. Three key enzymes contribute to H2S biosynthesis, including cystathionine γ-lyase (CSE), cystathionine β-synthase (CBS), and 3-mercaptopyruvate sulfur-transferase (3-MPST). Garlic (Allium sativum) has been reported as a natural H2S donor in pharmacological studies, including anti-tumor, anti-inflammation, osteoarthritis, acute lung injury, etc. [Citation15]. The major components of garlic are cysteine-derived organosulfur compounds (OSCs), including the lipid-soluble allyl sulfur compounds, diallyl sulfide (DAS), diallyl disulfide (DADS) and diallyl trisulfide (DATS) and the water-soluble compounds, including S-allyl-L-cysteine (SAC) and S-allylmercaptocysteine (SAMC) [Citation16]. The DAS, DADS, DATS and SAC could increase the autophagosome formation in macrophages [Citation17]. We have demonstrated that SAMC can suppress colon cancer growth through autophagic pathway in combination with rapamycin [Citation18]. S-propargyl-cysteine (SPRC), a sulfur-containing amino acid derivative from SAC, has been reported exhibit more efficient effect in modulating endogenous H2S level by activating CSE expression [Citation19].
The macrophage phenotype change is a conspicuous consequence of sepsis, but the effect of H2S on inflammation remains controversial, and the mechanism is still not clear. This study aims to investigate the involvement of rapid-releasing H2S (NaSH) and chronic-releasing H2S (SPRC) donors in sepsis. Furthermore, the potential role of PINK1-mediated mitophagy in macrophage functions has been discussed.
Materials and methods
Drugs and chemicals
LPS (Escherichia coli O111:B4, L4391), sodium hydrogen sulfide (NaHS, 161527) and chloroquine (CQ, C6628) were obtained from Sigma-Aldrich (Merck KGaA, Darmstadt, Germany). Reagents were dissolved in PBS and prepared 30 min before use. L-cysteine (98%, IC1370) was purchased from Solarbio (Beijing, China). Propyl bromide (B0638, TCI Shanghai) and propargyl bromide (P106961, Aladdin, Shanghai, China) were reacted with L-cysteine to synthesize SPRC, and purified by re-crystallization from an ethanol-water mixture as previously reported [Citation20,Citation21].
Cell culture
RAW264.7 murine macrophage-like cells were obtained from the National Collection of Authenticated Cell Cultures (Shanghai, China; ref. no. SCSP-5036). The cells were cultured in Dulbecco’s modified Eagle’s medium (Gibco; Thermo Fisher Scientific, Inc., Waltham, MA, USA) supplemented with 10% fetal bovine serum (Gibco) and 1% (v/v) penicillin/streptomycin at 37 °C in a humidified atmosphere of 5% CO2. For experiments, cells were seeded in 96-well plates or six-well plates (Costar, Cambridge, MA), after serum starved for 6 h, cells were incubated with LPS (1 μg/mL) plus NaSH or SPRC (1 or 10 μmol/L) for another 24 h.
Cell proliferation assay by CCK-8
The Cell Counting Kit-8 (CCK-8) assay kit (C0005) was obtained from Topscience Co. Ltd. The cells in logarithmic phase were seeded in 96-well plate for 16 h. After serum-starved for 6 h, 1 μg/mL LPS with or without NaSH (0.1, 1, 10 μM) and SPRC (0.1, 1, 10 μM) were added to each well and incubated for 24 h. The cell viability was measured at 450 nm of optical density (OD) using a microplate reader (Bio-Rad Instruments, Hercules, CA, USA). The cell viability = (Treatment Group OD − Blank Group OD)/(Control Group OD − Blank Group OD).
Macrophage phagocytosis assay
Macrophages RAW264.7 were seeded in 96-well plates for 1 × 105 cells per well. After LPS (1 μg/mL), and NaSH (1 μM), SPRC (1 μM) treatment for 24 h respectively, the cells were incubated with Neutral Red (C0013, Beyotime Biotechnology) at 37 °C for 2 h. The cells were washed with PBS for three times, then incubated with lysis buffer for 10 min on a shaker at room temperature. Finally, the OD value at 540 nm was determined using a plate reader.
RNA extraction and real-time polymerase chain reaction (RT-PCR)
Total cellular RNA was isolated using TRIzol (Invitrogen), following cDNA synthesis with a FirstStrand cDNA Synthesis Kit (Invitrogen). Quantitative real-time PCR was performed with SYBR Green Realtime PCR Master Mix (QPK-201, TOYOBO CO., LTD.), using CFX96 Touch Real-Time PCR Detection System (Bio-Rad Laboratories, Inc.). Primers used were as follows: TNF-α: forward 5′-AGGCTCTGGAGAACAGCACAT-3′, reverse 5′-TGG CTTCTCTTCCTGCACCAAA-3′; Nitric oxide synthase 2 ((Nos2): forward: 5′-GAGCCCGGAGCCTTTAGAC-3′, reverse: 5′-GCTGAACGAGGAGGGTGGT-3′; C-X-C motif chemokine ligand 10 (Cxcl10): forward: 5′-GAAGCCTCCCCATCAGCA-3′, reverse: 5′-TCGTGGCAATGATCTCAACAC-3′; Interleukin-6 (Il-6) forward: 5′-TACCACTTCACAAGTCGGAGGCTTA-3′, reverse: 5′-CTGCAAGTGCATCATCGTTGTTCAT-3′; Mannose receptor C-Type 1 (Mrc1): forward 5′-TGGAATCAAGGGCACAGAAGT-3′, reverse 5′-CTCCATTTGCATTGCCCAGT-3′; CD206: forward 5′-CTCTGTTCAGCTATTGGACGC-3′, reverse: 5′-CGGAATTTCTGGGATTCAGCTTC-3′; Transforming growth factor beta-1 (Tgfβ1): forward 5′-TAATGGTGGACCGCAACAAC-3′, reverse: 5′-CTTCCCGAATGTCTGACGTATT-3′; Interleukin 10 (Il-10): forward 5′-GCC TTGCAGAAAAGAGAGCT-3′, reverse 5′-AAAGAAAGTCTTCACCTGGC-3′; Glyceraldehyde-3-phosphate dehydrogenase (Gapdh): forward 5′-AGGCCGGTGCTGAGTATGTC-3′, reverse 5′-TGCCTGCTTCACCTTCT-3′. The relative expression of target gene mRNA was normalized to the mRNA level of Gapdh using the 2-ΔΔCt calculating method.
The intracellular ROS measurement
After being stimulated for 24 h, RAW264.7 cells were washed with PBS, then incubated with 10 μM 2,7-dichlorodihydrofluorescein diacetate (H2DCFDA) (D399, Thermo Fisher Scientific Inc.) at 37 °C for 30 min. The fluorescence intensity was tested by a Multi-Mode Microplate Reader (Synergy™ 2, BioTek Instruments, Inc.) using an excitation wavelength of 488 nm and emission wavelength of 525 nm. Intracellular ROS production was also visualized by a Fluorescence Motorized Phase Contrast Microscope (Olympus IX81, Olympus, Inc.).
Mitochondrial function assays
In order to investigate the intensity of mitochondria, the cells were incubated with 150 nM Mito-Tracker Green (C1048, Beyotime) at 37 °C for 30 min. Mitochondrial Membrane Potential (ΔΨm) was assayed by 50 nmol/L tetramethylrhodamine ethyl ester (TMRE, C2001S, Beyotime) for 15 min. Fluorescence images of mitotracker and TMRE were obtained using DeltaVision ultrahigh-resolution microscope (GE Healthcare, Issaquah, WA, USA). The average fluorescent intensity was read by a multifunction microplate reader using an excitation wavelength of 590 nm and an emission wavelength of 635 nm. Images were analyzed using ImageJ software (version 1.41, National Institutes of Health).
Immunofluorescence assay
RAW264.7 cells were plated on glass coverslips in 24-well plates. Cells were treated with LPS (1 μg/mL) with or without NaSH or SPRC for 24 h. After treatment, cells were fixed with 4% paraformaldehyde for 10 min, and permeabilized with 0.1% Triton X-100 for 15 min. Cells then were blocked with 10% goat serum for 1 h at RT, and stained with anti-LC3 (L8918, Sigma-Aldrich) antibody overnight at 4 °C. The autophagosome marker LC3 was visualized by incubating with Alexa Fluor594 (red) conjugated secondary antibodies (A32740, ThermoFisher) for 2 h at room temperature. The coverslips were mounted with antifading mounting medium with DAPI (S2110, Solarbio). Cells were visualized through DeltaVision ultrahigh-resolution microscope. DAPI and Fluor 594 (Red) fluorescence was excited under 405 nm, and 561 nm laser excitation, respectively.
Enzyme-linked immunosorbent assay (ELISA) assay
The concentrations of TNF-α (CSB-E04741m) and IL-6 (CSB-E04639m) in the cellular supernatants were measured using ELISA kits purchased from Cusabio Biotech Co., Ltd (Wuhan China). Serum troponin T (TnT) was measured by using Mouse Troponin T ELISA Kit (SBJ-M0066, NanJing SenBeiJia Biotechnology Co., LTD.)
Animal model of LPS-induced acute cardiorenal injury
Male C57BL/6J mice weighted 18–20 g were purchased from Beijing Vital River Laboratory Animal Technology Co., Ltd. All animal studies were performed in accordance with the institutional guidelines of the Animal Care and Use Committee of Shandong University (Approval No. ECSBMSSDU2018-2-025). Mice were housed in a room with a 12:12h light-dark cycle, with room temperature maintained at 25 ± 2 °C with the relative humidity of 40%-50%, and were free access to the standard commercial diet and water. After one week’ acclimatization to the laboratory conditions, the mice were randomly divided into 4 groups (n = 8) as follows: Vehicle group, LPS (5 mg/kg) group, LPS + NaSH (6 mg/kg) group, LPS + SPRC (10 mg/kg) group. Mice received NaHS and SPRC daily for 7 days with i.p. injection. The dosage of NaSH and SPRC were chosen as previous reported [Citation22,Citation23]. One hour after the last injection of NaSH and SPRC, mice were challenged with LPS (5 mg/kg, i.p.) and sacrificed 6 h later. Normal saline was used as vehicle control.
At the end of the experiments, mice were sacrificed using isoflurane and cervical dislocation. Blood samples were obtained from the inferior vena cava and collected in serum tubes and stored at −80 °C until used. Coronal sections of the kidneys and hearts were fixed in 10% formalin and then embedded in paraffin for histological evaluation. The remainder of the kidney and heart were snap-frozen in liquid nitrogen and stored at −80 °C for further analysis.
Oxidative stress markers
Kidney and heart tissue homogenate samples were used to determine tissue levels of reduced glutathione (GSH) (S0052), malondialdehyde (MDA, S0131M), superoxide dismutase (SOD, S0101M) activity and catalase (CAT, S0051) were determined using commercial kits from Beyotime Biotechnology (China). All procedures were performed according to the manufacturer’s instructions.
Biochemical analysis
Plasma samples were used for the measurement of blood urea nitrogen (BUN, C013-2-1) and creatinine (Cr, C011-2-1). Kits for measurement of BUN and Cr were purchased from Jiancheng Bioengineering Institute (Nanjing, China). H2S level was analyzed by micro H2S content assay kit (BC2055, Solarbio).
Histological analysis and immunohistochemistry
The transverse section of mice’s heart and kidney tissues were harvested and fixed with 4% paraformaldehyde for at least 24 h. After embedded with paraffin, 5-μm thick sections were cut, and then processed for Hematoxylin and Eosin (H&E) staining.
The immunohistochemistry was performed to examine the macrophage infiltration following a DAB method. Briefly, the paraffin sections were processed under antigen retrieval, anti-F4/80 (CI:A3-1, GTX26640, GeneTex, Inc.) incubation, DAB developing and hematoxylin counterstaining. The histological changes of heart and kidney were evaluated under a light microscope (Olympus, Tokyo, Japan).
Macrophage transfection
SiRNA duplexes were synthesized and purified by GenePharma Co., Ltd (Shanghai, China). RAW264.7 macrophage cells were transfected with PINK1 siRNA (5′-CCAAGCGCGTGTCTGACCC-3′) or scramble control siRNA (5′-AGCCGTCGACTGCACTCGC3′) using Lipofectamine 3000 (L3000001, Thermo Fisher Scientific, Waltham, MA, USA) according to the manufacturer’s protocol. The cells were placed in an incubator, and fresh serum-containing medium was replaced 6 h later. After 72 h, the transfection efficiency was detected to examine the mRNA and protein expression respectively.
Western blot
Total protein was prepared using RIPA lysis buffer containing protease and phosphatase inhibitors. Mitochondrial fraction was isolated using a mitochondrial extraction kit (SM0020, Solarbio, Beijing, China) according to manufacturer instructions. The protein extracts were separated by 10–12% SDS-PAGE electrophoresis and transferred onto PVDF membranes (IPVH00010, Millipore, MA, USA). The membranes were incubated with following primary antibodies overnight at 4 °C: anti-LC3 (L8918, Sigma-Aldrich), anti-SQSTM1/p62 (ab91526, Abcam), anti-Beclin 1 (ab62557, Abcam), anti-PINK1 (ab23707, Abcam), anti-Parkin (ab77924, Abcam).
Followed by incubating with secondary antibodies for 1 h at room temperature, the blots were detected using Pierce™ ECL Plus Western Blotting Substrate (32132, ThermoFisher Scientific) with Tanon Immunoassay System (Tanon, China). Relative signal intensity of bands was analyzed by Image J software. Protein expression levels were standardized by normalizing to GAPDH or β-actin.
Statistical analysis
Data were collected from each group and were expressed as Mean ± standard deviation (SD). Comparisons among three or more groups were performed by one-way analysis of variance (ANOVA) followed by Tukey’s test. All statistical analyses were performed using Prism 8 (GraphPad Software Inc, San Diego, CA, USA). A P-value < 0.05 was considered to be statistically significant.
Results
Effects of H2S donors on morphological and functional changes of heart and kidney in LPS-induced cardio-renal syndrome
LPS is a critical factor to induce severe and systemic inflammatory response and acute tissue injury on organs such as lung, liver and kidney during sepsis [Citation24]. In order to examine the impact of sepsis on cardiac and renal functions, we investigated the effects of NaSH (H2S donor) and SPRC, which provided exogenous H2S production, in LPS-treated mice. Although the concentration of serum H2S didn’t change obviously in each group, LPS induced H2S level decreasing in kidney and heart tissues, which could be reversed under NaSH or SPRC treatment (). Compared with vehicle group, the LPS group exhibited evident renal pathological injury examined by H&E staining, such as inflammatory cells infiltration, red blood cells “leaky”, tubular cell vacuolation and nuclear loss, tubular dilation and distortion. NaSH and SPRC relieved the renal injury by reducing inflammatory cells infiltration and tubular cells death (). The microscopic pictures of heart H&E staining showed a loosened structure of myocardial fibers with disorganization in LPS group compared with the vehicle group. After NaSH and SPRC treatment, the LPS-induced edema and rupture of myocardial fibers were mitigated (). The serum levels of Creatinine, BUN, and TnT were also measured. Creatinine and BUN, which reflected the renal function, were elevated in LPS-challenged group, but NaSH and SPRC could significantly decrease the serum level to show a protective effect of kidney (). Similarly, NaSH and SPRC showed a cardiac protective role by lowering serum TnT level after LPS-treatment (). These findings collectively demonstrated that NaSH and SPRC effectively mitigate tissue damage caused by LPS in vivo.
Figure 1. The protective effect of H2S donors in LPS-induced heart and kidney injury. (A) The H2S level in serum, kidney tissue and heart. (B) Representative pictures of H&E Staining of kidney. The renal tissue showed swelling, hydropic degeneration of tubules and vacuolization in renal tissue (red arrows). (C) Representative pictures of H&E Staining of heart. Myocardial fibers showed fragmentation and lysis, cell necrosis and vacuolar degeneration (red arrows). Scale bar: 100 μm. (D) The renal function was evaluated by serum creatine and BUN. (E) The heart function was evaluated by serum TnT. n = 8. Data are represented as Mean ± SD. *p < 0.05, **p < 0.01, ***p < 0.001 vs. Vehicle; #p < 0.05, ##p < 0.01, ###p < 0.001 vs. LPS.
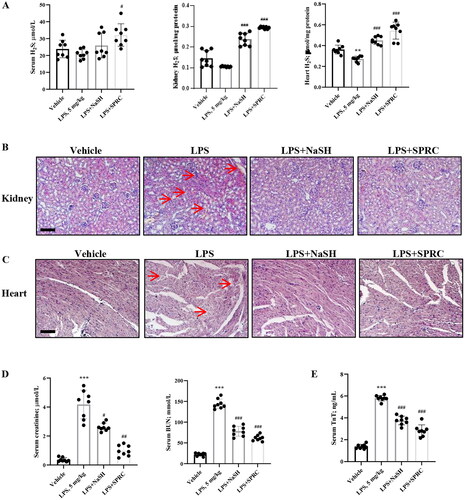
The role of macrophages in LPS-induced sepsis
The LPS-induced inflammatory response has been extensively utilized to replicate sepsis model in vitro and in vivo [Citation3], and it may be regarded as a potential drug target for sepsis treatment. The protective role of H2S has been demonstrated in regulating immune response [Citation25]. F4/80 was employed to assess the infiltration of macrophages in LPS-treated models. LPS significantly augmented the recruitment of the F4/80+ macrophages in kidney and heart tissues, whereas NaSH and SPRC relieved the infiltration of macrophages (). In order to elucidate the effect of macrophage in LPS-caused organ dysfunction, RAW264.7 cells were used to explore the mechanism in this study. NaSH is extensively employed in pharmacological research, but it is rapidly releasing, and high dosage will cause H2S toxicity. In this study, we chose different doses (0.1, 1, and 10 μM) of NaSH and SPRC respectively to explore the protective effect of exogenous H2S under LPS stimulation. The cell viability tested by CCK8 showed that LPS inhibited the macrophages proliferation, whereas NaSH and SPRC increased the cell number, even exhibited a pro-proliferation effect ().
Figure 2. The H2S donors regulate the macrophages recruitment and proliferation in LPS-induced sepsis model. Immunohistochemistry was used to detect F4/80+ macrophages (brown, indicated by red arrows) infiltration in kidney (A) and heart (B) tissues. (C) The cell proliferation rate was detected by CCK8. Data are represented as Mean ± SD. All experiments were repeated triplicate. *p < 0.05, **p < 0.01, ***p < 0.001 vs. M0; #p < 0.05, ##p < 0.01, ###p < 0.001 vs. LPS.
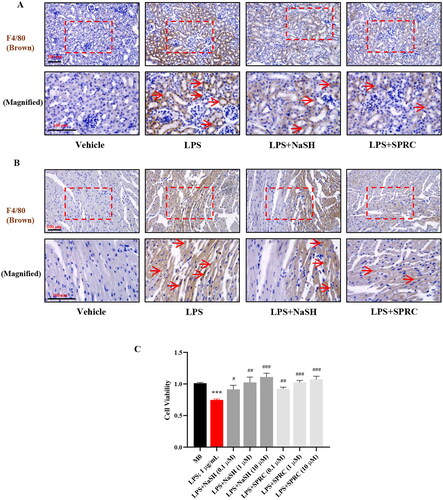
The H2S donors regulated macrophage functions and phenotypic switch
Macrophages play a key role in LPS-induced inflammation response. To investigate the effect of H2S in LPS-promoted phagocytosis of macrophages, the neutral red (NR) staining was employed, and the fluorescence intensity was assessed. It was observed that phagocytosis is enhanced under LPS stimulation; and notably, NaSH and SPRC significantly increased the phagocytotic effect upon LPS treatment ().
Figure 3. The H2S donors modulate the macrophage phenotypic in LPS-treated RAW264.7 cells. (A) The effect of H2S on macrophage phagocytosis function under LPS stimulation. (B) The macrophage phenotype changes of pro-inflammatory M1 and anti-proinflammatory M2 in mRNA level. The expression of IL-6 (C) and TNF-α (D) in cultured macrophages supernatant was measured by ELISA. Data are represented as Mean ± SD. All experiments were repeated triplicate. *p < 0.05, **p < 0.01, ***p < 0.001 vs. M0; #p < 0.05, ##p < 0.01, ###p < 0.001 vs. LPS.
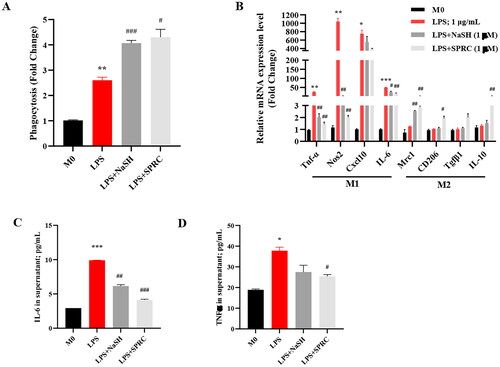
The polarization of macrophages is directly affecting their functions. The LPS simulated the macrophage polarizing toward M1 pro-inflammatory phenotype, and caused the production of a large number of pro-inflammatory cytokines. As shown in , the M1 makers, Tnf-α, Nos2, Cxcl10, and IL-6 were markedly increased in the mRNA level under LPS stimulation. Compared with LPS group, these M1 gene expressions decreased significantly in NaSH and SPRC-treated groups. Even though LPS alone did not change the M2-related gene obviously, H2S donors, especially SPRC elevated the M2 markers Mrc1, CD206, Tgfβ1, and IL-10 in a certain degree (2–3 fold changes). These results were accordingly related to the pro-inflammatory cytokines, including IL-6 and TNF-α expression in the supernatant of cultured macrophage cells (). These results imply that H2S may play a role in maintaining a balance between the pro-inflammation of M1 and anti-inflammation of M2, thereby preventing extensive inflammatory injury caused by LPS.
The H2S donors alleviated LPS-induced oxidative stress and ROS production in macrophages
It has been demonstrated that the LPS-induced macrophage inflammatory cytokines production was related to ROS-mediated NF-κB activation [Citation26]. In the LPS-induced sepsis model, LPS significantly increased the MDA content, but declined the CAT, SOD activities, and GSH content, which indicated an oxidative state in LPS-treated mice. Nevertheless, NaSH and SPRC treatment effectively modulate the oxidative imbalance in kidney () and heart () tissues.
Figure 4. The H2S donors reduced oxidative markers and ROS production in LPS-induced sepsis model. Oxidative and antioxidant parameters including MDA, CAT, SOD, and GSH of kidney tissues (A) and heart tissues (B) from each group of mice were measured. n = 8. (C) ROS generation in LPS-treated RAW264.7 macrophage cells were determined under fluorescence microscope (scale bar, 20 μm) and tested by fluorescent intensity. Data are represented as Mean ± SD. *p < 0.05, **p < 0.01, ***p < 0.001 vs. Vehicle; #p < 0.05, ##p < 0.01, ###p < 0.001 vs. LPS.
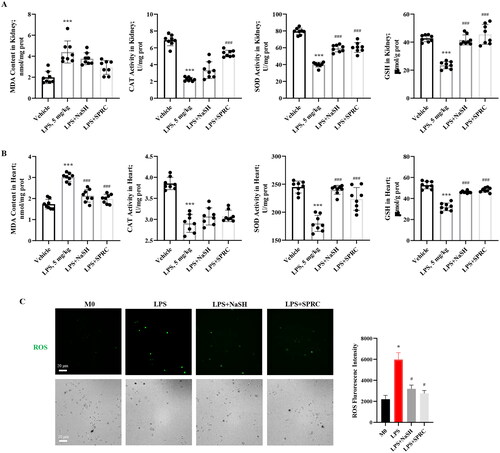
RAW264.7 macrophage cells were treated with LPS with or without NaSH or SPRC for 24 h to quantify the ROS production. Under LPS treatment, the cell number was decreased, but the ROS production was increased. The NaSH and SPRC could significantly decrease ROS production induced by LPS (), which demonstrated that the exogenous H2S may exhibit anti-oxidative effect, and subsequently alleviate the LPS-caused inflammatory injury.
The H2S donors could rescue impaired autophagy induced by LPS in macrophages
Autophagy has been recognized to play an important role in sepsis for its regulatory effect on immunity [Citation27]. LPS treatment decreased the LC3II expression, a major component of autophagosome formation, while augmented the abundance of autophagic cargo SQSTM1/P62 (). However, the impaired autophagy was rescued by the administration of NaSH or SPRC (1, 10 μM), which promoted the autophagosome formation. Additionally, fluorescence images showed that NaSH or SPRC (1 μM) alleviated the reduction of intracellular LC3 expression induced by LPS (), which is consistent with the results of proteins. To verify the autophagic activity, the utilization of a lysosome inhibitor called chloroquine (CQ) was employed. Under CQ treatment, increased LC3 expression served as evidence for active autophagy, referred to as “autophagic flux” [Citation28]. As shown in , LPS impaired autophagic flux by impeding the successful clearance of autophagosomes by lysosomes. Conversely, NaSH and SPRC (1 μM) effectively activated autophagic flux, with SPRC exhibiting a more pronounced effect.
Figure 5. The autophagy is activated by H2S donors in LPS-treated RAW264.7 cells. (A) RAW264.7 cells were treated with or without NaSH and SPRC (1, 10 μM) for 24 h, western blot was used to examine the expression of LC3, p62 and Beclin 1. (B) Cells were stained with anti-LC3 antibody to observe autophagosome formation and analyzed by DeltaVision Ultra. At least 30 cells were counted for each treatment. Scale bar, 20 μm. (C) CQ was used to examine autophagic flux. Data are represented as Mean ± SD. All experiments were repeated triplicate. *p < 0.05, **p < 0.01, ***p < 0.001 vs. M0; #p < 0.05, ##p < 0.01, ###p < 0.001 vs. LPS.
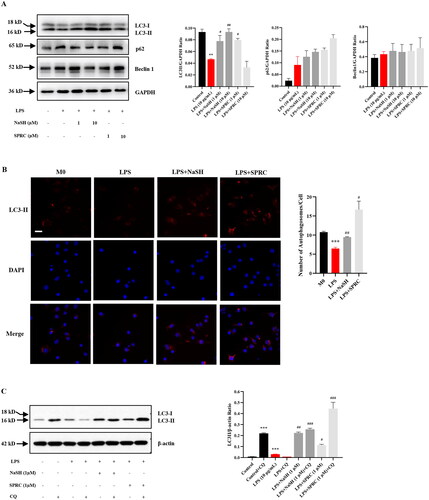
The H2S donors triggered mitophagy process through PINK1/Parkin pathway in LPS-challenged macrophages
ROS over-production was closely correlated with protein misfolding and organelles damages [Citation29]. To investigate the LPS-induced mitochondria injury due to excess ROS production, MitoTracker was utilized to evaluate mitochondria abundance, and a mitochondrion-specific fluorescent probe, TMRE, was employed to observe mitochondrial membrane potential. LPS decreased mitochondrial number () and caused mitochondrial membrane depolarization () compared with control. NaSH and SPRC (1 μM) significantly improved the mitochondrial function by increasing mitochondrial quantity and mitochondrial membrane integrity. It has been demonstrated that damaged mitochondria could be cleared by the autophagy process, known as mitophagy [Citation30]. The PINK1/Parkin pathway, which is related to mitophagy, was identified in LPS-treated macrophages [Citation31]. Compared with control, the expression of PINK1 and Parkin was upregulated in LPS-treated macrophages. The subsequent NaSH and SPRC treatment further enhanced the PINK1 expression, particularly with a dosage of 1 μM NaSH and 10 μM SPRC ().
Figure 6. H2S donors regulate the morphology and function of mitochondria in LPS-treated RAW264.7 cells. (A) Fluorogram of mitochondria abundance and membrane potential to show the effect of NaSH and SPRC in LPS-challenged macrophages. (B) Quantitative analysis of mitochondria abundance and membrane potential. (C) Western blot to examine the mitophagy markers PINK1 and Parkin expression in LPS-treated macrophages with or without NaSH and SPRC. Data are represented as Mean ± SD. All experiments were repeated triplicate. *p < 0.05, **p < 0.01, ***p < 0.001 vs. M0; #p < 0.05, ##p < 0.01, ###p < 0.001 vs. LPS.
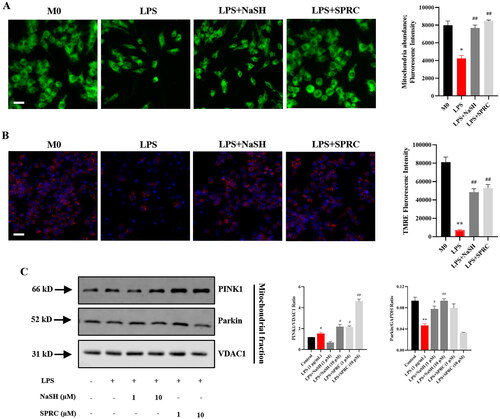
To further investigate the H2S donors-mediated mitophagy process through the PINK1/Parkin pathway, macrophages were transfected with Pink1 siRNA. As shown in , Pink1 knockdown weakened the protective effect of NaSH and SPRC under LPS-challenge macrophages. Accordingly, siPink1 significantly inhibited the NaSH and SPRC-induced autophagy activation in LPS-treated macrophages ().
Figure 7. The protective effect of H2S donors mainly through PINK1-mediated mitophagy in LPS-treated RAW264.7 cells. (A) Effects of mitophagy inhibition by siPink1 on cell viability. (B) Western blot to analyze mitophagy inhibition by siPink1 with or without H2S donors under LPS-treatment. Data are represented as Mean ± SD. All experiments were repeated triplicate. *p < 0.05, **p < 0.01, ***p < 0.001 vs. M0; #p < 0.05, ##p < 0.01, ###p < 0.001 vs. LPS.
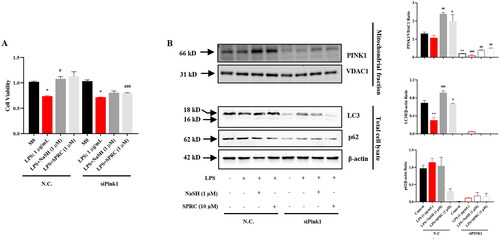
Discussion
Sepsis causes multi-organ dysfunctions and has become one of the leading causes of death worldwide contributor to mortality globally. LPS is is commonly employed to induce inflammatory response to mimic sepsis in vivo and in vitro. Macrophages play an important role in innate immune response by adopting two distinct phenotypes: the M1 phenotype, which producing pro-inflammatory cytokines (IL-1β, IL-6, TNF-α, etc.); and the M2 phenotype secreting anti-inflammatory cytokines (IL-4 and IL-10) for tissue repair. Modulating macrophage phenotype has been demonstrated essential effect on inflammation and facilitates the resolution of sepsis treatment [Citation32]. In this study, exogenous H2S donors were employed to elucidate the involvement of H2S-regulated macrophage phenotype change and functions in sepsis, in which the main mechanism was by modulating PINK1-mediated mitophagy ().
Figure 8. Graphic abstract. The schematic picture to demonstrate the immunoregulatory effect of hydrogen sulfide (H2S) in regulating macrophage phenotype change. LPS impaired the PINK1/Parkin-mediated mitophagy, which inducing the pro-inflammatory phenotype of macrophages. Otherwise, the H2S donors (NaSH and SPRC) rescued the damaged mitophagy process and promoted the macrophage switching to anti-inflammatory phenotype.
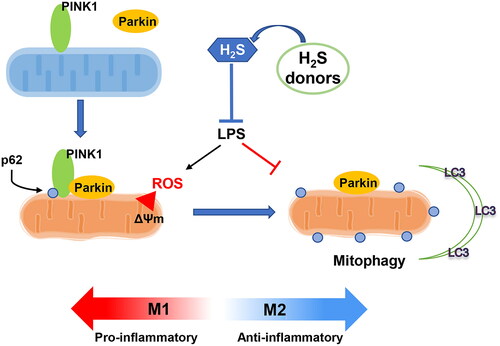
H2S is a gas molecule involved in signal transduction, possessing significant capabilities in both physiological and pathological process. However, the low and high dosage of the rapid-releasing H2S donors NaSH would cause controversial outcomes [Citation33]. The natural slow-releasing H2S donors have been paid more and more attention, notably, garlic exhibited protective effect in LPS-caused tissue damage [Citation34,Citation35]. OSCs are natural agents from garlic, which play multiple functions in various diseases through anti-inflammation [Citation36,Citation37], anti-oxidative stress [Citation38], and autophagy-inducing effects [Citation18], etc. The immunoregulatory function of OSCs has been demonstrated in various immune cells, including macrophages, lymphocytes, natural killer cells, dendritic cells [Citation39]. In this study, the protective effect of H2S was confirmed in LPS-induced sepsis model. The H2S donors, NaSH and SPRC showed a protective effect in LPS caused injury in kidney and heart, a common cardiorenal syndrome related to sepsis. The two H2S donors increased H2S levels in serum, kidney and heart tissues. The markers to represent kidney function (BUN, creatinine) and heart function (TnT) were relieved after NaSH and SPRC treatment in a murine sepsis model induced by LPS. F4/80, an indicator of macrophages, was ubiquitously expressed in kidney and heart tissues after LPS treatment. The NaSH and SPRC administration could decrease the macrophage infiltration. In LPS-treated RAW264.7 macrophages, the cytotoxicity of LPS was reversed by NaSH and SPRC.
LPS could induce macrophage phenotype change mainly toward the M1-phenotype by upregulating Tnf-α, Nos2, Cxcl10, and IL-6 [Citation6]. In neuroinflammation status, H2S exhibited anti-inflammatory effect by suppressing M1 and promoting M2 phenotype of microglia [Citation40]. In our study, NaSH and SPRC could reduce the inflammatory cytokine production, especially Tnf-α and Nos2; and increase M2 cytokines such as Mrc1, CD206, Tgfβ1, and IL-10 simultaneously. The regulation of cytokine changes by H2S donors indicated that H2S could balance the pro- and anti-inflammatory cytokine production, thereby preserving the macrophage phenotype and functions, and subsequently reducing diminished cell death and tissue destruction.
The induction of inflammation by LPS is accompanied with oxidative stress. The oxidative marker (MDA) was down-regulated, while anti-oxidative stress markers (CAT, SOD and GSH) were up-regulated by NaSH and SPRC treatment in heart and kidney tissues. Additionally, LPS triggered ROS production was evaluated in macrophages, which showed a remarkable reduction in NaSH and SPRC treatment group. These results implied that H2S donors relieved oxidative stress induced by LPS in macrophages.
It has been demonstrated the involvement of autophagy in innate immunity, which participated in regulating inflammatory response [Citation41]. Autophagy is a cellular quality control process by eliminating damaged micro-organelles, misfolded proteins and protein aggregates, or even degrading overload lipids. Since autophagy has been reported to modulate inflammatory response in sepsis, it is worthy to discover new small molecules targeting autophagic pathway for the treatment of inflammatory diseases [Citation42]. In our study, the H2S donors NaSH and SPRC have been shown beneficial effects in modulating autophagy in LPS-treated macrophages. As active autophagy is indicated by autophagy flux, lysosome inhibitor CQ was used [Citation28]. LPS impaired autophagy by inhibiting autophagic flux; however, H2S could promote autophagy flux to activate autophagy in the LPS-induced inflammatory process [Citation43]. Previous studies have demonstrated that intact mitophagy could prevent inflammasome activation [Citation31]. Sun Y et al. have reported that Beclin-1-mediated cardiac mitophagy regulated mitochondria quality control during sepsis [Citation44]. Zhong Z et al. have specifically deleted the gene encoding SQSTM1/p62 in macrophage, which resulted in excessive inflammasome activation, accumulation of damaged mitochondria, and cell death [Citation45]. LPS caused mitochondrial numbers decreasing and the membrane potential reducing, while NaSH and SPRC rescued LPS-induced mitochondrial injury and cell death.
Mitophagy can occur through multiple pathways, including targeting of damaged mitochondria with lost membrane potential by PINK1 [Citation46]. PINK1 accumulates at the outer mitochondrial membranes (OMM), and recruits PARK2 from cytosol which consequently ubiquitinated for reorganization by microtubule-associated protein 1 light chain 3 beta (LC3B) to form autophagosome and degradation [Citation11,Citation47]. Other pathways, such as mitochondrial receptors, BCL2, adenovirus E1B BNIP3, BNIP3-like (BNIP3L/NIX), and FUNDC1 have also been demonstrated in mitophagy [Citation13]. In this study, we have demonstrated that H2S mainly functions in LPS-related mitophagy through PINK1/Parkin pathway. To interfere Pink1 expression by siRNA, NaSH and SPRC could not protect LPS-induced macrophage death and autophagy activation. The results indicated the protective role of H2S by activating PINK1-Parkin pathway-mediated mitophagy.
Conclusions
As mentioned above, LPS changed macrophage phenotype and functions by impairing autophagy in sepsis. The H2S donors, NaSH and SPRC, could relieve the LPS-induced cell death and tissue damage by promoting autophagy flux. The main mechanism is by regulating PINK1/Parkin pathway triggered mitophagy, which maintains the immunoregulatory function of macrophages. In this process, the natural H2S donor SPRC showed more effective effect in activating mitophagy and tissue protection, suggesting its potential as a promising small molecule compound for sepsis treatment. Nevertheless, more natural H2S donors could be investigated in the treatment of sepsis, and the precise mechanism in regulating the function of immune cells needs to be studied further.
Disclosure statement
No potential conflict of interest was reported by the author(s).
Data availability statement
The data that support the findings of this study are available from the corresponding author, S. L. and G. Y., upon reasonable request.
Additional information
Funding
References
- Kotecha A, Vallabhajosyula S, Coville HH, et al. Cardiorenal syndrome in sepsis: a narrative review. J Crit Care. 2018;43:122–127. doi: 10.1016/j.jcrc.2017.08.044.
- Hotchkiss RS, Monneret G, Payen D. Sepsis-induced immunosuppression: from cellular dysfunctions to immunotherapy. Nat Rev Immunol. 2013;13(12):862–874. doi: 10.1038/nri3552.
- Pfalzgraff A, Weindl G. Intracellular lipopolysaccharide sensing as a potential therapeutic target for sepsis. Trends Pharmacol Sci. 2019;40(3):187–197. doi: 10.1016/j.tips.2019.01.001.
- Shapouri-Moghaddam A, Mohammadian S, Vazini H, et al. Macrophage plasticity, polarization, and function in health and disease. J Cell Physiol. 2018;233(9):6425–6440. doi: 10.1002/jcp.26429.
- Gordon S, Pluddemann A, Martinez Estrada F. Macrophage heterogeneity in tissues: phenotypic diversity and functions. Immunol Rev. 2014;262(1):36–55. doi: 10.1111/imr.12223.
- Carestia A, Mena HA, Olexen CM, et al. Platelets promote macrophage polarization toward pro-inflammatory phenotype and increase survival of septic mice. Cell Rep. 2019;28(4):896–908 e5. Jul 23 doi: 10.1016/j.celrep.2019.06.062.
- Levine B, Kroemer G. Biological functions of autophagy genes: a disease perspective. Cell. 2019;176(1–2):11–42. doi: 10.1016/j.cell.2018.09.048.
- Mantzarlis K, Tsolaki V, Zakynthinos E. Role of oxidative stress and mitochondrial dysfunction in sepsis and potential therapies. Oxid Med Cell Longev. 2017;2017:5985209–5985210. doi: 10.1155/2017/5985209.
- Xu Y, Shen J, Ran Z. Emerging views of mitophagy in immunity and autoimmune diseases. Autophagy. 2020;16(1):3–17. doi: 10.1080/15548627.2019.1603547.
- Zhou H, Ren J, Toan S, et al. Role of mitochondrial quality surveillance in myocardial infarction: from bench to bedside. Ageing Res Rev. 2021;66:101250. doi: 10.1016/j.arr.2020.101250.
- Eiyama A, Okamoto K. PINK1/parkin-mediated mitophagy in mammalian cells. Curr Opin Cell Biol. 2015;33:95–101. doi: 10.1016/j.ceb.2015.01.002.
- Zhang J, Ney PA. Role of BNIP3 and NIX in cell death, autophagy, and mitophagy. Cell Death Differ. 2009;16(7):939–946. doi: 10.1038/cdd.2009.16.
- Lampert MA, Orogo AM, Najor RH, et al. BNIP3L/NIX and FUNDC1-mediated mitophagy is required for mitochondrial network remodeling during cardiac progenitor cell differentiation. Autophagy. 2019;15(7):1182–1198. doi: 10.1080/15548627.2019.1580095.
- Dilek N, Papapetropoulos A, Toliver-Kinsky T, et al. Hydrogen sulfide: an endogenous regulator of the immune system. Pharmacol Res. 2020;161:105119. doi: 10.1016/j.phrs.2020.105119.
- Beltowski J. Hydrogen sulfide in pharmacology and medicine–an update. Pharmacol Rep. 2015;67(3):647–658. doi: 10.1016/j.pharep.2015.01.005.
- Yagdi E, Cerella C, Dicato M, et al. Garlic-derived natural polysulfanes as hydrogen sulfide donors: friend or foe? Food Chem Toxicol. 2016;95:219–233. doi: 10.1016/j.fct.2016.07.016.
- Wu Y, Hu Y, Zhou H, et al. Organosulfur compounds induce cytoprotective autophagy against apoptosis by inhibiting mTOR phosphorylation activity in macrophages. Acta Biochim Biophys Sin. 2018;50(11):1085–1093. doi: 10.1093/abbs/gmy114.
- Li S, Yang G, Zhu X, et al. Combination of rapamycin and garlic-derived S-allylmercaptocysteine induces Colon cancer cell apoptosis and suppresses tumor growth in xenograft nude mice through autophagy/p62/Nrf2 pathway. Oncol Rep. 2017;38(3):1637–1644. doi: 10.3892/or.2017.5849.
- Wen YD, Zhu YZ. The pharmacological effects of S-propargyl-cysteine, a novel endogenous H2S-Producing compound. Handb Exp Pharmacol. 2015;230:325–336. doi: 10.1007/978-3-319-18144-8_16.
- Wang Q, Liu HR, Mu Q, et al. S-propargyl-cysteine protects both adult rat hearts and neonatal cardiomyocytes from ischemia/hypoxia injury: the contribution of the hydrogen sulfide-mediated pathway. J Cardiovasc Pharmacol. 2009;54(2):139–146. doi: 10.1097/FJC.0b013e3181ac8e12.
- Wang Q, Wang XL, Liu HR, et al. Protective effects of cysteine analogues on acute myocardial ischemia: novel modulators of endogenous H(2)S production. Antioxid Redox Signal. 2010;12(10):1155–1165. May 15 doi: 10.1089/ars.2009.2947.
- Wang M, Tang W, Xin H, et al. S-propargyl-cysteine, a novel hydrogen sulfide donor, inhibits inflammatory hepcidin and relieves anemia of inflammation by inhibiting IL-6/STAT3 pathway. PLoS One. 2016;11(9):e0163289. doi: 10.1371/journal.pone.0163289.
- Sidhapuriwala JN, Hegde A, Ang AD, et al. Effects of S-propargyl-cysteine (SPRC) in caerulein-induced acute pancreatitis in mice. PLoS One. 2012;7(3):e32574. doi: 10.1371/journal.pone.0032574.
- Virzi GM, Breglia A, Castellani C, et al. Lipopolysaccharide in systemic circulation induces activation of inflammatory response and oxidative stress in cardiorenal syndrome type 1. J Nephrol. 2019;32(5):803–810. doi: 10.1007/s40620-019-00613-2.
- Li T, Chu X, Xin D, et al. H2S prevents peripheral immune cell invasion, increasing [Ca(2+)]i and excessive phagocytosis following hypoxia-ischemia injury in neonatal mice. Biomed Pharmacother. 2021;135:111207. doi: 10.1016/j.biopha.2020.111207.
- Maciel E, Neves BM, Martins J, et al. Oxidized phosphatidylserine mitigates LPS-triggered macrophage inflammatory status through modulation of JNK and NF-kB signaling Cascades. Cell Signal. 2019;61:30–38. doi: 10.1016/j.cellsig.2019.04.015.
- Deretic V, Levine B. Autophagy balances inflammation in innate immunity. Autophagy. 2018;14(2):243–251. doi: 10.1080/15548627.2017.1402992.
- Pasquier B. Autophagy inhibitors. Cell Mol Life Sci. 2016;73(5):985–1001. doi: 10.1007/s00018-015-2104-y.
- Kowald A, Hamann A, Zintel S, et al. A systems biological analysis links ROS metabolism to mitochondrial protein quality control. Mech Ageing Dev. 2012;133(5):331–337. doi: 10.1016/j.mad.2012.03.008.
- Wang Y, Jasper H, Toan S, et al. Mitophagy coordinates the mitochondrial unfolded protein response to attenuate inflammation-mediated myocardial injury. Redox Biol. 2021;45:102049. doi: 10.1016/j.redox.2021.102049.
- Patoli D, Mignotte F, Deckert V, et al. Inhibition of mitophagy drives macrophage activation and antibacterial defense during sepsis. J Clin Invest. 2020;130(11):5858–5874. doi: 10.1172/JCI130996.
- Delano MJ, Ward PA. Sepsis-induced immune dysfunction: can immune therapies reduce mortality? J Clin Invest. 2016;126(1):23–31. doi: 10.1172/JCI82224.
- Pouokam E, Althaus M. Epithelial electrolyte transport physiology and the gasotransmitter hydrogen sulfide. Oxid Med Cell Longev. 2016;2016:4723416–4723413. doi: 10.1155/2016/4723416.
- Kim MJ, Yoo YC, Kim HJ, et al. Aged black garlic exerts anti-inflammatory effects by decreasing no and proinflammatory cytokine production with less cytoxicity in LPS-stimulated raw 264.7 macrophages and LPS-induced septicemia mice. J Med Food. 2014;17(10):1057–1063. doi: 10.1089/jmf.2013.3043.
- Rousta AM, Mirahmadi SM, Shahmohammadi A, et al. S-allyl cysteine, an active ingredient of garlic, attenuates acute liver dysfunction induced by lipopolysaccharide/d-galactosamine in mouse: underlying mechanisms. J Biochem Mol Toxicol. 2020;26:e22518. doi: 10.1002/jbt.22518.
- Yang G, Sun S, Wang J, et al. S-Allylmercaptocysteine targets Nrf2 in osteoarthritis treatment through NOX4/NF-kappaB pathway. Drug Des Devel Ther. 2020;14:4533–4546. doi: 10.2147/DDDT.S258973.
- Lee DY, Li H, Lim HJ, et al. Anti-inflammatory activity of sulfur-containing compounds from garlic. J Med Food. 2012;15(11):992–999. doi: 10.1089/jmf.2012.2275.
- Zhu X, Jiang X, Li A, et al. S-allylmercaptocysteine attenuates cisplatin-induced nephrotoxicity through suppression of apoptosis, oxidative stress, and inflammation. Nutrients. 2017;9(2):166. Feb 20 doi: 10.3390/nu9020166.
- Schepetkin IA, Kirpotina LN, Khlebnikov AI, et al. Neutrophil immunomodulatory activity of natural organosulfur compounds. Molecules. 2019;24(9):1809. doi: 10.3390/molecules24091809.
- Duan H, Li L, Shen S, et al. Hydrogen sulfide reduces cognitive impairment in rats after subarachnoid hemorrhage by ameliorating neuroinflammation mediated by the TLR4/NF-kappaB pathway in microglia. Front Cell Neurosci. 2020;14:210. doi: 10.3389/fncel.2020.00210.
- Shibutani ST, Saitoh T, Nowag H, et al. Autophagy and autophagy-related proteins in the immune system. Nat Immunol. 2015;16(10):1014–1024. doi: 10.1038/ni.3273.
- Ho J, Yu J, Wong SH, et al. Autophagy in sepsis: degradation into exhaustion? Autophagy. 2016;12(7):1073–1082. doi: 10.1080/15548627.2016.1179410.
- Ashrafi G, Schwarz TL. The pathways of mitophagy for quality control and clearance of mitochondria. Cell Death Differ. 2013;20(1):31–42. doi: 10.1038/cdd.2012.81.
- Sun Y, Yao X, Zhang QJ, et al. Beclin-1-dependent autophagy protects the heart during sepsis. Circulation. 2018;138(20):2247–2262. Nov 13 doi: 10.1161/CIRCULATIONAHA.117.032821.
- Zhong Z, Umemura A, Sanchez-Lopez E, et al. NF-kappaB restricts inflammasome activation via elimination of damaged mitochondria. Cell. 2016;164(5):896–910. doi: 10.1016/j.cell.2015.12.057.
- Zhu H, Toan S, Mui D, et al. Mitochondrial quality surveillance as a therapeutic target in myocardial infarction. Acta Physiol. 2021;231(3):e13590. doi: 10.1111/apha.13590.
- Sun D, Wang J, Toan S, et al. Molecular mechanisms of coronary microvascular endothelial dysfunction in diabetes mellitus: focus on mitochondrial quality surveillance. Angiogenesis. 2022;25(3):307–329. doi: 10.1007/s10456-022-09835-8.