Abstract
Staphylococcal colonization of implants is a serious complication of orthopaedic surgery. Anti-infectious modification of implant surfaces may serve to prevent bacterial colonization. The authors set out to develop an in vitro test system for the analysis of prevention of biofilm formation by Staphylococcus epidermidis and Staphylococcus aureus on implant materials. Biofilm growth was monitored over 10 days on titanium disks in order to develop appropriate test parameters. Bacterial cell counts following ultrasonic treatment of the colonized samples were compared with scanning electron microscope images of the specimens. Copper ion containing surfaces (ie copper [Cu] and inter-metallic Ti-Cu films) were used for growth inhibition assays: Copper ion releasing specimens led to reduced bacterial numbers in biofilms and decreased bacterial persistence in the model used. The assay used represents an inexpensive and quick in vitro screen for the antibacterial effects of novel implant surface materials.
Introduction
Infections of endoprostheses are relatively rare but cause serious complications of total joint replacement. Even though the risk of implant-associated infections has decreased in recent years to 0.5–2% (Phillips et al. Citation2006; Hellman et al. Citation2009), the total number of infections is rising due to the growing number of joint replacements in the ageing population (Zimmerli Citation2005; Del Pozo and Patel Citation2009). Endoprosthetic joint infections have been classified according to their temporal occurrence in early (<3 months post surgery), delayed, and late infections (>24 months post surgery) (Coventry Citation1975). Early infections are commonly acquired during the surgical procedure, whereas the majority of late infections are thought to be caused by hematogenous seeding from remote sources (Zimmerli et al. Citation2004). Typical risk factors for early endoprosthetic joint infections include obesity, diabetes mellitus, the duration of surgery, and revision procedures (Choong et al. Citation2007; Uckay et al. Citation2009).
The two predominant microorganisms responsible for bacterial infections of implants are Staphylococcus epidermidis and Staphylococcus aureus (Barth et al. Citation1989; Rupp and Archer Citation1994). Generally, prosthetic infections are caused by bacterial cells organized in a biofilm on the surface of the implant (Gristina and Costerton Citation1985). During biofilm formation, the microorganisms secrete a polysaccharide matrix, which protects the cells from host defence factors (Costerton et al. 1999; Phillips et al. Citation2006; Hellmann et al. Citation2009). In addition, growth within the polysaccharide matrix, changes in growth rate and metabolism, and the development of persister cells within the biofilm decrease the susceptibility of the bacteria to therapeutically administered antibiotics and hamper their detection by microbiological diagnostic procedures (Brown et al. Citation1988; Stewart and Costerton Citation2001; Hoiby et al. Citation2011; Rogers et al. Citation2011).
Infection prevention is a major goal for scientists and clinicians working on the development of optimized artificial joints. The reasons for this are the high morbidity and cost of implant-associated infections. Additionally, there is a risk of severe complications directly caused by the infection or induced by surgical treatment of the infection, eg removal of the respective implant. A promising strategy to decrease the probability of infection is the anti-infectious surface coating of implant materials with the aim of controlled release of the antibacterial compounds (Kwok et al. Citation1999; Gollwitzer et al. Citation2003; Gagliardi et al. Citation2010).
When developing such surface modifications, the antibacterial effects have to be studied in an appropriate in vitro test system. Preferably, the experimental setting should allow testing of the influence of the antibacterial agents on biofilm formation rather than on planktonic growth. Testing of potential implant materials is often done by measuring planktonic growth in the presence of the respective material or by ‘zone of inhibition’ determination (Jose et al. Citation2005; Feng et al. Citation2008; Li et al. Citation2009). Short-term incubation (5–24 h) of bacterial suspensions directly on implant materials and analysis of viable counts is also a standardized method to examine antibacterial activity (Japanese Industrial Standards, described in Shirai et al. Citation2009).
One of the well established high-throughput assays for the determination of antibiotic susceptibilities of bacterial biofilms uses the Calgary Biofilm Device (CBD) (Ceri et al. Citation1999; Harrison et al. Citation2010). In this system, biofilms of different bacterial species are allowed to form on the CBD pegs, before susceptibility towards antimicrobial substances is tested. The same principle is applied by commercially available in vitro diagnostic tools based on this invention (MBEC Assay™, Innovotech, Edmonton, Alberta, Canada). Another approach to study biofilms is the use of capillary flow cells, which allow observation of the different biofilm stages under constant nutrient, salt and pH conditions (Pratten and Ready Citation2010).
However, the present study aimed at developing a system that was comparable to the situation in the implant-associated wound. Little is known about potential flow rates in the implant environment. It appears prudent to expect some low level substance transport and liquid exchange. Flow chambers or periodical media exchange will most likely overestimate the flow in this environment. Consistently, in a mouse model of implant-associated osteomyelitis, bacterial metabolic activity as monitored by bioluminescence peaked on day 4 of infection, although the total bacterial load as determined by qRT-PCR increased until day 11 (Li et al. Citation2008). It is also not known to what degree shear forces inherent to flow systems resemble the situation on the implant surface with respect to biofilm formation and maturation. Most importantly, rapid dilution of antimicrobial or antiseptical agents released from the tested material would change the outcome of the experiment by allowing growth even of the most susceptible species. Therefore, the batch culture approach supports biofilm maturation with minimal metabolic activity and thus tolerance to potentially harmful substances of the involved bacterial cells. It also allows extended observation periods to monitor biofilm formation kinetics including delayed growth after exhaustion of potentially inhibitory substances.
In the present study, a repeatable batch culture test system was established by examining biofilm formation under various conditions on hydroxyapatite blasted titanium alloy disks (Ti-6Al-4V_HA). By using well established biofilm forming strains of the species S. epidermidis and S. aureus, the degree of biofilm development was studied. Efficient removal of adherent bacteria from the metal specimens was achieved by sonication and allowed the quantification of adherent bacterial cells on different surfaces. Planktonic growth plus biofilm formation in established bacterial culture medium supplemented with human serum were also compared to experiments in tissue culture medium. Repeatable quantification of adhered bacteria accompanied by monitoring of biofilm formation by SEM studies enabled the authors to conclude that simple bacterial count determination of adhered bacteria could serve as an indicator for biofilm formation on varying surfaces. Thus, initial evaluation of novel materials could be performed without corresponding SEM monitoring.
As a proof of principle, the development of staphylococcal biofilms on two antimicrobial samples, viz. copper (Cu) and inter-metallic Ti-Cu films was explored. Cu represents a promising metal ion for deposition applications because of its low eukaryotic cell toxicity and high cytocompatibility (Heidenau et al. Citation2005). Furthermore, copper is a metabolizable agent (Shirai et al. Citation2009) whereas silver, another metal ion frequently used for implant surface modifications, tends to stay in the human body and increases the silver serum levels (Gollwitzer et al. Citation2003). For these reasons copper was employed as the antibacterial agent in the present study.
A Cu-based inter-metallic thin film was deposited on the TiAlV substrata. A second component of the inter-metallic alloy was titanium, which was used to increase adhesion to TiAlV surfaces and to increase the hardness of the Ti-Cu films (Stranak et al. Citation2011). Differential antimicrobial effects of Cu and Ti-Cu films were detected and, moreover, differences in the susceptibility of the two species towards Ti-Cu films could be observed. In summary, a quantitative and inexpensive system was developed that permitted the study of the prevention of biofilm formation on diverse surfaces in a simple format.
Materials and methods
Bacterial strains and culture conditions
S. epidermidis strain RP 62A (ATCC 35984) and S. aureus strain ATCC 25923 were used in this study. If not mentioned otherwise, the strains were grown in Caso-Bouillion (CB) medium (Carl Roth, Karlsruhe, Germany) overnight at 37°C and in a 5% CO2–20% O2 atmosphere. Since transition phase staphylococci produce adhesins at a higher rate as opposed to stationary phase bacteria, the overnight culture was diluted 1:20 in CB and incubated for 6 to 8 h at 37°C and in a 5% CO2–20% O2 atmosphere to the transitional growth phase. Bacterial cells were washed with phosphate-buffered saline (PBS: 2 g KCl, 2.4 g KH2PO4, 80 g NaCl and 14.4 g Na2HPO4, to 1000 ml; pH 7.4) and adjusted with culture medium to 1 × 102 colony forming units CFU ml−1. Use was made of the fact that the composition of serum and interstitial fluid are very similar and CB was supplemented with 50% human serum (CB-HS) (Sigma-Aldrich Chemie, Munich, Germany) to mimic the in vivo situation. Alternatively, cells were adjusted with Dulbecco’s Modified Eagle Medium (DMEM) (Invitrogen, Karlsruhe, Germany) supplemented with 10% FCS (PAA Laboratories, Pasching, Austria). One ml of this suspension (correspondingly 102 CFU) was taken for inoculation of the experimental samples.
In vitro growth assays in 24-well plates
Twenty four-well polystyrole plates were prepared with either CB supplemented with 50% human serum or DMEM and one metal disk as described in the section Titanium and copper specimens. Four wells per experiment were prepared in parallel, ie one well for Scanning electron microscopy (SEM) and three wells for viability count determination (technical replicates). As contamination control, three to four wells were prepared with the respective medium and no bacterial inoculum on the same plate. The remaining wells in the 24-well polystyrole plates were inoculated with 1 ml of bacterial suspensions containing 1 × 102 CFU well−1. Six identical plates were prepared for each experiment. On the days indicated (days 0, 1, 2, 4, 7, 10) the samples were harvested and processed as described in the following sections. Principle organization of the plates is shown in Supplementary [Supplementary material is available via a multimedia link on the online article webpage]. The plates were incubated at 37°C in a 5% CO2–20% O2 atmosphere. Each experiment was performed on four independent occasions (biological replicates).
Figure 1. Bacterial growth in the presence of Ti-6Al-4V_HA disks. Comparison of growth in CB/HS medium with growth in DMEM tissue culture medium. (A) Planktonic growth of S. epidermidis in the presence of Ti-6Al-4V_HA disks detected by CFU determination of culture supernatants; (B) planktonic growth of S. aureus in the presence of Ti-6Al-4V_HA disks detected by CFU determination of culture supernatants; (C) formation of S. epidermidis biofilms on Ti-6Al-4V_HA disks detected by sonication of the Ti-6Al-4V_HA specimens followed by CFU determination; (D) formation of S. aureus biofilms on Ti-6Al-4V_HA disks detected by sonication of the Ti-6Al-4V_HA specimens followed by CFU determination.
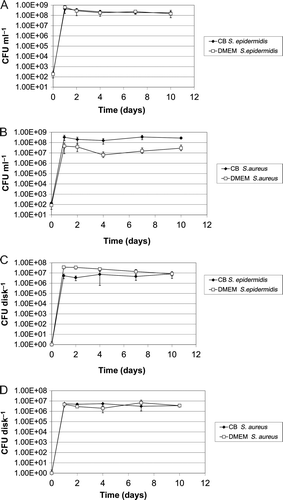
Analysis of planktonic growth in the presence of metal specimens
Analysis of planktonic growth was performed at days 0, 1, 2, 4, 7, and 10. The culture supernatant was collected, the disks were carefully washed using 1 ml of PBS, and the washing fluid was pooled with the supernatant (4,000 rpm, 10 min, 4°C, Heraeus Varifuge 3.OR; Kendro Laboratory Products, Osterode, Germany). Serial dilutions of the pooled samples were plated on CB agar plates and the number of cultivable bacteria was determined as CFU ml−1 following incubation for 24 h at 37 C in a 20% O2–5% CO2 atmosphere.
Analyses of bacterial biofilm formation on metal specimens
Numbers of adherent bacteria were determined with the PBS-washed disks from the experiments described above. For that purpose, the disks were carefully removed from the wells and transferred to a glass test tube containing 1 ml of PBS. To detach the biofilm from the metal disks, the tubes underwent low frequency (35 kHz) ultrasonic treatment (SONOREX DIGITAL 10P, Bandelin, Berlin, Germany). The optimal, ie least destructive but optimal suspending conditions for each strain had previously been determined experimentally (data not shown). In the experiments shown here, ultrasonic treatment was employed for 4 min at 100% intensity (S. epidermidis) and for 5 min at 80% intensity (S. aureus), respectively. Following sonication, the tubes were vortexed for ∼3 s. One hundred μl aliquots were removed from the tubes, serially diluted, and plated on CB agar plates. The number of cultivable bacteria was counted and calculated as CFU disk−1 following incubation for 24 h at 37°C in a 20% O2–5% CO2 atmosphere.
Scanning electron microscopy (SEM)
Disks with intact biofilms were analysed by SEM. Fixation of the disks was performed by incubation for 24 h in a solution containing 2.5% glutaraldehyde (Merck, Darmstadt, Germany). The samples were washed with 0.1 M sodium acetate buffer and dehydrated by passing through a graded series of ethanol-water mixtures to 100% ethanol and then dried by the critical-point method with CO2. The samples were sputtered with gold using a SCD 004 Balzers Sputter Coater (Bal Tec AG, Liechtenstein) and examined with a Zeiss DSM 960A electron microscope (Carl Zeiss, Jena, Germany).
Titanium and copper specimens
For a bacterial growth reference, the following titanium disks of chemically pure quality were employed: Ti-6AI-4V_HA, 11 mm diameter, 2 mm thickness, hydroxyapatite (HA) blasted (DOT GmbH, Rostock, Germany) and a roughness of Ra = 0.725 μm. Ti-6AI-4V disks were also used for the preparation of Ti-Cu films.
For complementary investigations, copper disks of the same size (Cu, Cziotek GmbH, Greifswald, Germany) were prepared. The samples were cut from sheets of chemically pure Cu with a roughness of Ra = 0.860 and 0.210 μm on front and back sides, respectively. Before further usage, the surfaces were cleaned in diluted nitric acid and rinsed with deionized water to neutrality.
Deposition of thin Ti-Cu films
Thin Ti-Cu films were prepared by plasma-assisted dual high-power impulse magnetron sputtering (dual-HiPIMS). The method is described elsewhere (Stranak et al. 2011). In brief, two sputtering sources (Gencoa, Liverpool, UK), with opposite magnetic field configurations equipped with Ti and Cu targets are situated in a vacuum chamber. Argon was used as a carrier gas whose ions provide sputtering of metal targets. The pressure was kept constant (p = 3 Pa) during all experiments. The main feature of HiPIMS discharges is operation in a pulsed regime with low repetition frequency and a short duty cycle. The dual-HiPIMS system is based on the parallel combination of two electrical circuits alternately employing sputtering sources as cathode and anode. Each electrical circuit employs a DC power supply, designed for continuous operation, used for charging a large capacitor bank during the idle phase of the pulses. The conditions for magnetron sputtering were as follows: repetition frequency 100 Hz; pulse width 100 μs; duty cycle, 1%. The voltages were set to adjust the required mean discharge currents of 100 mA for copper and 400 mA for titanium. The sputtered particle condensate on TiAlV substrata placed 50 mm from the target results in the formation of a homogeneous film. Homogeneity of the Ti-Cu composition and film thickness are achieved by the rotation of a substratum holder (diameter 160 mm, 5 rpm).
Sterilization of the metal specimens
Both the titanium and copper samples were packed into sterilization foils (Direct, Konstanz, Germany), sealed, and γ-sterilized with a minimum dose of 25 kGy of Co-60 radiation (Gamma Service, Radeberg, Germany).
Copper release measurement
The concentration of copper released from Ti-Cu films deposited on TiAlV substrata was measured by atomic absorption spectrometry (AAS) (ZEEnit 650, Analytik Jena AG, Jena, Germany) with electrothermal atomization (Zietz et al. Citation2012). The coated substrata were stored in 700 μl of DMEM (Invitrogen, Carlsbad, USA) with 10% fetal calf serum (FCS Gold, PAA Laboratories GmbH, Pasching, Austria) at 37°C in a humidified atmosphere with 5% CO2. The Cu concentration in the DMEM solution was measured at 24 h, 4 and 10 days on five samples. The DMEM was changed after 24 h and 4 days.
Statistical analysis
Data are presented as mean ± standard error (SE) of the mean. The significance of growth inhibition by Cu disks in comparison to Ti-6Al-4V_HA disks and Ti-Cu films was analyzed using the two-tailed Mann–Whitney U-test.
Results
S. epidermidis and S. aureusbiofilm formation on titanium (Ti-6Al-4V_HA) surfaces
A prerequisite for the investigation of antibacterial effects of implant materials in vitro is the detection of biofilm formation on the specimens of interest. Titanium alloy is a material without intrinsic antibacterial effects, which is widely employed for prosthetic implants. Thus, biofilm formation by the S. epidermidis and S. aureus strains on Ti-6Al-4V_HA specimens was first studied by comparing vital bacterial counts with SEM analysis. Therefore, 1x102 CFU of transition phase bacteria were seeded in wells containing the Ti-6Al-4V_HA samples in Caso-Bouillion medium supplemented with human serum (CB/HS). To detect planktonic bacteria, culture supernatants were examined at different time points (A and B). In parallel, adherent cells were quantified after gentle washing, sonication of the specimens in defined volumes (Christensen et al. Citation1995; Bjerkan et al. Citation2009), and serial dilution plus CFU determination (C and D). Cell numbers of biofilm organized S. epidermidis and S. aureus reached their maxima within 1 day and then slightly decreased within the next 9 days.
Testing of modified surfaces should use experimental conditions, which simultaneously allow investigation of bacterial and host cell behaviour in the presence of such materials. Therefore, S. epidermidis and S. aureus planktonic growth and counts of adherent cells on Ti-6Al-4V_HA specimens were compared using CB/HS bacterial culture medium and DMEM/10% FCS tissue culture medium. Adherent cells could be detected on titanium alloy Ti-6Al-4V_HA specimens in CB/HS as well as in the tissue culture medium. Both planktonic and adherent bacteria reached comparable cell counts in both media (). The differences between two of the samples were marginally significant (B and C) (P < 0.05, two-tailed U test). However, bacterial growth in all DMEM samples was high enough to allow further investigation of biofilm formation under various experimental conditions.
In a second approach, the development of biofilms on the Ti-6Al-4V_HA specimens by S. epidermidis and S. aureus was observed by SEM for the purpose of visual confirmation. Representative results for bacterial cells in DMEM on days 0, 1, and 10 for S. epidermidis and S. aureus are shown in and , respectively. Consistent with the results from the viability counts, adherent bacteria from both strains could be detected on the chips during incubation. Furthermore, S. epidermidis as well as S. aureus formed biofilm structures on the Ti-6Al-4V_HA specimens over the course of the experiment.
Figure 2. SEM micrographs of Ti-6Al-4V_HA disks inoculated with S. epidermidis. A, B = day 0; C, D = day 2; E, F = day 10. Scale bars: A, C, E = 40 μm; B, D, F = 4 μm.
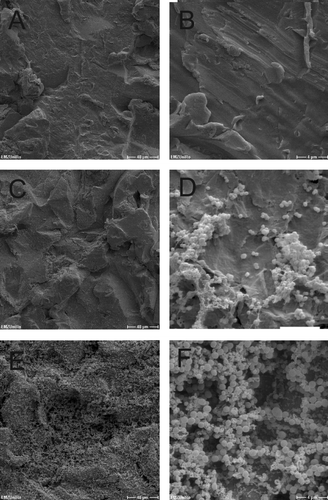
Figure 3. SEM micrographs of Ti-6Al-4V_HA disks inoculated with S. aureus. A, B = day 0; C, D = day 2; E, F = day 10. Scale bars: A, C, E = 40 μm; B, D, F = 4 μm.
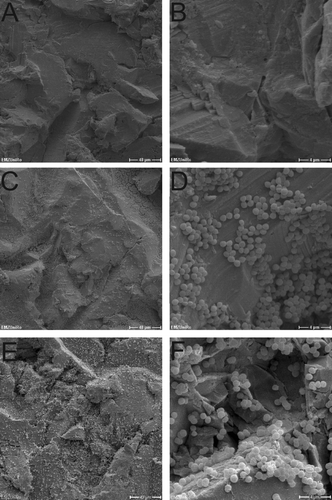
Efficient standardization of the method was achieved by the use of 24-well plates. Metal disk size as well as bacterial inoculation size were constant under standard conditions. The well-position of the different experimental samples did not influence the experimental outcome (data not shown). Thus, for simplicity, a uniform set-up as shown in Supplemental [Supplementary material is available via a multimedia link on the online article webpage] was used. SEM samples were always run on the same plate as the corresponding cell count determination samples. With this set-up, the media, strains, and metal-disks could be varied. It should be stressed that even though the system represents a batch reactor with no flux of nutrients or waste, high bacterial survival rates were observed under the conditions tested here. However, using an expanded set-up, bacterial long-term survival on selected materials could potentially be tested in flow-chambers mimicking the liquid exchange situation in real-live surgical sites.
Attenuation of the antibacterial effects of Cu2 + ions by inter-metallic Ti-Cu films
The effect of copper ions on biofilm formation was analysed by using pure Cu specimens compared to inter-metallic Ti-Cu films prepared by plasma-assisted magnetron sputtering (dual high-power impulse magnetron sputtering, dual-HiPIMS). According to the measurements made, copper was swiftly (within 24 h) and completely released from the dual-HiPIMS film (). Thus, a cytotoxic effect of the Ti-Cu films is mediated by copper ion release into the culture medium. To study the temporal dynamics of biofilm formation under the different conditions, planktonic growth was again monitored, as well as biofilm development of S. epidermidis and S. aureus for 10 days.
Figure 4. Copper release from dual-HiPIMS specimens in DMEM over time as measured by atomic absorption spectrometry.
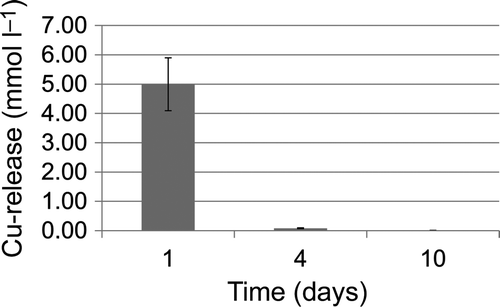
There was no detectable planktonic growth in the presence of pure Cu disks (A and B). For dual-HiPIMS, planktonic growth was initially repressed by copper released from the specimens. S. epidermidis reached maximal cell counts on day 2 (A), S. aureus at day 7 (B). Later, cell counts decreased and on day 10 no bacterial cells could be detected. Overall, growth in samples containing Cu or dual-HiPIMS disks was not significantly different (P > 0.05, two-tailed U test). The antimicrobial effect of dual-HiPIMS disks on adherent S. epidermis and S. aureus cells was less pronounced than on the planktonic counterparts. Biofilm formation was not detectable on Cu disks (C and D). On dual-HiPIMS, almost all S. aureus cells died within 4 days of exposure to copper. However, persisting S. aureus cells started to re-grow and could be detected on day 10 (D). A possible explanation would be the quantitative release of copper within a short period of time. After that period, bacteria could attach to the surface and grow within the protected environment of the biofilm. It has to be noted that growth did not occur in all samples and that the cell counts were still low. Differences in bacterial cell counts in the presence of Ti-Cu films were not statistically significant in comparison to the experimental set-up with Cu disks (P > 0.05, two-tailed U test). In contrast, bacterial biofilm formation of S. epidermidis was not prevented on dual-HiPIMS (C). However the cell counts were reduced by three to four orders of magnitude compared to Ti-6Al-4V_HA specimens. This effect was statistically significant (P < 0.05, two-tailed U test). The log reduction of recovery is summarized in .
Figure 5. The antimicrobial effect of dual-HiPIMS disks on bacterial growth and biofilm formation. A = S. epidermidis planktonic growth in the presence of Ti-6Al-4V_HA disks, dual-HiPIMS disks, and Cu disks detected by CFU determination of culture supernatants; B = S. aureus planktonic growth under similar conditions; C = S. epidermidis biofilm formation on Ti-6Al-4V_HA disks, dual-HiPIMS disks, and Cu disks detected by sonication of the Ti-6Al-4V_HA specimens followed by CFU determination; D = S. aureus biofilm formation under similar conditions.
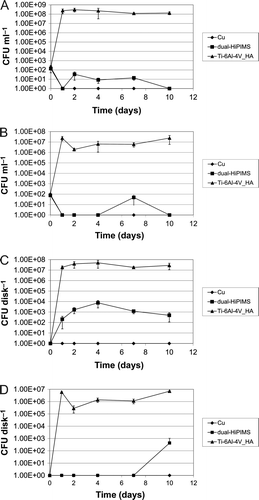
Table 1. Recovery reduction from antimicrobial disks.
Throughout the experiment, copper released from the disc surfaces was present in the medium. Upon sonication of the sample, bacterial cells were probably exposed to low copper ion concentrations. Thus, low cell counts could result from cellular damage caused in the process of sample preparation. To exclude this possibility, biofilm behaviour on copper-releasing specimens was directly observed by SEM. The surface of all Cu disks and Ti-Cu films was characterized by rosette-shaped structures (Supplementary and [Supplementary material is available via a multimedia link on the online article webpage]; and ), probably caused by crystallization of mixed salts with a low solubility, including copper(II) and phosphate ions. SEM analyses confirmed that neither strain was able to colonize the copper surfaces (Supplementary and [Supplementary material is available via a multimedia link on the online article webpage]).
Figure 6. SEM micrographs of dual-HiPIMS disks with S. epidermidis. A, B = day 0; C, D = day 2; E, F = day 10. Scale bars: A, C, E = 40 μm; B, D, F = 4 μm.
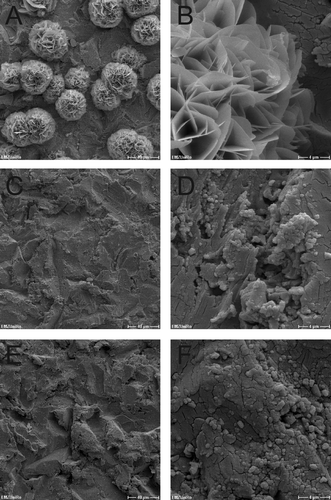
Figure 7. SEM micrographs of dual-HiPIMS disks inoculated with S. aureus. A, B = day 0; C, D = day 2; E, F = day 10. Scale bars: A, C, E = 40 μm; B, D, F = 4 μm.
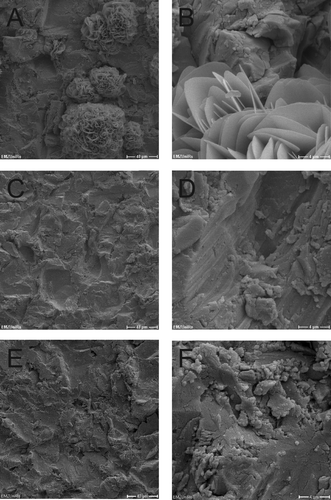
Following inoculation of dual-HiPIMS specimens, single bacterial cells were rarely detectable by SEM ( and ). On day 10 low amounts of bacterial masses were detected on the dual-HiPIMS specimens ( and ). Bacterial cells were attached to the surface but the biofilm structures appeared to be amorphous and sponge-like, probably due to the high content of cell debris and extracellular matrix. Comparison of viability counts of adherent bacteria with SEM pictures shows that differences in the degree of biofilm development can be monitored by simple cell counts. This allows the setting up of simple medium throughput, half automated screens of different implant materials for their antimicrobial properties. In the ‘proof of principle‘ presented here, the authors were able to distinguish the impact of different metal discs on bacterial growth and the differential reaction of two different infection relevant strains.
Discussion
Postoperative implant-associated infections are comparatively rare but devastating complications may follow orthopaedic surgery. Biofilm forming bacteria cause persistent infections that are difficult to treat with anti-microbial regimens, because the extracellular matrix acts as a physical barrier preventing the penetration of antibiotics into the biofilm (Fux et al. Citation2005). The usefulness of prophylactic prescription of antibiotics for patients with endoprosthetic joints following the surgical procedure is still under debate (Kuong et al. Citation2009). To prevent bacterial colonization of implants, the design of novel implant materials should aim at the implementation stage of antibacterial materials while displaying a high degree of eukaryotic cytocompatibility. The surface of such implants should inhibit bacterial growth and biofilm formation, thus preventing early and delayed infections at the implant site. In order to be able to test new potential implant materials efficiently, both a robust and a sensitive repeatable screening tool for bacterial biofilm formation is needed.
The results show that the authors were able to establish a method that allows quantifying the growth of biofilm forming bacteria on potential implant material. This method was capable of differentiating between planktonic growth in the presence of metallic specimens and bacterial cells growing organized in a biofilm (A and B). Quantification of bacterial CFU proved to be repeatable (Supplementary [Supplementary material is available via a multimedia link on the online article webpage]) and corresponded to the appearance of metal specimens in the SEM analyses (, 3, 6, 7). Thus, in the future, SEM is not required for the initial screening of antimicrobial effects of implant materials with the method developed. However, microscopy complements the method for a more thorough characterization of novel materials. While SEM images are a fairly good representation of the 3 D structure, it has to be kept in mind that changes in the ultrastructure are inevitable during the preparation of the samples. As an alternative, visualization of biofilm formation on the specimens by live-dead staining could be employed. This method would provide an additional measure for quantification of living bacteria and additional information about the biofilm structure. The protocols with S. epidermidis and S. aureus cover two of the most relevant pathogenic species responsible for infections of total joint replacements. The method works in medium generally used for tissue culture. In the future, establishment of simultaneous examination of bacterial and host cell growth on the respective implant material either in parallel or in co-culture formats could be tried.
Biofilm formation is a dynamic process dependent on a variety of parameters including implant surface, accurate hygiene measures during surgery (Matthews et al. Citation2009), the genetic properties of the infectious agent (Handke et al. Citation2004), and the susceptibility of the patient. Little is known about the clinical establishment of device related infection. In mice, the minimal inoculum required for infection of titanium implants varied between 102 and 104, depending on the strain used (Hudetz et al. Citation2008). Even higher CFUs were required for postoperative infections. Similarly, only a 25% infection rate of stainless-steel implants was observed in a rat model using bacterial suspensions with a low count (105 CFU) introduced at surgery, whereas a higher count (106 CFU) led to a 75% infection rate (Monzon et al. Citation2002). Additionally, for effective treatment and prevention, the time course of biofilm development on orthopaedic devices should be taken into account (Jaeger et al. Citation2006; Kajiyama et al. Citation2009).
The present study shows that temporally limited release of substances from novel implant material, which leads to initially reduced bacterial counts, will influence biofilm formation kinetics (D). Almost all S. aureus biofilm cells died within 4 days of exposure to copper. However, on the 10th day of cultivation a few persisting S. aureus biofilm bacteria re-grew to lesser bacterial counts than observed for S. epidermidis, probably because of low amounts of the antimicrobial (Cu) agent after its absorption by the initially seeded bacteria. Thus, even though bacterial counts on Ti-6Al-4V_HA specimens seemed to reach a maximum at days 1 to 2, it is important to monitor bacterial growth on test materials for an extended period of time. In certain circumstances, antimicrobial effects of the implant material can delay bacterial growth and biofilm formation rather than eradicate the infectious agents.
The antibacterial effect of copper and its influence on biofilm formation is well established (Baker et al. Citation2010). Even though copper is a trace element and Cu2+ ions are required for the activity of a number of enzymes, higher concentrations of copper are toxic for any type of cells. Copper is capable of generating hydroxyl radicals in a Fenton-like process. This observation led to the assumption that oxidative DNA damage represents a major component of copper toxicity (Gunther et al. Citation1995). The present results show that pure copper disks efficiently prevented colonization by S. epidermidis and S. aureus, whereas Ti-Cu films showed a modified antibacterial effect both on growing and mature biofilms. The short period of copper release also influences the growth of osteoblastic cells on the surface. It has been previously shown in SEM images (Stranak et al. Citation2011) that the vitality of osteoblastic cells significantly increases after rinsing for 24 h. Following the total release of copper from the Ti-Cu film the vitality of exposed human osteoblast MG-63 cells increased significantly. An initial cytotoxic effect followed by the growth of osteoblastic cells was demonstrated. Materials with initial antimicrobial effect, which allow consecutive growth of osteoblastic cells, could be used in joint replacement surgery to reduce the possibility of infection and to increase adoption of the implants.
In conclusion, the authors would like to stress that they were able to monitor the development of bacterial biofilm formation by simply removing bacterial cells from the respective surface and analyzing the viability count. The results were repeatable, and the bacterial cell counts visually corresponded well to the SEM observation. Bacterial growth behavior could be distinguished on different surfaces. Differential susceptibility of S. epidermidis and S. aureus to thin Ti-Cu films could be detected by delineation of the initial response and the overall growth dynamics of the two strains.
The method described here has already been used successfully to evaluate the use of Ti-Cu films as potential implant material. The assay is now set up for 24-well tissue culture plates. It could be further developed to use 96-well formats in order to perform semi-automatic medium-throughput screens. To increase the sensitivity of the analysis, a filter based approach could be added to the protocol allowing for the detection of low bacterial numbers. Initial screens are inexpensive, do not need complementary SEM analyses, and could be reduced to time points covering days 1, 4, and 10. Interesting candidates could then be re-screened on days 1–10, analyzed by SEM, and further tested in rinsing experiments. The use of tissue culture medium will allow studying host-cell-bacteria interactions on potential implant materials.
gbif_a_671305_sup_25175035.pdf
Download PDF (680.1 KB)Acknowledgements
The authors thank L. Middelborg for excellent technical assistance. They also thank G. Fulda and W. Labs from the Electron Microscopic Centre of the Medical Faculty of the University of Rostock for their technical assistance. KA, CZ, and VS were financially supported by the BMBF program Campus PlasmaMed (sub-project PlasmaImp, 13N9775).
References
- Baker , J , Sitthisak , S , Sengupta , M , Johnson , M , Jayaswal , RK and Morrissey , JA . 2010 . Copper stress induces a global stress response in Staphylococcus aureus and represses sae and agr expression and biofilm formation . Appl Environ Microbiol , 76 : 150 – 160 .
- Barth , E , Myrvik , QM , Wagner , W and Gristina , AG . 1989 . In vitro and in vivo comparative colonization of Staphylococcus aureus and Staphylococcus epidermidis on orthopaedic implant materials . Biomaterials , 10 : 325 – 328 .
- Bjerkan , G , Witso , E and Bergh , K . 2009 . Sonication is superior to scraping for retrieval of bacteria in biofilm on titanium and steel surfaces in vitro . Acta Orthop , 80 : 245 – 250 .
- Brown , MR , Allison , DG and Gilbert , P . 1988 . Resistance of bacterial biofilms to antibiotics: a growth-rate related effect? . J Antimicrob Chemother , 22 : 777 – 780 .
- Ceri , H , Olson , ME , Stremick , C , Read , RR , Morck , D and Buret , A . 1999 . The Calgary Biofilm Device: new technology for rapid determination of antibiotic susceptibilities of bacterial biofilms . J Clin Microbiol , 37 : 1771 – 1776 .
- Choong , PF , Dowsey , MM , Carr , D , Daffy , J and Stanley , P . 2007 . Risk factors associated with acute hip prosthetic joint infections and outcome of treatment with a rifampinbased regimen . Acta Orthop , 78 : 755 – 765 .
- Christensen , GD , Baldassarri , L and Simpson , WA . 1995 . Methods for studying microbial colonization of plastics . Methods Enzymol , 253 : 477 – 500 .
- Costerton , JW , Stewart , PS and Greenberg , EP . 1999 . Bacterial biofilms: a common cause of persistent infections . Science , 284 : 1318 – 1322 .
- Coventry , MB . 1975 . Treatment of infections occurring in total hip surgery . Orthop Clin North Am , 6 : 991 – 1003 .
- Del Pozo , JL and Patel , R . 2009 . Clinical practice. Infection associated with prosthetic joints . N Engl J Med , 361 : 787 – 794 .
- Feng , Y , Cao , C , Li , BE , Liu , XY and Dong , YQ . 2008 . Primary study on the antibacterial property of silver-loaded nano-titania coatings . Zhonghua Yi Xue Za Zhi , 88 : 2077 – 2080 .
- Fux , CA , Costerton , JW , Stewart , PS and Stoodley , P . 2005 . Survival strategies of infectious biofilms . Trends Microbiol , 13 : 34 – 40 .
- Gagliardi , M , Silvestri , D , Cristallini , C , Guadagni , M , Crifaci , G and Giusti , P . 2010 . Combined drug release from biodegradable bilayer coating for endovascular stents . J Biomed Mater Res B Appl Biomater , 93 : 375 – 385 .
- Gollwitzer , H , Ibrahim , K , Meyer , H , Mittelmeier , W , Busch , R and Stemberger , A . 2003 . Antibacterial poly(D,L-lactic acid) coating of medical implants using a biodegradable drug delivery technology . J Antimicrob Chemother , 51 : 585 – 591 .
- Gristina , AG and Costerton , JW . 1985 . Bacterial adherence to biomaterials and tissue. The significance of its role in clinical sepsis . J Bone Joint Surg Am , 67 : 264 – 273 .
- Gunther , MR , Hanna , PM , Mason , RP and Cohen , MS . 1995 . Hydroxyl radical formation from cuprous ion and hydrogen peroxide: a spin-trapping study . Arch Biochem Biophys , 316 : 515 – 522 .
- Handke , LD , Conlon , KM , Slater , SR , Elbaruni , S , Fitzpatrick , F , Humphreys , H , Giles , WP , Rupp , ME , Fey , PD and O‘Gara , JP . 2004 . Genetic and phenotypic analysis of biofilm phenotypic variation in multiple Staphylococcus epidermidis isolates . J Med Microbiol , 53 : 367 – 374 .
- Harrison , JJ , Stremick , CA , Turner , RJ , Allan , ND , Olson , ME and Ceri , H . 2010 . Microtiter susceptibility testing of microbes growing on peg lids: a miniaturized biofilm model for high-throughput screening . Nat Protoc , 5 : 1236 – 1254 .
- Heidenau , F , Mittelmeier , W , Detsch , R , Haenle , M , Stenzel , F , Ziegler , G and Gollwitzer , H . 2005 . A novel antibacterial titania coating: metal ion toxicity and in vitro surface colonization . J Mater Sci Mater Med , 16 : 883 – 888 .
- Hellmann , M , Mehta , SD , Bishai , DM , Mears , SC and Zenilman , JM . 2009 . The estimated magnitude and direct hospital costs of prosthetic joint infections in the United States, 1997 to 2004 . J Arthroplasty 25:766–771 ,
- Hoiby , N , Ciofu , O , Johansen , HK , Song , ZJ , Moser , C , Jensen , PO , Molin , S , Givskov , M , Tolker-Nielsen , T and Bjarnsholt , T . 2011 . The clinical impact of bacterial biofilms . Int J Oral Sci , 3 : 55 – 65 .
- Hudetz , D , Ursic , HS , Harris , LG , Luginbuhl , R , Friederich , NF and Landmann , R . 2008 . Weak effect of metal type and ica genes on staphylococcal infection of titanium and stainless steel implants . Clin Microbiol Infect , 14 : 1135 – 1145 .
- Jaeger , M , Maier , D , Kern , WV and Sudkamp , NP . 2006 . Antibiotics in trauma and orthopedic surgery - a primer of evidence-based recommendations . Injury , 37 ( Suppl 2 ) : S74 – S80 .
- Jose , B , Antoci , V Jr. , Zeiger , AR , Wickstrom , E and Hickok , NJ . 2005 . Vancomycin covalently bonded to titanium beads kills Staphylococcus aureus . Chem Biol , 12 : 1041 – 1048 .
- Kajiyama , S , Tsurumoto , T , Osaki , M , Yanagihara , K and Shindo , H . 2009 . Quantitative analysis of Staphylococcus epidermidis biofilm on the surface of biomaterial . J Orthop Sci , 14 : 769 – 775 .
- Kuong , EE , Ng , FY , Yan , CH , Fang , CX and Chiu , PK . 2009 . Antibiotic prophylaxis after total joint replacements . Hong Kong Med J , 15 : 458 – 462 .
- Kwok , CS , Horbett , TA and Ratner , BD . 1999 . Design of infection-resistant antibiotic-releasing polymers. II. Controlled release of antibiotics through a plasma-deposited thin film barrier . J Control Release , 62 : 301 – 311 .
- Li , B , Liu , X , Cao , C , Dong , Y and Ding , C . 2009 . Biological and antibacterial properties of plasma sprayed wollastonite/silver coatings . J Biomed Mater Res B Appl Biomater , 91 : 596 – 603 .
- Li , D , Gromov , K , Soballe , K , Puzas , JE , O‘Keefe , RJ , Awad , H , Drissi , H and Schwarz , EM . 2008 . Quantitative mouse model of implant-associated osteomyelitis and the kinetics of microbial growth, osteolysis, and humoral immunity . J Orthop Res , 26 : 96 – 105 .
- Matthews , PC , Berendt , AR , McNally , MA and Byren , I . 2009 . Diagnosis and management of prosthetic joint infection . BMJ , 338 : 1378 – 1383 .
- Monzon , M , Garcia-Alvarez , F , Lacleriga , A and Amorena , B . 2002 . Evaluation of four experimental osteomyelitis infection models by using precolonized implants and bacterial suspensions . Acta Orthop Scand , 73 : 11 – 19 .
- Phillips , JE , Crane , TP , Noy , M , Elliott , TS and Grimer , RJ . 2006 . The incidence of deep prosthetic infections in a specialist orthopaedic hospital: a 15-year prospective survey . J Bone Joint Surg Br , 88 : 943 – 948 .
- Pratten , J and Ready , D . 2010 . Use of biofilm model systems to study antimicrobial susceptibility . Methods Mol Biol , 642 : 203 – 215 .
- Rogers , GB , Carroll , MP and Bruce , KD . 2011 . Enhancing the utility of existing antibiotics by targeting bacterial behaviour? . Br J Pharmacol 165:845–857 ,
- Rupp , ME and Archer , GL . 1994 . Coagulase-negative staphylococci: pathogens associated with medical progress . Clin Infect Dis , 19 : 231 – 243 .
- Shirai , T , Tsuchiya , H , Shimizu , T , Ohtani , K , Zen , Y and Tomita , K . 2009 . Prevention of pin tract infection with titanium-copper alloys . J Biomed Mater Res B Appl Biomater , 91 : 373 – 380 .
- Stewart , PS and Costerton , JW . 2001 . Antibiotic resistance of bacteria in biofilms . Lancet , 358 : 135 – 138 .
- Stranak , V , Wulff , H , Rebl , H , Zietz , C , Arndt , K , Bogdanowicz , R , Nebe , B , Bader , R , Podbielski , A , Hubicka , Z and Hippler , R . 2011 . Deposition of thin titanium-copper films with antimicrobial effect by advanced magnetron sputtering methods . J MSEC , 31 : 1512 – 1519 .
- Uckay , I , Lubbeke , A , Emonet , S , Tovmirzaeva , L , Stern , R , Ferry , T , Assal , M , Bernard , L , Lew , D and Hoffmeyer , P . 2009 . Low incidence of haematogenous seeding to total hip and knee prostheses in patients with remote infections . J Infect , 59 : 337 – 345 .
- Zietz , C , Fritsche , A , Finke , B , Stranak , V , Haenle , M , Hippler , R , Mittelmeier , W and Bader , R . 2012 . Analysis of the release characteristics of Cu-treated antimicrobial implant surfaces using atomic absorption spectrometry . Bioinorg Chem Appl , 2012 : 850390
- Zimmerli , W . 2005 . Implant infections. What must an internist know? . Internist (Berl) , 46 : 652 – 658 .
- Zimmerli , W , Trampuz , A and Ochsner , PE . 2004 . Prosthetic-joint infections . N Engl J Med , 351 : 1645 – 1654 .
Supplemental Figure 1. Principal organization of the 24-well plate for the experiments presented in this work. A, B = Bacterial growth in the presence of Ti-6Al-4V_HA disks. Comparison of growth in CB/HS medium with growth in DMEM tissue culture medium. Plates were photographed on days 2 (A) and 10 (B). Lanes 1 and 2: test of S. epidermidis in CB/HS (lane 1) and in DMEM (lane 2). Lanes 3 and 4: medium without inoculation of bacteria, CB/HS (lane 3) and in DMEM (lane 4). Lanes 5 and 6: test of S. aureus in CB/HS (lane 5) and in DMEM (lane 6). The wells from row A were taken for SEM analyses, wells from rows B–D represent the three technical replicates of each plate. C, D = Antimicrobial effects of copper-containing disks. Plates were photographed on days 2 (C) and 10 (D). Lanes 1–3, growth of S. epidermidis in the presence of Ti-6Al-4V_HA disks (1), Cu-disks (2), and Ti-Cu films (3). Lanes 4–5, growth of S. aureus in the presence of Ti-6Al-4V_HA disks (4), Cu-disks (5), and Ti-Cu films (6). Wells in row A1-A3 were used for SEM of S. epidermidis samples. Wells A4-A6 contained medium without inoculation of bacteria. This order was reversed on plates with SEM samples for S. aureus. Rows B–D represent the three technical replicates of each plate.
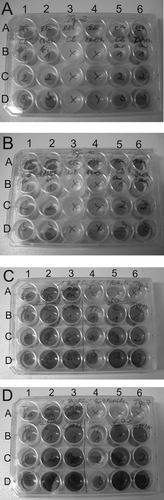
Supplemental Figure 2. Repeatability of viability count determination of adherent bacteria. Bacterial growth in the presence of Ti-6Al-4V_HA disks. Formation of S. epidermidis biofilms in DMEM on Ti-6Al-4V_HA disks detected by sonication of the Ti-6Al-4V_HA specimens followed by CFU determination. The results from four independent experiments are shown. The error bars represent three technical replicates.
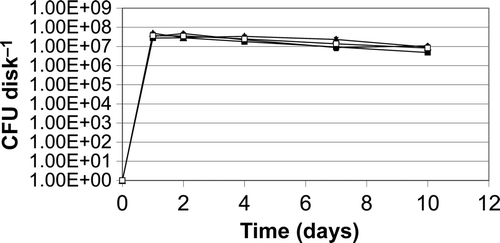