Abstract
Antimicrobial peptides (AMPs) have a broad spectrum of activity and unspecific mechanisms of action. Therefore, they are seen as valid alternatives to overcome clinically relevant biofilms and reduce the chance of acquired resistance. This paper reviews AMPs and anti-biofilm AMP-based strategies and discusses ongoing and future work. Recent studies report successful AMP-based prophylactic and therapeutic strategies, several databases catalogue AMP information and analysis tools, and novel bioinformatics tools are supporting AMP discovery and design. However, most AMP studies are performed with planktonic cultures, and most studies on sessile cells test AMPs on growing rather than mature biofilms. Promising preliminary synergistic studies have to be consubstantiated and the study of functionalized coatings with AMPs must be further explored. Standardized operating protocols, to enforce the repeatability and reproducibility of AMP anti-biofilm tests, and automated means of screening and processing the ever-expanding literature are still missing.
Introduction
Biofilms are complex, localized and fixed networks of microbial cells enclosed in a self-produced polymeric matrix, whose formation undergoes essentially four typical stages, namely (1) adherence of planktonic cells to the abiotic surface or tissue, (2) growth of the attached cells and production of the extracellular polymeric protective matrix (mainly proteins, polysaccharides and extracellular DNA resulting from autolysis (eDNA), (3) biofilm maturation involving the development of water channels and specialized zones, and (4) dispersion of cells and/or parts of the biofilm with subsequent colonization of other locations (Costerton et al. Citation1999; Donlan and Costerton Citation2002; Fey Citation2010; Høiby et al. Citation2010). The architecture of a mature biofilm is variable, ranging from flat homogenous layers of cells to highly organized cell clusters with a mushroom shaped structure containing water-filled channels (Wimpenny et al. Citation2000).
Even though there have been important research advances in the biofilm area, biofilm control is still an open and pivotal research field. Approximately 80% of human bacterial infections are caused by biofilms (Harro et al. Citation2010), mainly health care associated infections related to the implant of medical devices, eg urinary catheters, intravascular catheters and prosthetic heart valves. As such, microbial adhesion onto surfaces and the subsequent formation of biofilms are critical concerns for many biomedical applications (Donlan and Costerton Citation2002; Fey Citation2010). Indeed, the increasing resistance of biofilms to traditional antimicrobial treatments is considered the major cause of dissemination of antibiotic resistance in the nosocomial infections (Fey Citation2010; Spížek et al. Citation2010).
Biofilm resistance has been related to several factors (Hall-Stoodley and Stoodley Citation2009; Høiby et al. Citation2010; Corbin et al. Citation2011), such as (1) the higher frequency of mutation and horizontal gene transmission found in biofilms when compared to planktonic bacteria, which explains the rapid development of antibiotic resistance in biofilms; (2) the appearance of nutrient depleted zones throughout biofilms, due to oxygen and nutrient gradients, which cause bacteria to enter into a stationary phase-like dormancy and not be affected by antimicrobials; (3) the development of oxidative stress, caused by an imbalance between the formation of reactive oxygen species (ROS) and the antioxidant system, that increases mutability in biofilms; and (4) the delay of antibiotic penetration into the matrix of the biofilm, which contains polymers that bind to antibiotics and hinder their action, and antibiotic-degrading enzymes that deactivate them.
The difficulty of successfully treating biofilm-associated infections and the increasing resistance of microbes to traditional treatments call for the discovery of compounds with novel mechanisms of action. Most of the antimicrobial products that have been developed are derivatives of already known compounds and target the same resistance mechanism, so their action can only be somewhat better. Now, research is drifting towards the discovery of non-traditional sources of antimicrobials, microbial genome sequencing focused on antibiotic gene expression, metagenomics, and investigation of new targets with respect to pathogenic bacteria. Within this scope, natural products, namely antimicrobial peptides (AMPs), stand out because they have a much higher hit rate in high-throughput screens than the combinational libraries of traditional antimicrobials. Moreover, natural products are usually much more complex than synthetic products and present scaffolds with viable and biologically validated starting points for the design of chemical libraries (Spížek et al. Citation2010).
The following sections describe AMPs and their application to biofilm prophylaxis and therapeutics. Emphasis is given to the main sources and categories of AMPs, their common anti-biofilm mechanisms of action, and customized bioinformatic approaches.
Sources of antimicrobial peptides
AMPs are short-length peptide antibiotics (between 15 and 30 amino acids), the majority of which are cationic, amphipathic, gene-encoded and directed to the cell membrane (Rossi et al. Citation2008; Melo et al. Citation2009; Splith and Neundorf Citation2011). AMPs are usually co-expressed in groups that act together (Lai and Gallo Citation2009), but despite their similarities, AMP sequences vary greatly. Usually, AMPs are classified as α-helical, β-sheeted, extended and looped (Melo et al. Citation2009) or ribosomally and non-ribosomally synthesized (Rossi et al. Citation2008).
AMPs come from a variety of sources and in many forms. Their widespread distribution throughout the animal and plant kingdoms suggests that AMPs play a fundamental role in the evolution of complex multicellular organisms. Despite their ancient lineage, AMPs have remained effective defensive weapons (Zasloff Citation2002). Indeed, it has been proposed that AMPs and AMP-directed resistance mechanisms have co-evolved, leading to a host-pathogen balance that has shaped the existing AMP portfolio (Peschel and Sahl Citation2006).
AMPs are part of the innate immune system of animals and plants, but can also be found in bacteria and fungi (Rossi et al. Citation2008; Splith and Neundorf Citation2011). Mammalian AMPs are generally expressed and easily induced in epithelial surfaces to repel assault by bacteria, viruses, fungi and parasites (Lai and Gallo Citation2009). AMPs have also been found in the glandular cells of amphibian skin, fish and most classes of invertebrates. In plants, AMPs help in the adaptation to stressful environmental conditions. For example, plant cells act as recognition sites against pathogen-derived metabolites (elicitors), leading to the accumulation of AMPs in the affected plant tissue (Kido et al. Citation2010). Additionally, bacteria and fungi produce AMPs as a defense mechanism and as a means of gaining competitive advantage against other microorganisms, sometimes of the same species (Sang and Blecha Citation2008).
Synthetic AMPs, produced by de novo synthesis or by modification of existing AMPs, emerged as an alternative to reduce production costs (Wimley and Hristova Citation2011). Recently, some reviews (Vooturi and Firestine Citation2010; Giuliani and Rinaldi Citation2011) have reported the engineering of AMP mimetics or peptidomimetics, ie non-peptide molecules aimed at retaining and improving the basic features of AMPs.
A resume of the sources and classifications of the various AMPs is available in .
Table 1. AMP sources and classes.
Mechanisms of action of antimicrobial peptides
AMPs have been recognized as promising candidates for replacing classical antibiotics () due to their multiple mechanisms of action and low specificity in terms of molecular targets, which reduces the chance of acquired resistance (Zasloff Citation2002; Beckloff et al. Citation2007). Moreover, compared with conventional antimicrobials, which are generally active only against bacteria or fungi, AMPs exert activity against a broad spectrum of microorganisms, such as both Gram-negative and Gram-positive bacteria, including drug-resistant strains, parasites, enveloped viruses and even some cancer cells (Sang and Blecha Citation2008; Splith and Neundorf Citation2011; Wimley and Hristova Citation2011). AMPs are also cell specific and are able to distinguish host from non-host cells based on their charge (Beckloff et al. Citation2007). Besides their antimicrobial action, AMPs can also influence processes which support antimicrobial action, like cytokine release, chemotaxis, antigen presentation, angiogenesis and wound healing (Lai and Gallo Citation2009).
Figure 1. General statistics on the number of publications on AMPs (blue bars) and AMP applications to biofilms (red bars) in PubMed. The word cloud highlights research areas where these studies are most relevant.
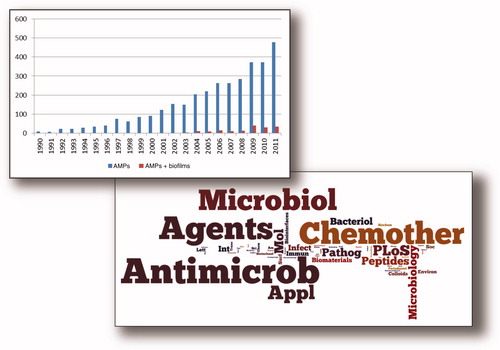
Conventional antibiotics usually act by inhibition of cell wall synthesis or DNA, RNA and protein synthesis (Sang and Blecha Citation2008; Chau Citation2010). Most AMPs permeabilize microbial membranes, inducing either a large-scale failure or small defects that dissipate the transmembrane potential, which results in cell death (Sang and Blecha Citation2008; Wimley and Hristova Citation2011). This mechanism of action does not depend on the recognition of chiral targets and, therefore, all D-enantiomers are equally active, giving AMPs a broad action spectrum (Podda et al. Citation2006).
The mechanisms of action of AMPs are divided into pore and non-pore models (Wimley and Hristova Citation2011). Pore models account for the formation of membrane-spanning pores, namely the ‘barrel stave pore model’ (Rapaport and Shai Citation1991), in which AMPs interact to form a hydrophilic channel, and the ‘toroidal pore model’ (Ludtke et al. Citation1996), in which AMPs affect the curvature of the membrane. In turn, non-pore models comprise: the ‘carpet model’ (Gazit et al. Citation1996), which is the most cited model and accounts for the parallel deposition of AMPs on the membrane, causing global bilayer destabilization due to a detergent-like effect; the ‘detergent model’ (Ostolaza et al. Citation1993) that explains catastrophic collapse of the membrane using high concentrations of AMPs; the ‘molecular shape models’ (Bechinger and Lohner Citation2006), in which AMP-lipid interactions can be portrayed with phase diagrams; the ‘lipid clustering model’ (Epand and Epand Citation2009), in which AMPs induce lipid phase separation; the ‘sinking raft model’ (Pokorny et al. Citation2002), in which AMP activity is described in terms of binding, insertion and perturbation; and the ‘interfacial activity model’ (Rathinakumar and Wimley Citation2008), which is used to explain, predict and engineer the activity of AMPs. All the aforementioned models imply the need to reach a certain threshold concentration of AMPs in the membrane prior to disruption (Melo et al. Citation2009). Biomembrane interaction studies with model bacterial and mammalian lipid membranes are very important for determining the mechanisms of action of membrane active peptides (Gao et al. Citation2012).
Some AMPs act by alternative means, like binding to DNA, inhibiting cell wall, DNA, RNA and protein synthesis, and autolysin and inhibiting enzyme activity (Sang and Blecha Citation2008). The type of mechanism of action of AMPs can dictate their application fields. For example, it has been noticed that AMPs targeted at the membrane are better suited for use in surface coatings than AMPs that act at an intracellular level (Bagheri et al. Citation2012).
More details about the mechanisms of action of AMPs are available in the reviews of Melo et al. (Citation2009), Nguyen et al. (Citation2011), Park et al. (Citation2011), Splith and Neundorf (Citation2011) and Wimley and Hristova (Citation2011).
Anti-biofilm antimicrobial peptide strategies
Biofilm control can be achieved in three ways, namely (1) reduction of the planktonic population, (2) prevention of the initial adhesion of cells to the surface, and (3) removal of the established biofilm. Studies on biofilm-forming bacteria or yeasts in the planktonic state may open routes to the first strategy. However, in vivo application of the first strategy is quite complicated, given that the planktonic population in the body is widespread and identification of the presence of the planktonic biofilm-forming bacteria is difficult, so microbial adhesion must be prevented as the next step.
Conceptually, the easiest method for preventing microbial attachment is by pre-treating the surfaces. This can be achieved by impregnating the surface with an antimicrobial agent or using functionalized coatings that allow a localized antimicrobial delivery (Zilberman and Elsner Citation2008; Shukla et al. Citation2010; Yala et al. Citation2011). As reviewed by Glinel et al. (Citation2012), several AMP-based coatings have been tested successfully.
When surface pre-treatments are not effective, biofilms may form. Strategies based on the administration of antimicrobials to the infected live tissue (antibiotic treatments) or the non-living surface (disinfectant treatments) must then be applied to kill the biofilm-growing microorganisms. A summary of the latest biofilm control studies using AMPs is depicted in .
Table 2. Recent applications of AMPs in the control of biofilms.
Analysis of shows that the AMPs tested on biofilms come from various natural sources, such as humans (AMP-IBP5; HBD3; LL-37; α-MSH), other mammals (BMAP-28; cathelicidin WAM1), amphibians (aurein 2.5; magainin I; phylloseptin-1), fish (chrysophsin-1; pleurocidin), arthropods (tachyplesin III), bacteria (gramicidin A; lacticin 3147; nisin) and plants (Tn-AFP1). Non-natural AMPs are classified into mimetics (peptoid 1; peptoid 1-C134mer; (RW)4D) and synthetic (F2,5,12W; KSL; PTP-7; Tet213; SAMPs Ltx5, Ltx9 and Ltx10; omiganan pentahydrochloride; STAMPs C16G2, M8G2, C16-33, M8-33 and G10KHc). A substantial part of the AMPs tested is synthetic, which means that improving the optimal performance of AMPs is nowadays becoming an important issue. Most of the microorganisms tested are bacteria, probably due to their ubiquity in Nature and their frequent association with infectious diseases and biofilms.
It is also noteworthy that a substantial number of biofilm-related studies, as seen in , cover mainly biofilm growth in the presence of AMPs, ie prophylactic strategies meant to prevent biofilm formation, rather than testing AMPs against pre-established biofilms, ie therapeutic strategies meant to treat existing biofilms. This suggests that prevention of biofilm formation is possibly the current favorite research strategy in the combat of nosocomial infections. However, more work must be done in order to evaluate the anti-biofilm efficacy of AMPs on mature biofilms. Some recent, successful studies on the development of AMP-mediated anti-biofilm prophylactic and therapeutic strategies can be found in .
One of the characteristics that appears to be linked to the anti-biofilm efficacy of some AMPs is their dual capacity to act both on the cytoplasmic membrane and on intracellular targets, once they have entered the cell. For example, it is thought that the synthetic AMP meta-phenylene ethynylene (mPE), based on magainin and active at nanomolar concentrations against biofilms of Streptococcus mutans, acts both as a membrane-active molecule, inhibiting lipopolysaccharides (LPSs), similar to magainin, and as an intracellular antibiotic by binding to DNA at equimolar ratios (Beckloff et al. Citation2007). Another example is pleurocidin, which is thought to inhibit nucleic acid and protein synthesis without damaging the cytoplasmic membrane of Escherichia coli at low concentrations (Patrzykat et al. Citation2002), but it is able to cause membrane leakage and pore-like channels at higher concentrations (Mason et al. Citation2006).
Some studies have also reported AMP activity against biofilms at lower concentrations than those required for killing planktonic cells. This is the case for the synthetic AMP NA-CATH:ATRA1-ATRA1 and the natural AMP LL-37, both from the cathelicidin family, that are effective against Staphylococcus aureus and Pseudomonas aeruginosa biofilms, respectively. These AMPs are thought to act internally on the bacterial cells, affecting gene expression essential for the development of biofilms (Overhage et al. Citation2008; Dean et al. Citation2011). In P. aeruginosa, the AMP LL-37 also alters the expression of biofilm related genes, such as Type IV pili, rhamnolipid and Las quorum sensing systems at sub-antimicrobial levels, and genes associated with the assembly of flagella, involved in initial adherence during biofilm formation, were found to be down regulated (Overhage et al. Citation2008). LL-37 is also capable of inhibiting initial biofilm attachment (by 58–62%), suggesting that peptides of this kind may be interacting with bacterial adhesins as part of their anti-biofilm mechanism (Dean et al. Citation2011). Another study (de la Fuente-Núñez et al. Citation2012) also showed that the AMP 1037 directly inhibits biofilms by reducing swimming and swarming motilities, stimulating twitching motility, and suppressing the expression of a variety of genes involved in biofilm formation in P. aeruginosa (eg PA2204).
Anti-adhesion may be one of the major anti-biofilm properties of AMPs, which allows them to be used as an effective pre-treatment strategy. The AMP nisin, which is known to interfere with cell wall synthesis and to form membrane pores (Peschel and Sahl Citation2006), retards biofilm formation without inhibiting the growth of S. aureus when immobilized in multi-walled carbon nanotubes (Qi et al. Citation2011). Another example is the cathelicidin-2 derived peptide, F2,5,12W, which suppresses Staphylococcus epidermidis biofilm formation at a concentration four times below the minimal inhibitory concentration (MIC), and this is reflected in decreased initial adhesion of the bacterial cells (Molhoek et al. Citation2011).
Anti-attachment capabilities may be related to binding of DNA also. DNA binding may facilitate the detachment or disruption of biofilm structures, since it has been reported that eDNA is involved in cell–cell attachment, having an important role in cell adhesion to surfaces and cell aggregation (Allesen-Holm et al. Citation2006; Das et al. Citation2010). This is the case for cationic AMPs and peptoids (Lobo et al. Citation2003; Otvos Citation2005; Hale and Hancock Citation2007). For example, the development of P. aeruginosa biofilms is disrupted by the enzyme DNase I (Whitchurch et al. Citation2002).
AMPs may also cause matrix disruption in biofilms. It is thought that the peptoid 1-C134mer, which has an hydrophobic tail and is active against P. aeruginosa biofilms, interacts strongly with and disrupt the hydrophobic matrix due to its surfactant like nature, facilitating deeper penetration (Kapoor et al. Citation2011).
Additionally, AMPs have some organism-specific features. For example, lactoferrin inhibits biofilm formation in P. aeruginosa due to its iron-chelating properties, increasing surface motility and causing the bacterial cells to wander around the surface, forming thin, flat biofilms (Singh et al. Citation2002). In turn, the inhibition of Porphyromonas gingivalis and Prevotella intermedia biofilms by lactoferrin is independent of the iron status of the protein. Lactoferrin may interact with the cell surface of these bacteria and interfere with their adherence (Wakabayashi et al. Citation2009), since this peptide is reported to interfere with the binding of P. intermedia to subepithelial matrix proteins, as well as fibroblasts and epithelial cells (Alugupalli et al. Citation1994; Alugupalli and Kalfas Citation1995).
Biofilm resistance to antimicrobial peptides
Although the development of resistance to AMPs is rare, some studies have reported this phenomenon. The general mechanisms of microbial resistance to AMPs, which are valid both for the planktonic and sessile states, include mutations that affect the structure and charge distribution of the cytoplasmatic membrane, modifications to the lipopolysaccharide structure of Gram-negative bacteria, and active pumping of the AMPs out of the cell (Altman et al. Citation2006). Specifically, it has been reported that Gram-negative bacteria have evolved mechanisms to remodel the composition of the outer membrane through modification of the LPS molecules (Miller et al. Citation2005), which impairs LPS-binding AMPs.
Biofilm structure is another factor correlated with biofilm resistance to AMPs. For instance, the increased survival of E. coli biofilms when treated with colistin is not related directly with biofilm forming ability, but rather to the organization of the biofilm (Folkesson et al. Citation2008). Also, there is some evidence that biofilm formation in E. coli induces tolerance to AMPs due to changes in intra-biofilm physiochemical gradients (Folkesson et al. Citation2008).
AMP activity over intracellular targets is countered by genetic mutations. Interestingly, in S. aureus, the regulatory system GraRS, which is involved in up-regulation of biofilm production, has been reported to mediate the resistance of the planktonic cells to AMPs (Herbert et al. Citation2007). In cystic fibrosis, where P. aeruginosa biofilms cause pneumonia, results show that colistin kills the stalk subpopulation (a deeper layer with low metabolic activity) preferentially, whereas the metabolically active cap-forming subpopulation in the upper layer becomes colistin resistant due to the up-regulation of the pmr and mexAB-oprM genes (Haagensen et al. Citation2007; Pamp et al. Citation2008).
Bioinformatic resources
Bioinformatic approaches are an invaluable asset to attain a thorough understanding of the activity of AMPs and thus exploit their potential as antimicrobial drugs. Current approaches address the screening and in silico modelling of novel AMPs as a means of accelerating the process of antimicrobial drug discovery and design, and the construction of information systems in support of the deposition, curation and comparison of AMP related data, as means of keeping track of the continuous discovery of new AMPs with diverse antimicrobial potential.
A schematic overview of existing tools and resourcesin support of AMP studies is presented in , and details of the different areas of development and application are provided in the next subsections.
Figure 2. Bioinformatics resources for AMP studies. MS = mass spectrometry; LC = liquid chromatography; ANNs = artificial neural networks; HMMs = hidden Markov models; QMs = quantitative matrices; SVMs = support vector machines; RFs = random forests; DA = discriminant analysis; QSAR = quantitative structure–activity relationship.
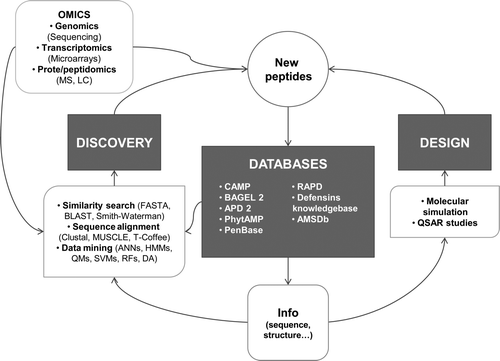
Discovery and classification of AMPs
Several ‘omics’ platforms are addressing the development of computational strategies to identify distinct classes of AMPs (Hammami and Fliss Citation2010; Pestana-Calsa et al. Citation2010). Among them, there are consolidated tools for local and global alignments of DNA and protein sequences such as FASTA (Lipman and Pearson Citation1985), BLAST (Altschul et al. Citation1990), HMMER (Finn et al. Citation2011) and the Smith-Waterman algorithm (Smith and Waterman Citation1981), and data mining tools that apply large-scale computational analysis algorithms to the information saved in genomic and proteomic databases, as well as in published structure–activity data. Indeed, advanced algorithms based in Hidden Markov Models (HMMs) (Eddy Citation1998), Artificial Neural Networks (ANNs) (Hopfield Citation1988), Quantitative Matrices (QMs) (Brusic et al. Citation1998), Support Vector Machines (SVMs) (Zhang Citation2001), Random Forests (RFs) (Breiman Citation2001), Discriminant Analysis (DA) (Lachenbruch and Goldstein Citation1979), Minimum Redundancy Maximum Relevance (mRMR) (Peng et al. Citation2003) and Incremental Feature Selection (IFS) (Liu and Setiono Citation1998) have been successfully applied to AMP data mining.
Some authors have used predictive data mining to assess the antimicrobial potential of new peptides. For example the AMPer method (Fjell et al. Citation2007) recognizes individual classes of AMPs (such as defensins, cathelicidins and cecropins) and discovers novel AMP candidates based on HMMs fed on publicly available data; the BACTIBASE method (Hammami et al. Citation2007, Citation2010) uses HMMs to produce bacteriocin profiles for each known family and the sequence analysis tool HMMER to provide statistical descriptions of family consensus sequences in order to support sequence-based searches on the bacterial families producing bacteriocins; the AntiBP and AntiBP2 methods (Lata et al. Citation2007, Citation2010) predict antibacterial peptides applying ANN, QM and SVM models to the analysis of the N and C terminal residues of proteins; the CAMP method (Thomas et al. Citation2010) uses RF, DA and SVM models to predict the antimicrobial activity of peptide sequences; the BAGEL2 method (de Jong et al. Citation2010) combines HMMs and simple decision rules in the prediction of bacteriocin sub-classes; the DAMPD method (Sundararajan et al. Citation2011) predicts AMPs based on SVMs that can classify peptides into one of 27 AMP families in catalogue; and the tool of Wang et al. (Citation2011b) integrates protein BLAST (BLASTP) and a feature selection method based on mRMR and IFS models to select the optimal features for the prediction of AMPs vs non-AMPs. In addition, Juretić et al. (Citation2009, Citation2011) have been addressing the correlation between the physical characteristics of natural AMPs and high selectivity to generate potential peptide antibiotics not homologous to any existing natural or synthetic AMPs. This has resulted in the creation of a structure-selectivity database (AMPad) of frog-derived, helical AMPs. The AMPA web application ( http://tcoffee.crg.cat/apps/ampa) (Torrent et al. Citation2011) was constructed for assessing the antimicrobial domains of proteins, based on an antimicrobial propensity scale for each amino acid (related to the IC50 values for all amino acid replacements in the AMP bactenecin 2A) and identifying the regions (>12 amino acids in length) located below the threshold, which are considered putative antimicrobial domains. There is also a search method (Fernandes et al. Citation2009) for sequence similarity and physico-chemical properties followed by a fuzzy inference system in order to find AMPs that are more appropriate for certain target domains. Finally, the Antibp server ( http://www.imtech.res.in/raghava/antibp/index.html) (Lata et al. Citation2007) predicts antibacterial peptides in protein sequences, with QM, ANN and SVM based methods using the binary patterns of peptide sequences.
A detailed review on designing AMPs is available (Fjell et al. Citation2012).
Genomics
In genomics, DNA sequencing and mapping constitute a high yield and an useful step for seeking AMP-coding genes (Pestana-Calsa et al. Citation2010; Pestana-Calsa and Calsa Jr. Citation2011). One of the most used methods for AMP discovery is the analysis of homologs of known genes by comparing new sequences with sequences of biochemically characterized AMPs (Hammami and Fliss Citation2010; Pestana-Calsa and Calsa Jr. Citation2011), since the sequence segments with high identity tend to share structure and function. For example: an in silico search based on HMMs (Silverstein et al. Citation2005) supported genome assignment of 317 defensin-like sequences in Arabidopsis thaliana; a novel cathelicidin-like AMP, designated cathelicidin-AM, was identified (Yan et al. Citation2011) by mining the genome of panda using BLAST and the sequence of a dog cathelicidin; and the systematic mining of the Nasonia vitripennis genome (Tian et al. Citation2010), using the tools BLASTP, translated BLAST nucleotide (TBLASTN) and ScanProsite ( http://prosite.expasy.org/scanprosite/scanprosite-doc. html), has delivered a large and diverse peptidome AMP repertoire of defensins, abaecins, hymenoptaecins and tachystatins.
Transcriptomics and proteomics
Transcriptome and proteome profiling offer powerful approaches for studying antimicrobial inhibitory action (Hutter et al. Citation2004; Freiberg et al. Citation2005; Overton et al. Citation2011). It is possible to screen a large number of compounds for antimicrobials, and also to predict their mechanism of action by gene expression profiling (Hammami and Fliss Citation2010). In principle, the mRNA and protein profiles generated in response to the imposition of antimicrobial stress reflect modulation of particular cellular functions, and provide a signature of the type of stress imposed (Overton et al. Citation2011). For example: an integrated transcriptome and proteome profiling of drug exposed bacteria (Overton et al. Citation2011) revealed 22 novel virulence factors from methicillin-resistant S. aureus (MRSA) and novel information on killing mechanisms of the AMP ranalexin; a Super SAGE transcriptional analysis (Kido et al. Citation2010) investigated AMP candidates in Brazilian plants; and a comparative proteomics study (Kim et al. Citation2011) evaluated milk proteome profiles during acute and chronic phases of S. aureus intramammary infection, revealing unique host protein expression profiles depending on the infecting strain and the phase of infection, and implicating the protein CPP3 as a potential AMP.
Peptidomics
Peptidomics addresses the study of small polypeptides, eg by coupling bi- or multidimensional liquid chromatography to mass spectrometry (Pestana-Calsa et al. Citation2010). Thirty-four novel AMPs from skin secretions of Rana nigrovittata were identified by integrating genomics and peptidomics analysis (Ma et al. Citation2010). Also, a functional peptidomics approach (Wang et al. Citation2008), integrating peptidome and transcriptome studies of the defensive skin secretion of the frog Agalychnis callidryas has led to the identification of three new AMPs: DRP-AC4 (dermaseptin), ARP-AC1 (adenoregulin) and CRP-AC1 (caerin). More recently, a peptidomics-based approach (Osaki et al. Citation2011) allowed the identification of the AMP-IBP5, which exhibits antimicrobial activity against Gram-negative and Gram-positive bacteria and fungi at concentrations similar or lower than those of cathelicidin and β-defensin-2. This was accomplished by applying tandem mass spectrometry (MS/MS) techniques to the characterization of a pool of naturally occurring peptides released by exocytosis from cells in culture.
Additionally, peptidomic approaches tackle the study of resistance mechanisms, in support of the development of more sustainable antimicrobial agents (Shen et al. Citation2010). For example, proteins regulating AMP resistance in Vibrio parahaemolyticus have been discovered using membrane subproteome analysis (Shen et al. Citation2010).
Design of antimicrobial peptides
Simulations and structural bioinformatic approaches can help to focus experimental work that, in turn, will eventually guide and verify simulations (Matyus et al. Citation2007). Classical molecular dynamics simulations have been proven useful in describing elements of the mechanism of action of AMPs, including the binding of peptides to the bilayer surface, the properties of surface-bound complexes, peptide insertion, structural modifications of lipid bilayers, and the structure of model pores constructed from AMPs and lipids (Matyus et al. Citation2007). For example, new synthetic cationic peptides with two LPS- and lipid A (LA)-binding sites, similar to AMPs such as protegrin 1, thanatin, and androctonin, were synthesized de novo based on molecular modeling simulation and quantitative structure-activity relationship (QSAR) analysis (Frecer et al. Citation2004).
QSAR analyses, which are used broadly in chemoinformatics to correlate the variation in measured biological activity with the variation in molecular structure, are now being used as a tool for antimicrobial drug discovery (Hammami and Fliss Citation2010). QSAR analyses have been applied successfully to the analysis of AMP data, resulting in a model that quantifies linear sequence patterns (Hilpert et al. Citation2006), contact energy between neighboring amino acids (Jenssen et al. Citation2008) and amphipathicity (Frecer et al. Citation2004). Also, QSAR analyses have been used in the production of lactoferricin, bactenecin, and protegrin derivatives (Hilpert et al. Citation2008), and the optimization of the activity of novispirin G10 (Taboureau et al. Citation2006; Blondelle and Lohner Citation2010). Moreover, a 3D-QSAR analysis of a series of AMPs (Bhonsle et al. Citation2007; Blondelle and Lohner Citation2010) revealed that for any particular AMP, cell selectivity and potency are controlled by the chemical composition of the target cell membrane, and a combined approach of atomic-resolution QSARs with ANNs (Cherkasov et al. Citation2008; Fjell et al. Citation2009; Blondelle and Lohner Citation2010), applied to a large data set of peptides containing high sequence diversity, has shown that the most potent peptides exhibit a high frequency of the amino acids tryptophan, arginine, lysine, isoleucine and leucine.
Information storage and search
A comprehensive database on AMPs with information on their activity would facilitate the study of peptide potential, enabling and promoting sequence-specificity and sequence-activity studies (Hammami et al. Citation2009; Thomas et al. Citation2010). Although many AMPs are now well characterized, much information is still missing or scattered over scientific literature, ie its collection and analysis is troublesome and implies time consuming manual curation.
Available and fully operational databases on AMPs are presented in . Most AMP databases specialize in one type of AMP family, like BAGEL2 (bacteriocins), PhytAMP (plant AMPs), Defensins Knowledgebase (defensins), BACTIBASE (bacteriocins), Peptaibol Database (peptaibols) and PenBase (penaidins). This is due to the diversity of AMPs that are being described and the need to accommodate the most extensive (or application-specific) subclasses into specialized databases. Bioinformatic tools accounting for similarity search (FASTA, BLAST, Smith-Waterman), sequence alignment (ClustalW, T-Coffee and MUSCLE), AMP prediction and physico-chemical profile analysis are present in the majority of the databases described, allowing users easy access to peptide/DNA sequence analysis.
Table 3. Contents and tools (similarity search, sequence alignment, prediction and others) of current AMP-related databases.
Databases covering proteins and antimicrobials are also considered useful resources for AMP related studies. For example: UniProt ( http://www.uniprot. org/), which is a central repository of protein sequence and function data; Protein Data Bank ( http://www.pdb.org/), which covers the processing and distribution of 3D biological macromolecular structure data; CyBase ( http://www.cybase.org.au/), which keeps cyclic protein sequences and structures; AMICBASE ( http://www.reviewscience.com/), which documents the antimicrobial properties of natural compounds; EROP (Endogenous Regulatory OligoPeptide knowledgebase)-Moscow ( http://erop.inbi. ras.ru/index.html) (Zamyatnin Citation1991; Zamyatnin et al. Citation2006), which is a curated oligopeptide (2-50 amino acid residues) sequence database; Norine ( http://bioinfo. lifl.fr/norine/), which is a database for nonribosomal peptides with tools for their analysis; PepBank ( http://pepbank.mgh.harvard.edu/), which is a database on biologically active peptides; PeptideDB database ( http://www.peptides.be/), which assembles all naturally occurring signalling peptides from animal source, which are derived by cleavage from prepropeptide precursor proteins; the Novel Antibiotics DataBase ( http://www.nih.go.jp/∼jun/NADB/search.html), which contains substances reported first in the Journal of Antibiotics; and the Amicbase Drugs-Online 2011 report ( http://www.manetec-52.de/apps/amicbase_drugs-online/base.nsf), which provides antibiotic inhibitory data on licensed drugs in Europe, Japan and the USA.
Current challenges to AMP application
Despite all the aforementioned advantages, most AMP-based strategies present some limitations that must be overcome to achieve widespread implementation of such strategies in biotechnological and clinical settings.
AMP production and application
AMPs are difficult and expensive to obtain in large quantities (Beckloff et al. Citation2007), mainly due to the complex processes needed for their extraction, isolation and purification. While large amounts of AMPs may be applied to a localized external site (as topical agents), the use of AMPs for internal infections raises some problems: (1) their antimicrobial power is reduced in the presence of biological fluids, (2) inadequate safety margins and rapid renal clearance, (3) the non-specific effect of AMPs may compromise the eradication of a specific infectious agent, and (4) some AMPs display toxicity to the host cells and may induce unwanted pro-inflammatory responses (Baltzer and Brown Citation2011; Batoni et al. Citation2011). Thus, efforts are being focused on modifying or synthesizing de novo AMPs that are easier to produce and may overcome some of the limitations referred to above.
Prophylactic strategies
Although most anti-biofilm AMP studies have focused on the prevention of biofilm formation, little is known about some prophylaxis-oriented evaluations. For example, the immobilization or ‘grafting’ of AMPs into surfaces. This immobilization of AMPs, alone or combined, onto a biomaterial surface may circumvent some of the limitations of the peptides, such as a short half-life and cytotoxicity associated with higher concentrations of soluble peptides (Costa et al. Citation2011). The rationale, at this point, is to obtain functionalized surfaces with proven anti-adhesive and anti-biofilm traits to later be tested as potential harmless biomaterials. Several promising studies have already been made, namely with polymer brushes, which have the advantage of being robust and can be generated in most biomedical materials (Gao et al. Citation2011a, Citation2011b). Glinel et al. (Citation2012) report on the existing findings in this field, but further studies are required in order to escalate findings onto a broader range of biomedical surfaces and devices.
Therapeutic strategies
Therapeutic studies are a pressing need as means of assessing the ability of AMPs to kill already existing biofilms. Indeed, it is common knowledge that mature biofilms are more difficult to eradicate, mainly due to the exopolymeric matrix surrounding the cells. Moreover, that mature biofilms are resistant to treatment may be due to their slow growth rate and low metabolic activity and thus, most antibiotics will not be useful because their primary target is metabolically active cells (Lewis Citation2001; Walters et al. Citation2003). AMPs have the ability to act on slow-growing or even non-growing bacteria because they act mainly by permeabilizing and/or forming pores within the cytoplasmic membrane. However, there is still little understanding about mechanisms of action of anti-biofilm AMPs.
Naturally, studies on the toxicity and the post-AMP effect (PAE) are the next steps. Toxicity studies on animal cell lines are fundamental for evaluating AMPs as possible therapeutic drugs to be administered to human patients. Likewise, the analysis of biofilm behavior in response to AMP administration cycles and the post-treatment existence of persister cells are very important to ensure the effectiveness and improvement of AMP strategies.
Synergism studies
Synergistic compounds are a relatively unexplored source of new pharmaceutical products. The combination of innovative and conventional antimicrobial compounds is being put into practice to improve both the prophylactic and therapeutic efficacy of some drugs and lower drug dosage, thus reducing toxic side effects (Ncube et al. Citation2008; Wei et al. Citation2011).
Natural synergic mechanisms are known in Nature, namely those involved in AMP action. This explains why AMPs require much lower physiological concentrations than those in vitro to be effective (Lai and Gallo Citation2009). There are already some examples of the positive outcomes of this type of approach in biofilms, although the number of studies is still far from what would be expected given the prospects of this line of research ().
Table 4. Synergism studies involving AMPs.
Most synergism studies involving AMPs are centered on the planktonic state. Also, AMPs are tested in combination with other chemicals but rarely with other AMPs. Studies focus either on Gram-positive and Gram-negative bacteria, more precisely into the biofilm-forming strains of P. aeruginosa, S. aureus and S. mutans. Due to their clinical profile, these strains are of obvious interest but studies should be extended to other biofilm-forming species to evaluate and take full advantage of the potential of AMPs.
Standardization of laboratory procedures
Current protocols for laboratory research lack standardization, and therefore validity, in some ways. Specifically, the lack of standard protocols hampers the quality of the information generated by researchers and makes the reproduction of results in different laboratories difficult. Studies often target the same scenario but the results are not comparable because they are performed using different methods of analysis, which result in diverse biological data and lead to varying conclusions (Jackson et al. Citation2001).
There are three basic statistical requirements which should be attained in order to establish a standard operating procedure (SOP): ruggedness, repeatability and reproducibility. Ruggedness is the resistance to change in the results produced by an analytical method when minor deviations are made from the experimental conditions described in the procedure. Repeatability accounts for random errors in the measurements and includes the contributions from any part of the procedure that varies within a run, including gravimetric and volumetric errors, heterogeneity of the materials being tested, and variation in the chemical treatment stages. Reproducibility relates to the ability of the procedure to be reproduced by others, namely in different laboratories. Between-laboratory variation arises from factors such as variations in calibration standards, differences between local interpretations of the protocol, changes in equipment or reagent source, and environmental factors, such as differences in the average climatic conditions (Thompson et al. Citation2002).
Currently, no SOP is available for AMP studies, but there are some biofilm and antibiotic SOPs. The SOPs proposed for biofilm studies are specific for: the growth of reproducible mixed biofilms of P. aeruginosa (ATCC#700829), Pseudomonas fluorescens (ATCC# 700830) and Klebsiella pneumoniae (ATCC#700831), analyzed in terms of structure and viable cell counts using a flat-plate, open channel reactor (Jackson et al. Citation2001); the growth of P. aeruginosa biofilm using the CDC biofilm reactor (CBR) system (Goeres et al. Citation2005); the assessment of resistance of Staphylococcus aureus biofilm cells to disinfectants (Luppens et al. Citation2002); and the optimized quantification of enterococci biofilms using microtitre-plates (Extremina et al. Citation2011). Also, as a first step towards the establishment of a SOP for methods of analysis, several authors have studied and compared methods in use on biofilm related research for evaluating biofilm disinfectant efficacy with different fluid dynamics (CDC, drip flow and static biofilm reactor systems) (Buckingham-Meyer et al. Citation2007), comparing methods for quantification of microbial biofilms grown in microtiter plates (crystal violet (CV), Syto9, fluorescein diacetate (FDA), resazurin, XTT and dimethyl methylene blue (DMMB) assays) (Peeters et al. Citation2008), and checking the validity of the harvesting and disaggregating steps (Hamilton et al. Citation2009).
The SOPs proposed for antibiotic studies address, among other things: the assessment of antibiotic resistance using a disc susceptibility test for various genera of microorganisms (Andrews Citation2001; Kronvall et al. Citation2003; Wikler et al. Citation2009a) and specifically, for aquaculture-organisms at international level (Huys et al. Citation2005) and infrequently isolated or fastidious bacteria (Jorgensen et al. Citation2005); broth dilution and disk diffusion antifungal susceptibility testing of yeasts (Rex et al. Citation2008, Citation2009); and dilution antimicrobial susceptibility tests for aerobic bacteria (Wikler et al. Citation2009b).
Data standardization and interchange
Understanding AMP related phenomena requires the integration of heterogeneous data sources. This implies the development and use of annotation standards such as controlled vocabularies and ontologies, ie a formal description of the concepts and relationships involved in AMP studies that facilitate both communication between researchers and the computational use of domain knowledge for multiple purposes (Consortium Citation2004).
These integration efforts have already succeeded in some research areas and various ontologies are being developed and available for use. One major example is the Gene Ontology (GO) project, which provides consistent descriptors for gene products, in different databases, and standardizes classifications for sequences and sequence features (Consortium Citation2004). Other examples follow, such as the Chemical Entities of Biological Interest (ChEBI), which is a freely available dictionary of molecular entities focused on ‘small’ chemical compounds (Degtyarenko et al. Citation2008), or the Plant Ontology Database, which aims to create, maintain and facilitate the use of a controlled vocabulary for plants, providing a semantic framework to make meaningful cross-species and database comparisons (Avraham et al. Citation2008). Some work has also been focused on antibiotics, namely the DebugIT Core Ontology, which focuses on patients, diseases, pathogens, their analyses and medications, allowing a semantic integration of antibiotics resistance patterns (Schober et al. Citation2010). As for AMPs, no nomenclature is yet available, apart from generic protein ontology, such as the Protein Ontology (PRO) that provides a formal, logically-based classification of specific protein classes (Natale et al. Citation2011).
On a need basis, the curation teams of AMP databases are taking the lead in this subject: PenBase suggests a nomenclature for penaeidins, based on the genus and species of the source, its subgroup and an identification number ( http://www.penbase.immun aqua.com/); APD2 proposes a nomenclature for AMPs based on properties, source and a combination of property and source data, and provides a glossary for terms and abbreviations for the entire database ( http://aps.unmc.edu/AP/main.php).
Data screening and processing
As the volume of related literature increases, it becomes urgent to help researchers and database curators to keep up with new literature, especially to provide the means for the systematic screening and prioritization of literature of interest. Bioinformaticians/chemoinformaticians can benefit from this support as well, in particular to delineate new hypotheses to be modeled and mined.
Given the success reported in other biomedical domains, the integration of semi-automated mechanisms into AMP curation workflows would seem to be an ideal application for text mining. State-of-the-art recognizers can be used to tag organisms (Gerner et al. Citation2010), chemical entities (Jessop et al. Citation2011) and genes, proteins and other biological entities (Settles Citation2005). The vocabulary on AMPs, eg derived from the UniProt knowledge base (Magrane and Consortium Citation2011) and antibiotics lexicon derived from the ARDB database (Liu and Pop Citation2009) and the antibiotics list in Wikipedia (Wikipedia Citation2012), can support the pattern matching of AMP mentions and further development of suitable recognizers. Also, to start with, article screening and ranking could be based on statistical considerations, such as the number and diversity of unique concepts detected in the texts, weighted by the relevance associated with the different biological categories, and the degree of certainty associated with detection.
To date, the curation of AMP databases is exclusively manual and little has been reported on text mining developments (Jorge et al. Citation2012).
Conclusions
In the nosocomial scenario, infections are often caused by or associated with microbial adhesion and biofilm development. Compared to planktonic (non-attached) cells, bacteria growing in biofilms have a specific phenotype and demonstrate higher resistance to antimicrobial agents. Therefore, biofilms are difficult to kill and eradicate, contributing to surface persistent contamination and the prevelance of nosocomial pathogens.
The challenges posed to conventional strategies have led to the investigation of alternative prophylaxis and therapeutics, namely AMP-based strategies. These strategies take advantage of the non-specific mechanisms of action of AMPs and the wide availability of AMP-deriving sources. AMPs act mainly by destabilizing cellular membranes, which is a mechanism low in specificity and therefore impairs the development of resistance. Also, AMPs are spread throughout Nature, and can be synthesized or modified to improve their action and reduce production costs.
To date, most AMP studies have focused on planktonic cells. Nevertheless, since biofilms are involved in 80% of human bacterial infections (Harro et al. Citation2010), it is crucial to extend the studies on biofilm inhibition and AMP dispersion effects to already pre-formed biofilms and to developing biofilms. Also, synergism studies involving AMPs are needed to discover successful AMP-AMP or AMP-drug combinations for lowering the dosage and minimizing the side effects of antimicrobials.
Finally, it is noteworthy that the discovery and design of AMPs is being revolutionized by the emergence of novel bioinformatic tools and resources, mainly ‘omics’ experiments and predictive applications.
Acknowledgements
Financial support from IBB-CEB and Fundação para a Ciência e Tecnologia (FCT) and European Community fund FEDER, through Program COMPETE, in the ambit of the FCT project ‘PTDC/SAU-SAP/113196/2009/ FCOMP-01-0124-FEDER-016012’ is gratefully acknowledged.
References
- Allesen-Holm , M , Barken , KB , Yang , L , Klausen , M , Webb , JS , Kjelleberg , S , Molin , S , Givskov , M and Tolker-Nielsen , T . 2006 . A characterization of DNA release in Pseudomonas aeruginosa cultures and biofilms . Mol Microbiol , 59 : 1114 – 1128 .
- Altman , H , Steinberg , D , Porat , Y , Mor , A , Fridman , D , Friedman , M and Bachrach , G . 2006 . In vitro assessment of antimicrobial peptides as potential agents against several oral bacteria . J Antimicrob Chemoth , 58 : 198 – 201 .
- Altschul , SF , Gish , W , Miller , W , Myers , EW and Lipman , DJ . 1990 . Basic local alignment search tool . J Mol Biol , 215 : 403 – 410 .
- Alugupalli , KR and Kalfas , S . 1995 . Inhibitory effect of lactoferrin on the adhesion of Actinobacillus actinomycetemcotnitans and Prevotella intermedia to fibroblasts and epithelial cells . APMIS , 103 : 154 – 160 .
- Alugupalli , KR , Kalfas , S , Edwardsson , S , Forsgren , A , Arnold , RR and Naidu , AS . 1994 . Effect of lactoferrin on interaction of Prevotella intermedia with plasma and subepithelial matrix proteins . Oral Microbiol Immun , 9 : 174 – 179 .
- Andrews , JM . 2001 . BSAC standardized disc susceptibility testing method . J Antimicrob Chemoth , 48 : 43 – 57 .
- Aoki , N , Tateda , K , Kikuchi , Y , Kimura , S , Miyazaki , C , Ishii , Y , Tanabe , Y , Gejyo , F and Yamaguchi , K . 2009 . Efficacy of colistin combination therapy in a mouse model of pneumonia caused by multidrug-resistant Pseudomonas aeruginosa . J Antimicrob Chemoth , 63 : 534 – 542 .
- Avraham , S , Tung , C-W , Ilic , K , Jaiswal , P , Kellogg , EA , McCouch , S , Pujar , A , Reiser , L , Rhee , SY Sachs , MM . 2008 . The Plant Ontology Database: a community resource for plant structure and developmental stages controlled vocabulary and annotations . Nucleic Acids Res , 36 : D449 – D454 .
- Bagheri , M , Beyermann , M and Dathe , M . 2012 . Mode of action of cationic antimicrobial peptides defines the tethering position and the efficacy of biocidal surfaces . Bioconjugate Chem , 23 : 66 – 74 .
- Bals , R and Wilson , JM . 2003 . Cathelicidins – a family of multifunctional antimicrobial peptides . Cell Mol Life Sci , 60 : 711 – 720 .
- Baltzer , SA and Brown , MH . 2011 . Antimicrobial peptides – promising alternatives to conventional antibiotics . J Mol Microbiol Biot , 20 : 228 – 235 .
- Batoni , G , Maisetta , G , Brancatisano , FL , Esin , S and Campa , M . 2011 . Use of antimicrobial peptides against microbial biofilms: advantages and limits . Curr Med Chem , 18 : 256 – 279 .
- Bechinger , B and Lohner , K . 2006 . Detergent-like actions of linear amphipathic cationic antimicrobial peptides . Biochim Biophys Acta , 1758 : 1529 – 1539 .
- Beckloff , N , Laube , D , Castro , T , Furgang , D , Park , S , Perlin , D , Clements , D , Tang , H , Scott , RW Tew , GN . 2007 . Activity of an antimicrobial peptide mimetic against planktonic and biofilm cultures of oral pathogens . Antimicrob Agents Ch , 51 : 4125 – 4132 .
- Bhonsle , JB , Venugopal , D , Huddler , DP , Magill , AJ and Hicks , RP . 2007 . Application of 3D-QSAR for identification of descriptors defining bioactivity of antimicrobial peptides . J Med Chem , 50 : 6545 – 6553 .
- Blondelle , SE and Lohner , K . 2010 . Optimization and high-throughput screening of antimicrobial peptides . Curr Pharm Design , 16 : 3204 – 3211 .
- Breiman , L . 2001 . Random forests . Mach Learn , 45 : 5 – 32 .
- Bruschi , M , Pirri , G , Giuliani , A , Nicoletto , SF , Baster , I , Scorciapino , MA , Casu , M and Rinaldi , AC . 2010 . Synthesis, characterization, antimicrobial activity and LPS-interaction properties of SB041, a novel dendrimeric peptide with antimicrobial properties . Peptides , 31 : 1459 – 1467 .
- Brusic , V , Rudy , G , Honeyman , G , Hammer , J and Harrison , L . 1998 . Prediction of MHC class II-binding peptides using an evolutionary algorithm and artificial neural network . Bioinformatics , 14 : 121 – 130 .
- Buckingham-Meyer , K , Goeres , DM and Hamilton , MA . 2007 . Comparative evaluation of biofilm disinfectant efficacy tests . J Microbiol Meth , 70 : 236 – 244 .
- Cederlund , A , Gudmundsson , GH and Agerberth , B . 2011 . Antimicrobial peptides important in innate immunity . FEBS J , 278 : 3942 – 3951 .
- Chau , T . 2010 . Delivery, design, and mechanism of antimicrobial peptides , 183 Berkeley , CA : University of California . [PhD thesis]
- Cherkasov , A , Hilpert , K , Jenssen , H , Fjell , CD , Waldbrook , M , Mullaly , SC , Volkmer , R and Hancock , REW . 2008 . Use of artificial intelligence in the design of small peptide antibiotics effective against a broad spectrum of highly antibiotic-resistant superbugs . ACS Chem Biol , 4 : 65 – 74 .
- Chongsiriwatana , NP , Patch , JA , Czyzewski , AM , Dohm , MT , Ivankin , A , Gidalevitz , D , Zuckermann , RN and Barron , AE . 2008 . Peptoids that mimic the structure, function, and mechanism of helical antimicrobial peptides . P Natl Acad Sci USA , 105 : 2794 – 2799 .
- Cirioni , O , Giacometti , A , Ghiselli , R , Bergnach , C , Orlando , F , Mocchegiani , F , Silvestri , C , Licci , A , Skerlavaj , B Zanetti , M . 2006 . Pre-treatment of central venous catheters with the cathelicidin BMAP-28 enhances the efficacy of antistaphylococcal agents in the treatment of experimental catheter-related infection . Peptides , 27 : 2104 – 2110 .
- Cirioni , O , Ghiselli , R , Silvestri , C , Kamysz , W , Orlando , F , Mocchegiani , F , Di , Matteo F , Riva , A , Łukasiak , J Scalise , G . 2007 . Efficacy of tachyplesin III, colistin, and imipenem against a multiresistant Pseudomonas aeruginosa strain . Antimicrob Agents Ch , 51 : 2005 – 2010 .
- Cirioni , O , Ghiselli , R , Kamysz , W , Orlando , F , Silvestri , C , Mocchegiani , F , Di Matteo , F , Kamysz , E , Riva , A Rocchi , M . 2008a . Tachyplesin III and granulocyte-colony stimulating factor enhance the efficacy of tazobactam/piperacillin in a neutropenic mouse model of polymicrobial peritonitis . Peptides , 29 : 31 – 38 .
- Cirioni , O , Silvestri , C , Ghiselli , R , Orlando , F , Riva , A , Mocchegiani , F , Chiodi , L , Castelletti , S , Gabrielli , E Saba , V . 2008b . Protective effects of the combination of alpha-helical antimicrobial peptides and rifampicin in three rat models of Pseudomonas aeruginosa infection . J Antimicrob Chemoth , 62 : 1332 – 1338 .
- Consortium , GO . 2004 . The Gene Ontology (GO) database and informatics resource . Nucleic Acids Res , 32 : D258 – D261 .
- Corbin , A , Pitts , B , Parker , A and Stewart , PS . 2011 . Antimicrobial penetration and efficacy in an in vitro oral biofilm model . Antimicrob Agents Ch , 55 : 3338 – 3344 .
- Costa , F , Carvalho , IF , Montelaro , RC , Gomes , P and Martins , MCL . 2011 . Covalent immobilization of antimicrobial peptides (AMPs) onto biomaterial surfaces . Acta Biomater , 7 : 1431 – 1440 .
- Costerton , JW , Stewart , PS and Greenberg , EP . 1999 . Bacterial biofilms: a common cause of persistent infections . Science , 284 : 1318
- Das , T , Sharma , PK , Busscher , HJ , van der Mei , HC and Krom , BP . 2010 . Role of extracellular DNA in initial bacterial adhesion and surface aggregation . Appl Environ Microb , 76 : 3405 – 3408 .
- de Jong , A , van Heel , AJ , Kok , J and Kuipers , OP . 2010 . BAGEL2: mining for bacteriocins in genomic data . Nucleic Acids Res , 38 : W647 – W651 .
- de la Fuente-Núñez , C , Korolik , V , Bains , M , Nguyen , U , Breidenstein , EBM , Horsman , S , Lewenza , S , Burrows , L and Hancock , REW . 2012 . Inhibition of bacterial biofilm formation and swarming motility by a small synthetic cationic peptide . Antimicrob Agents Ch , 56 : 2696 – 2704 .
- Dean , S , Bishop , B and van , Hoek M . 2011 . Natural and synthetic cathelicidin peptides with anti-microbial and anti-biofilm activity against Staphylococcus aureus . BMC Microbiol , 11 : 114
- Degtyarenko , K , de Matos , P , Ennis , M , Hastings , J , Zbinden , M , McNaught , A , Alcántara , R , Darsow , M , Guedj , M and Ashburner , M . 2008 . ChEBI: a database and ontology for chemical entities of biological interest . Nucleic Acids Res , 36 : D344 – D350 .
- Dennison , SR , Morton , LHG , Shorrocks , AJ , Harris , F and Phoenix , DA . 2009 . A study on the interactions of aurein 2.5 with bacterial membranes . Colloid Surface B , 68 : 225 – 230 .
- Desbois , A and Coote , P . 2011 . Bactericidal synergy of lysostaphin in combination with antimicrobial peptides . Eur J Clin Microbiol , 30 : 1 – 7 .
- Desbois , AP , Gemmell , CG and Coote , PJ . 2010 . In vivo efficacy of the antimicrobial peptide ranalexin in combination with the endopeptidase lysostaphin against wound and systemic meticillin-resistant Staphylococcus aureus (MRSA) infections . Int J Antimicrob Agents , 35 : 559 – 565 .
- Dobson , A , O'Connor , PM , Cotter , PD , Ross , RP and Hill , C . 2011 . Impact of the broad-spectrum antimicrobial peptide, lacticin 3147, on Streptococcus mutans growing in a biofilm and in human saliva . J Appl Microbiol , 111 : 1515 – 1523 .
- Doherty , T , Waring , AJ and Hong , M . 2006 . Peptide–lipid interactions of the β-hairpin antimicrobial peptide tachyplesin and its linear derivatives from solid-state NMR. Biochim . Biophys Acta , 1758 : 1285 – 1291 .
- Donlan , RM and Costerton , JW . 2002 . Biofilms: survival mechanisms of clinically relevant microorganisms . Clin Microbiol Rev , 15 : 167 – 193 .
- Duclohier , H . 2010 . Antimicrobial peptides and peptaibols, substitutes for conventional antibiotics . Curr Pharm Design , 16 : 3212 – 3223 .
- Eckert , R , He , J , Yarbrough , DK , Qi , F , Anderson , MH and Shi , W . 2006a . Targeted killing of Streptococcus mutans by a pheromone-guided ‘smart’ antimicrobial peptide . Antimicrob Agents Ch , 50 : 3651 – 3657 .
- Eckert , R , Brady , KM , Greenberg , EP , Qi , F , Yarbrough , DK , He , J , McHardy , I , Anderson , MH and Shi , W . 2006b . Enhancement of antimicrobial activity against Pseudomonas aeruginosa by coadministration of G10KHc and tobramycin . Antimicrob Agents Ch , 50 : 3833 – 3838 .
- Eddy , SR . 1998 . Profile hidden Markov models . Bioinformatics , 14 : 755 – 763 .
- Epand , RM and Epand , RF . 2009 . Lipid domains in bacterial membranes and the action of antimicrobial agents . Biochim Biophys Acta , 1788 : 289 – 294 .
- Extremina , CI , Costa , L , Aguiar , AI , Peixe , L and Fonseca , AP . 2011 . Optimization of processing conditions for the quantification of enterococci biofilms using microtitre-plates . J Microbiol Meth , 84 : 167 – 173 .
- Fernandes , FC , Porto , WF and Franco , OL . A wide antimicrobial peptides search method using fuzzy modeling . Proceedings of the 4th Brazilian Symposium on Bioinformatics; .
- Fey , PD . 2010 . Modality of bacterial growth presents unique targets: how do we treat biofilm-mediated infections? . Curr Opin Microbiol , 13 : 610 – 615 .
- Finn , RD , Clements , J and Eddy , SR . 2011 . HMMER web server: interactive sequence similarity searching . Nucleic Acids Res , 39
- Fjell , CD , Hancock , REW and Cherkasov , A . 2007 . AMPer: a database and an automated discovery tool for antimicrobial peptides . Bioinformatics , 23 : 1148 – 1155 .
- Fjell , CD , Hiss , JA , Hancock , REW and Schneider , G . 2012 . Designing antimicrobial peptides: form follows function . Nat Rev Drug Discov , 11 : 37 – 51 .
- Fjell , CD , Jenssen , H , Hilpert , K , Cheung , WA , Panté , N , Hancock , REW and Cherkasov , A . 2009 . Identification of novel antibacterial peptides by chemoinformatics and machine learning . J Med Chem , 52 : 2006 – 2015 .
- Flemming , K , Klingenberg , C , Cavanagh , JP , Sletteng , M , Stensen , W , Svendsen , JS and Flægstad , T . 2009 . High in vitro antimicrobial activity of synthetic antimicrobial peptidomimetics against staphylococcal biofilms . J Antimicrob Chemoth , 63 : 136 – 145 .
- Folkesson , A , Haagensen , JAJ , Zampaloni , C , Sternberg , C and Molin , S . 2008 . Biofilm induced tolerance towards antimicrobial peptides . PLoS One , 3 : e1891
- Frecer , V , Ho , B and Ding , J . 2004 . De novo design of potent antimicrobial peptides . Antimicrob Agents Ch , 48 : 3349 – 3357 .
- Freiberg , C , Fischer , HP and Brunner , NA . 2005 . Discovering the mechanism of action of novel antibacterial agents through transcriptional profiling of conditional mutants . Antimicrob Agents Ch , 49 : 749 – 759 .
- Gao , G , Yu , K , Kindrachuk , J , Brooks , DE , Hancock , REW and Kizhakkedathu , JN . 2011b . Antibacterial surfaces based on polymer brushes: investigation on the influence of brush properties on antimicrobial peptide immobilization and antimicrobial activity . Biomacromolecules , 12 : 3715 – 3727 .
- Gao , G , Cheng , JTJ , Kindrachuk , J , Hancock , REW , Straus , SK and Kizhakkedathu , JN . 2012 . Biomembrane interactions reveal the mechanism of action of surface-immobilized host defense IDR-1010 peptide . Chem Biol , 19 : 199 – 209 .
- Gao , G , Lange , D , Hilpert , K , Kindrachuk , J , Zou , Y , Cheng , JTJ , Kazemzadeh-Narbat , M , Yu , K , Wang , R Straus , SK . 2011a . The biocompatibility and biofilm resistance of implant coatings based on hydrophilic polymer brushes conjugated with antimicrobial peptides . Biomaterials , 32 : 3899 – 3909 .
- Gálvez , A , Abriouel , H , López , RL and Omar , NB . 2007 . Bacteriocin-based strategies for food biopreservation . Int J Food Microbiol , 120 : 51 – 70 .
- Garcia , AE and Camarero , JA . 2010 . Biological activities of natural and engineered cyclotides, a novel molecular scaffold for peptide-based therapeutics . Curr Mol Pharm , 3 : 153 – 163 .
- Gazit , E , Miller , IR , Biggin , PC , Sansom , MSP and Shai , Y . 1996 . Structure and orientation of the mammalian antibacterial peptide cecropin P1 within phospholipid membranes . J Mol Biol , 258 : 860 – 870 .
- Gerner , M , Nenadic , G and Bergman , C . 2010 . LINNAEUS: A species name identification system for biomedical literature . BMC Bioinformatics , 11 : 85
- Giovannini , MG , Poulter , L , Gibson , BW and Williams , DH . 1987 . Biosynthesis and degradation of peptides derived from Xenopus laevis prohormones . Biochem J , 243 : 113 – 120 .
- Giuliani , A and Rinaldi , A . 2011 . Beyond natural antimicrobial peptides: multimeric peptides and other peptidomimetic approaches . Cell Mol Life Sci , 68 : 2255 – 2266 .
- Glinel , K , Thebault , P , Humblot , V , Pradier , CM and Jouenne , T . 2012 . Antibacterial surfaces developed from bio-inspired approaches . Acta Biomater , 8 : 1670 – 1684 .
- Glinel , K , Jonas , AM , Jouenne , T , Leprince , Jrm , Galas , L and Huck , WTS . 2009 . Bioconjugate Chem . Antibacterial and antifouling polymer brushes incorporating antimicrobial peptide , 20 : 71 – 77 .
- Goeres , DM , Loetterle , LR , Hamilton , MA , Murga , R , Kirby , DW and Donlan , RM . 2005 . Statistical assessment of a laboratory method for growing biofilms . Microbiology , 151 : 757 – 762 .
- Graham , S and Coote , PJ . 2007 . Potent, synergistic inhibition of Staphylococcus aureus upon exposure to a combination of the endopeptidase lysostaphin and the cationic peptide ranalexin . J Antimicrob Chemoth , 59 : 759 – 762 .
- Gueguen , Y , Garnier , J , Robert , L , Lefranc , M-P , Mougenot , I , de Lorgeril , J , Janech , M , Gross , PS , Warr , GW Cuthbertson , B . 2006 . PenBase, the shrimp antimicrobial peptide penaeidin database: sequence-based classification and recommended nomenclature . Dev Comp Immunol , 30 : 283 – 288 .
- Haagensen , JAJ , Klausen , M , Ernst , RK , Miller , SI , Folkesson , A , Tolker-Nielsen , T and Molin , S . 2007 . Differentiation and distribution of colistin- and sodium dodecyl sulfate-tolerant cells in Pseudomonas aeruginosa biofilms . J Bacteriol , 189 : 28 – 37 .
- Hale , JDF and Hancock , REW . 2007 . Alternative mechanisms of action of cationic antimicrobial peptides on bacteria . Expert Rev Anti-Infect Ther , 5 : 951 – 959 .
- Hall-Stoodley , L and Stoodley , P . 2009 . Evolving concepts in biofilm infections . Cell Microbiol , 11 : 1034 – 1043 .
- Hamilton , M , Buckingham-Meyer , K and Goeres , DM . 2009 . Checking the validity of the harvesting and disaggregating steps in laboratory tests of surface disinfectants . J AOAC Int , 92 : 1755 – 1762 .
- Hammami , R and Fliss , I . 2010 . Current trends in antimicrobial agent research: chemo- and bioinformatics approaches . Drug Discov Today , 15 : 540 – 546 .
- Hammami , R , Zouhir , A , Ben , Hamida J and Fliss , I . 2007 . BACTIBASE: a new web-accessible database for bacteriocin characterization . BMC Microbiol , 7 : 89
- Hammami , R , Ben Hamida , J , Vergoten , G and Fliss , I . 2009 . PhytAMP: a database dedicated to antimicrobial plant peptides . Nucleic Acids Res , 37 : D963 – D968 .
- Hammami , R , Zouhir , A , Le Lay , C , Ben Hamida , J and Fliss , I . 2010 . BACTIBASE second release: a database and tool platform for bacteriocin characterization . BMC Microbiol , 10 : 22
- Hancock , REW and Lehrer , R . 1998 . Cationic peptides: a new source of antibiotics . Trends Biotechnol , 16 : 82 – 88 .
- Harro , JM , Peters , BM , O'May , GA , Archer , N , Kerns , P , Prabhakara , R and Shirtliff , ME . 2010 . Vaccine development in Staphylococcus aureus: taking the biofilm phenotype into consideration . FEMS Immunol Med Mic , 59 : 306 – 323 .
- He , J , Yarbrough , DK , Kreth , J , Anderson , MH , Shi , W and Eckert , R . 2010 . Systematic approach to optimizing specifically targeted antimicrobial peptides against Streptococcus mutans . Antimicrob Agents Ch , 54 : 2143 – 2151 .
- Héquet , A , Humblot , V , Berjeaud , J-M and Pradier , C-M . 2011 . Optimized grafting of antimicrobial peptides on stainless steel surface and biofilm resistance tests . Colloid Surface B , 84 : 301 – 309 .
- Herbert , S , Bera , A , Nerz , C , Kraus , D , Peschel , A , Goerke , C , Meehl , M , Cheung , A and Götz , F . 2007 . Molecular basis of resistance to muramidase and cationic antimicrobial peptide activity of lysozyme in staphylococci . PLoS Pathog , 3 : e102
- Herrmann , G , Yang , L , Wu , H , Song , Z , Wang , H , Høiby , N , Ulrich , M , Molin , S , Riethmüller , J and Döring , G . 2010 . Colistin-tobramycin combinations are superior to monotherapy concerning the killing of biofilm Pseudomonas aeruginosa . J Infect Dis , 202 : 1585 – 1592 .
- Hilpert , K , Fjell , CD and Cherkasov , A . 2008 . Short linear cationic antimicrobial peptides: screening, optimizing, and prediction . Methods Mol Biol , 494 : 127 – 159 .
- Hilpert , K , Elliott , MR , Volkmer-Engert , R , Henklein , P , Donini , O , Zhou , Q , Winkler , DFH and Hancock , REW . 2006 . Sequence requirements and an optimization strategy for short antimicrobial peptides . Chem Biol , 13 : 1101 – 1107 .
- Høiby , N , Bjarnsholt , T , Givskov , M , Molin , S and Ciofu , O . 2010 . Antibiotic resistance of bacterial biofilms . Int J Antimicrob Ag , 35 : 322 – 332 .
- Hopfield , JJ . 1988 . Artificial neural networks . IEEE Circuit Devic , 4 : 3 – 10 .
- Hou , S , Zhou , C , Liu , Z , Young , AW , Shi , Z , Ren , D and Kallenbach , NR . 2009 . Antimicrobial dendrimer active against Escherichia coli biofilms . Bioorg Med Chem Lett , 19 : 5478 – 5481 .
- Hutter , B , Schaab , C , Albrecht , S , Borgmann , M , Brunner , NA , Freiberg , C , Ziegelbauer , K , Rock , CO , Ivanov , I and Loferer , H . 2004 . Prediction of mechanisms of action of antibacterial compounds by gene expression profiling . Antimicrob Agents Ch , 48 : 2838 – 2844 .
- Huys , G , Cnockaert , M , Bartie , K , Oanh , DTH , Phuong , N , Somsiri , T , Chinabut , S , Yussoff , FM , Shariff , M Giacomini , M . 2005 . Intra- and interlaboratory performance of antibiotic disk-diffusion-susceptibility testing of bacterial control strains of relevance for monitoring aquaculture environments . Dis Aquat Organ , 66 : 197 – 204 .
- Iwasaki , T , Saido-Sakanaka , H , Asaoka , A , Taylor , D , Ishibashi , J and Yamakawa , M . 2007 . In vitro activity of diastereomeric antimicrobial peptides alone and in combination with antibiotics against methicillin-resistant Staphylococcus aureus and Pseudomonas aeruginosa . J Insect Biot Seric , 76 : 25 – 29 .
- Jackson , G , Beyenal , H , Rees , WM and Lewandowski , Z . 2001 . Growing reproducible biofilms with respect to structure and viable cell counts . J Microbiol Meth , 47 : 1 – 10 .
- Jenssen , H , Fjell , CD , Cherkasov , A and Hancock , REW . 2008 . QSAR modeling and computer-aided design of antimicrobial peptides . J Pept Sci , 14 : 110 – 114 .
- Jessop , D , Adams , S , Willighagen , E , Hawizy , L and Murray-Rust , P . 2011 . OSCAR4: a flexible architecture for chemical text-mining . J Cheminform , 3 : 41
- Jorge , P , Pereira , MO and Lourenço , A . Facilitating the screening of AMP literature via text mining strategies . Proceedings of the AMP2012. Lille (France): AMP2012. p. E-134 .
- Jorgensen , JH , Patel , JB , Hindler , J , Schreckenberger , PC , Citron , DM , Turnidge , JD , Cockerill , FR , Walker , RD , Fritsche , TR Welch , DF . 2005 . Methods for antimicrobial dilution and disk susceptibility testing of infrequently isolated or fastidious bacteria; proposed guideline . Wayne (PA): Clinical and Laboratory Standards Institute (CLSI). p. 1–61 ,
- Juretić , D , Vukičević , D , Ilić , N , Antcheva , N and Tossi , A . 2009 . Computational design of highly selective antimicrobial peptides . J Chem Inf Model , 49 : 2873 – 2882 .
- Juretić , D , Vukičević , D , Petrov , D , Novković , M , Bojović , V , Lučić , B , Ilić , N and Tossi , A . 2011 . Knowledge-based computational methods for identifying or designing novel, non-homologous antimicrobial peptides . Eur Biophys J , 40 : 371 – 385 .
- Kader , J-C . 1996 . Lipid-tranfer proteins in plants . Annu Rev Plant Phys , 47 : 627 – 654 .
- Kanthawong , S , Bolscher , JGM , Veerman , ECI , van Marle , J , de Soet , HJJ , Nazmi , K , Wongratanacheewin , S and Taweechaisupapong , S . 2012 . Antimicrobial and antibiofilm activity of LL-37 and its truncated variants against Burkholderia pseudomallei . Int J Antimicrob Ag , 39 : 39 – 44 .
- Kapoor , R , Wadman , MW , Dohm , MT , Czyzewski , AM , Spormann , AM and Barron , AE . 2011 . Antimicrobial peptoids are effective against Pseudomonas aeruginosa biofilms . Antimicrob Agents Ch , 55 : 3054 – 3057 .
- Kazemzadeh-Narbat , M , Kindrachuk , J , Duan , K , Jenssen , H , Hancock , REW and Wang , R. 2010 . Antimicrobial peptides on calcium phosphate-coated titanium for the prevention of implant-associated infections . Biomaterials , 31 : 9519 – 9526 .
- Kharidia , R and Liang , J . 2011 . The activity of a small lytic peptide PTP-7 on Staphylococcus aureus biofilms . J Microbiol , 49 : 663 – 668 .
- Kido , EA , Pandolfi , V , Houllou-Kido , LM , Andrade , PP , Marcelino , FC , Nepomuceno , AL , Abdelnoor , RV , Benko-Iseppon , AM and Burnquist , WL . 2010 . Plant antimicrobial peptides: an overview of SuperSAGE transcriptional profile and a functional review . Curr Protein Peptide Sci , 11 : 220 – 230 .
- Kim , SS , Kim , S , Kim , E , Hyun , B , Kim , K-K and Lee , BJ . 2003 . Synergistic inhibitory effect of cationic peptides and antimicrobial agents on the growth of oral streptococci . Anglais , 37 : 6
- Kim , Y , Atalla , H , Mallard , B , Robert , C and Karrow , N . 2011 . Changes in Holstein cow milk and serum proteins during intramammary infection with three different strains of Staphylococcus aureus . BMC Vet Res , 7 : 51
- Kronvall , G , Kahlmeter , G , Myhre , E and Galas , MF . 2003 . A new method for normalized interpretation of antimicrobial resistance from disk test results for comparative purposes . Clin Microbiol Infec , 9 : 120 – 132 .
- Krusong , K , Poolpipat , P , Supungul , P and Tassanakajon , A . 2012 . A comparative study of antimicrobial properties of crustinPm1 and crustinPm7 from the black tiger shrimp Penaeus monodon . Dev Comp Immunol , 36 : 208 – 215 .
- Lachenbruch , PA and Goldstein , M . 1979 . Discriminant analysis . Biometrics , 35 : 69 – 85 .
- Lai , Y and Gallo , RL . 2009 . AMPed up immunity: how antimicrobial peptides have multiple roles in immune defense . Trends Immunol , 30 : 131 – 141 .
- Lata , S , Sharma , B and Raghava , G . 2007 . Analysis and prediction of antibacterial peptides . BMC Bioinformatics , 8 : 263
- Lata , S , Mishra , N and Raghava , G . 2010 . AntiBP2: improved version of antibacterial peptide prediction . BMC Bioinformatics , 11 : S19
- Leite , JRSA , Silva , LP , Rodrigues , MIS , Prates , MV , Brand , GD , Lacava , BM , Azevedo , RB , Bocca , AL , Albuquerque , S and Bloch , C Jr . 2005 . Phylloseptins: a novel class of anti-bacterial and anti-protozoan peptides from the Phyllomedusa genus . Peptides , 26 : 565 – 573 .
- Lewis , K . 2001 . Riddle of biofilm resistance . Antimicrob Agents Ch , 45 : 999 – 1007 .
- Li , Y and Chen , Z . 2008 . RAPD: a database of recombinantly-produced antimicrobial peptides . FEMS Microbiol Lett , 289 : 126 – 129 .
- Lipman , D and Pearson , W . 1985 . Rapid and sensitive protein similarity searches . Science , 227 : 1435 – 1441 .
- Liu , Y , Wang , L , Zhou , X , Hu , S , Zhang , S and Wu , H . 2011 . Effect of the antimicrobial decapeptide KSL on the growth of oral pathogens and Streptococcus mutans biofilm . Int J Antimicrob Ag , 37 : 33 – 38 .
- Liu , B and Pop , M . 2009 . ARDB – Antibiotic resistance genes database . Nucleic Acids Res , 37
- Liu , H and Setiono , R . 1998 . Incremental feature selection . Appl Intell , 9 : 217 – 230 .
- Lobo , BA , Vetro , JA , Suich , DM , Zuckermann , RN and Middaugh , CR . 2003 . Structure/function analysis of peptoid/lipitoid:DNA complexes . J Pharm Sci , 92 : 1905 – 1918 .
- Lobos , O , Padilla , A and Padilla , C . 2009 . In vitro antimicrobial effect of bacteriocin PsVP-10 in combination with chlorhexidine and triclosan against Streptococcus mutans and Streptococcus sobrinus strains . Arch Oral Biol , 54 : 230 – 234 .
- Ludtke , SJ , He , K , Heller , WT , Harroun , TA , Yang , L and Huang , HW . 1996 . Membrane pores induced by magainin . Biochemistry , 35 : 13723 – 13728 .
- Luppens , SBI , Reij , MW , van der Heijden , RWL , Rombouts , FM and Abee , T . 2002 . Development of a standard test to assess the resistance of Staphylococcus aureus biofilm cells to disinfectants . Appl Environ Microb , 68 : 4194 – 4200 .
- Ma , Y , Liu , C , Liu , X , Wu , J , Yang , H , Wang , Y , Li , J , Yu , H and Lai , R . 2010 . Peptidomics and genomics analysis of novel antimicrobial peptides from the frog, Rana nigrovittata . Genomics , 95 : 66 – 71 .
- Madhuri , Shireen , T , Venugopal , SK , Ghosh , D , Gadepalli , R , Dhawan , B and Mukhopadhyay , K . 2009 . In vitro antimicrobial activity of alpha-melanocyte stimulating hormone against major human pathogen Staphylococcus aureus . Peptides , 30 : 1627 – 1635 .
- Magrane , M and Consortium , U . 2011 . UniProt Knowledgebase: a hub of integrated protein data . Database (Oxford) 2011:bar009 ,
- Malmsten , M , Kasetty , G , Pasupuleti , M , Alenfall , J and Schmidtchen , A . 2011 . Highly selective end-tagged antimicrobial peptides derived from PRELP . PLoS One , 6 : e16400
- Mandal , SM , Migliolo , L , Franco , OL and Ghosh , AK . 2011 . Identification of an antifungal peptide from Trapa natans fruits with inhibitory effects on Candida tropicalis biofilm formation . Peptides , 32 : 1741 – 1747 .
- Martti , V . 2010 . Polymyxins and their novel derivatives . Curr Opin Microbiol , 13 : 574 – 581 .
- Mason , AJ , Chotimah , INH , Bertani , P and Bechinger , B . 2006 . A spectroscopic study of the membrane interaction of the antimicrobial peptide pleurocidin . Mol Membr Biol , 23 : 185 – 194 .
- Matyus , E , Kandt , C and Tieleman , DP . 2007 . Computer simulation of antimicrobial peptides . Curr Med Chem , 14 : 2789 – 2798 .
- Melo , MN , Ferre , R and Castanho , MARB . 2009 . Antimicrobial peptides: linking partition, activity and high membrane-bound concentrations . Nat Rev Microbiol , 7 : 245 – 250 .
- Miller , SI , Ernst , RK and Bader , MW . 2005 . LPS, TLR4 and infectious disease diversity . Nat Rev Microbiol , 3 : 36 – 46 .
- Minardi , D , Ghiselli , R , Cirioni , O , Giacometti , A , Kamysz , W , Orlando , F , Silvestri , C , Parri , G , Kamysz , E Scalise , G . 2007 . The antimicrobial peptide tachyplesin III coated alone and in combination with intraperitoneal piperacillin-tazobactam prevents ureteral stent Pseudomonas infection in a rat subcutaneous pouch model . Peptides , 28 : 2293 – 2298 .
- Mogi , T and Kita , K . 2009 . Gramicidin S and polymyxins: the revival of cationic cyclic peptide antibiotics . Cell Mol Life Sci , 66 : 3821 – 3826 .
- Molhoek , EM , van Dijk , A , Veldhuizen , EJA , Haagsman , HP and Bikker , FJ . 2011 . A cathelicidin-2-derived peptide effectively impairs Staphylococcus epidermidis biofilms . Int J Antimicrob Ag , 37 : 476 – 479 .
- Nakamura , T , Furunaka , H , Miyata , T , Tokunaga , F , Muta , T , Iwanaga , S , Niwa , M , Takao , T and Shimonishi , Y . 1988 . Tachyplesin, a class of antimicrobial peptide from the hemocytes of the horseshoe crab (Tachypleus tridentatus). Isolation and chemical structure . J Biol Chem , 263 : 16709 – 16713 .
- Natale , DA , Arighi , CN , Barker , WC , Blake , JA , Bult , CJ , Caudy , M , Drabkin , HJ , D'Eustachio , P , Evsikov , AV Huang , H . 2011 . The protein ontology: a structured representation of protein forms and complexes . Nucleic Acids Res , 39
- Ncube , NS , Afolayan , AJ and Okoh , AI . 2008 . Assessment techniques of antimicrobial properties of natural compounds of plant origin: current methods and future trends . Afr J Biotechnol , 7 : 1797 – 1806 .
- Nguyen , LT , Haney , EF and Vogel , HJ . 2011 . The expanding scope of antimicrobial peptide structures and their modes of action . Trends Biotechnol , 29 : 464 – 472 .
- Nicolas , P and El Amri , C . 2009 . The dermaseptin superfamily: a gene-based combinatorial library of antimicrobial peptides . Biochim Biophys Acta , 1788 : 1537 – 1550 .
- Oppenheim , FG , Xu , T , McMillian , FM , Levitz , SM , Diamond , RD , Offner , GD and Troxler , RF . 1988 . Histatins, a novel family of histidine-rich proteins in human parotid secretion. Isolation, characterization, primary structure, and fungistatic effects on Candida albicans . J Biol Chem , 263 : 7472 – 7477 .
- Osaki , T , Sasaki , K and Minamino , N . 2011 . Peptidomics-based discovery of an antimicrobial peptide derived from insulin-like growth factor-binding protein 5 . J Proteome Res , 10 : 1870 – 1880 .
- Ostolaza , H , Bartolome , B , Ortiz de Zarate , I , de la Cruz , F and Goni , FM . 1993 . Release of lipid vesicle contents by the bacterial protein toxin alpha-haemolysin . Biochim Biophys Acta , 1147 : 81 – 88 .
- Otvos , L . 2005 . Antibacterial peptides and proteins with multiple cellular targets . J Pept Sci , 11 : 697 – 706 .
- Overhage , J , Campisano , A , Bains , M , Torfs , ECW , Rehm , BHA and Hancock , REW . 2008 . Human host defense peptide LL-37 prevents bacterial biofilm formation . Infect Immun , 76 : 4176 – 4182 .
- Overton , I , Graham , S , Gould , K , Hinds , J , Botting , C , Shirran , S , Barton , G and Coote , P . 2011 . Global network analysis of drug tolerance, mode of action and virulence in methicillin-resistant S. aureus . BMC Syst Biol , 5 : 68
- Pamp , SJ , Gjermansen , M , Johansen , HK and Tolker-Nielsen , T . 2008 . Tolerance to the antimicrobial peptide colistin in Pseudomonas aeruginosa biofilms is linked to metabolically active cells, and depends on the pmr and mexAB-oprM genes . Mol Microbiol , 68 : 223 – 240 .
- Park , S-C , Park , Y and Hahm , K-S . 2011 . The role of antimicrobial peptides in preventing multidrug-resistant bacterial infections and biofilm formation . Int J Mol Sci , 12 : 5971 – 5992 .
- Patrzykat , A , Friedrich , CL , Zhang , L , Mendoza , V and Hancock , REW . 2002 . Sublethal concentrations of pleurocidin-derived antimicrobial peptides inhibit macromolecular synthesis in Escherichia coli . Antimicrob Agents Ch , 46 : 605 – 614 .
- Peeters , E , Nelis , HJ and Coenye , T . 2008 . Comparison of multiple methods for quantification of microbial biofilms grown in microtiter plates . J Microbiol Meth , 72 : 157 – 165 .
- Peng , H , Long , F and Ding , C . 2003 . Feature selection based on mutual information: criteria of max-dependency, max-relevance, and min-redundancy . IEEE T Pattern Anal , 27 : 1226 – 1238 .
- Peschel , A and Sahl , H-G . 2006 . The co-evolution of host cationic antimicrobial peptides and microbial resistance . Nat Rev Microbiol , 4 : 529 – 536 .
- Pestana-Calsa , MC and Calsa Jr. , T . 2011 . “ In silico identification of plant-derived antimicrobial peptides ” . In Systems and computational biology – molecular and cellular experimental systems , 249 – 272 . Rijeka , , Croatia : InTech .
- Pestana-Calsa , MC , Ribeiro , ILAC and Calsa , Jr T . 2010 . Bioinformatics-coupled molecular approaches for unravelling potential antimicrobial peptides coding genes in Brazilian native and crop plant species . Curr Protein Peptide Sci , 11 : 199 – 209 .
- Piotto , SP , Sessa , L , Concilio , S and Iannelli , P . 2012 . YADAMP: yet another database of antimicrobial peptides . Int J Antimicrob Ag , 39 : 346 – 351 .
- Podda , E , Benincasa , M , Pacor , S , Micali , F , Mattiuzzo , M , Gennaro , R and Scocchi , M . 2006 . Dual mode of action of Bac7, a proline-rich antibacterial peptide . Biochim Biophys Acta , 1760 : 1732 – 1740 .
- Pokorny , A , Birkbeck , TH and Almeida , PFF . 2002 . Mechanism and kinetics of δ-Lysin interaction with phospholipid vesicles . Biochemistry , 41 : 11044 – 11056 .
- Qi , X , Poernomo , G , Wang , K , Chen , Y , Chan-Park , MB , Xu , R and Chang , MW . 2011 . Covalent immobilization of nisin on multi-walled carbon nanotubes: superior antimicrobial and anti-biofilm properties . Nanoscale , 3 : 1874 – 1880 .
- Raj , PA , Marcus , E and Sukumaran , DK . 1998 . Structure of human salivary histatin 5 in aqueous and nonaqueous solutions . Biopolymers , 45 : 51 – 67 .
- Rapaport , D and Shai , Y . 1991 . Interaction of fluorescently labeled pardaxin and its analogues with lipid bilayers . J Biol Chem , 266 : 23769 – 23775 .
- Rathinakumar , R and Wimley , WC . 2008 . Biomolecular engineering by combinatorial design and high-throughput screening: small, soluble peptides that permeabilize membranes . J Am Chem Soc , 130 : 9849 – 9858 .
- Reddy , KVR , Shahani , SK and Meherji , PK . 1996 . Spermicidal activity of magainins: in vitro and in vivo studies . Contraception , 53 : 205 – 210 .
- Rex , JH , Alexander , BD , Andes , D , Arthington-Skaggs , B , Brown , SD , Chaturvedi , V , Ghannoum , MA , Espinel-Ingroff , A , Knapp , CC Ostrosky-Zeichner , L . 2008 . Reference method for broth dilution antifungal susceptibility testing of yeasts; approved standard – third edition. Wayne (PA): Clinical and Laboratory Standards Institute (CLSI). p. 1–35
- Rex , JH , Ghannoum , MA , Alexander , BD , Andes , D , Brown , SD , Diekema , DJ , Espinel-Ingroff , A , Fowler , CL , Johnson , EM Knapp , CC . 2009 . Method for antifungal disk diffusion susceptibility testing of yeasts; approved guideline – second edition. Wayne (PA): Clinical and Laboratory Standards Institute (CLSI). p. 1–23
- Rolland , JL , Abdelouahab , M , Dupont , J , Lefevre , F , Bachère , E and Romestand , B . 2010 . Stylicins, a new family of antimicrobial peptides from the Pacific blue shrimp Litopenaeus stylirostris . Mol Immunol , 47 : 1269 – 1277 .
- Rosa , R and Barracco , M . 2010 . Antimicrobial peptides in crustaceans . Invertebr Surviv J , 7 : 262 – 284 .
- Rossi , LM , Rangasamy , P , Zhang , J , Qiu , X-Q and Wu , GY . 2008 . Research advances in the development of peptide antibiotics . J Pharm Sci , 97 : 1060 – 1070 .
- Rubinchik , E , Dugourd , D , Algara , T , Pasetka , C and Friedland , HD . 2009 . Antimicrobial and antifungal activities of a novel cationic antimicrobial peptide, omiganan, in experimental skin colonisation models . Int J Antimicrob Ag , 34 : 457 – 461 .
- Sai , KP , Reddy , PN and Babu , M . 1995 . Investigations on wound healing by using amphibian skin . Indian J Exp Biol , 33 : 673 – 676 .
- Sang , Y and Blecha , F . 2008 . Antimicrobial peptides and bacteriocins: alternatives to traditional antibiotics . Anim Health Res Rev , 9 : 227 – 235 .
- Sarig , H , Livne , L , Held-Kuznetsov , V , Zaknoon , F , Ivankin , A , Gidalevitz , D and Mor , A . 2010 . A miniature mimic of host defense peptides with systemic antibacterial efficacy . FASEB J , 24 : 1904 – 1913 .
- Savage , PB , Li , C , Taotafa , U , Ding , B and Guan , Q . 2002 . Antibacterial properties of cationic steroid antibiotics . FEMS Microbiol Lett , 217 : 1 – 7 .
- Schober , D , Boeker , M , Bullenkamp , J , Huszka , C , Depraetere , K , Teodoro , D , Nadah , N , Choquet , R , Daniel , C and Schulz , S . 2010 . The DebugIT Core Ontology: semantic integration of antibiotics resistance patterns . Stud Health Technol Inform , 160 : 1060 – 1064 .
- Seebah , S , Suresh , A , Zhuo , S , Choong , YH , Chua , H , Chuon , D , Beuerman , R and Verma , C . 2007 . Defensins knowledgebase: a manually curated database and information source focused on the defensins family of antimicrobial peptides . Nucleic Acids Res , 35 : D265 – D268 .
- Seshadri Sundararajan , V , Gabere , MN , Pretorius , A , Adam , S , Christoffels , A , Lehväslaiho , M , Archer , JAC and Bajic , VB . 2011 . DAMPD: a manually curated antimicrobial peptide database . Nucleic Acids Res , 40 : D1108 – D1112 .
- Settles , B . 2005 . ABNER: an open source tool for automatically tagging genes, proteins and other entity names in text . Bioinformatics , 21 : 3191 – 3192 .
- Shen , CJ , Kuo , TY , Lin , CC , Chow , LP and Chen , WJ . 2010 . Proteomic identification of membrane proteins regulating antimicrobial peptide resistance in Vibrio parahaemolyticus . J Appl Microbiol , 108 : 1398 – 1407 .
- Shi , Y , Song , W , Feng , Z , Zhao , Y , Li , F and Tian , Y . 2009 . Disinfection of maxillofacial silicone elastomer using a novel antimicrobial agent: recombinant human beta-defensin-3 . Eur J Clin Microbiol , 28 : 415 – 420 .
- Shukla , A , Fleming , KE , Chuang , HF , Chau , TM , Loose , CR , Stephanopoulos , GN and Hammond , PT . 2010 . Controlling the release of peptide antimicrobial agents from surfaces . Biomaterials , 31 : 2348 – 2357 .
- Silverstein , KAT , Graham , MA , Paape , TD and VandenBosch , KA . 2005 . Genome organization of more than 300 defensin-like genes in Arabidopsis . Plant Physiol , 138 : 600 – 610 .
- Singh , PK , Parsek , MR , Greenberg , EP and Welsh , MJ . 2002 . A component of innate immunity prevents bacterial biofilm development . Nature , 417 : 552 – 555 .
- Smet , K and Contreras , R . 2005 . Human antimicrobial peptides: defensins, cathelicidins and histatins . Biotechnol Lett , 27 : 1337 – 1347 .
- Smith , AB , Daly , NL and Craik , DJ . 2011 . Cyclotides: a patent review . Expert Opin Ther Pat , 21 : 1657 – 1672 .
- Smith , TF and Waterman , MS . 1981 . Identification of common molecular subsequences . J Mol Biol , 147 : 195 – 197 .
- Spížek , J , Novotná , J , Řezanka , T and Demain , A . 2010 . Do we need new antibiotics? The search for new targets and new compounds . J Ind Microbiol Biot , 37 : 1241 – 1248 .
- Splith , K and Neundorf , I . 2011 . Antimicrobial peptides with cell-penetrating peptide properties and vice versa . Eur Biophys J , 40 : 387 – 397 .
- Stec , B . 2006 . Plant thionins – the structural perspective . Cell Mol Life Sci , 63 : 1370 – 1385 .
- Strandberg , E , Tremouilhac , P , Wadhwani , P and Ulrich , AS . 2009 . Synergistic transmembrane insertion of the heterodimeric PGLa/magainin 2 complex studied by solid-state NMR . Biochim Biophys Acta , 1788 : 1667 – 1679 .
- Sundararajan , VS , Gabere , MN , Pretorius , A , Adam , S , Christoffels , A , Lehväslaiho , M , Archer , JAC and Bajic , VB . 2011 . DAMPD: a manually curated antimicrobial peptide database . Nucleic Acids Res , 40 : D1108 – D1112 .
- Taboureau , O , Olsen , OH , Nielsen , JD , Raventos , D , Mygind , PH and Kristensen , H-H . 2006 . Design of novispirin antimicrobial peptides by quantitative structure-activity relationship . Chem Biol Drug Des , 68 : 48 – 57 .
- Tao , R , Tong , Z , Lin , Y , Xue , Y , Wang , W , Kuang , R , Wang , P , Tian , Y and Ni , L . 2011 . Antimicrobial and antibiofilm activity of pleurocidin against cariogenic microorganisms . Peptides , 32 : 1748 – 1754 .
- Thomas , S , Karnik , S , Barai , RS , Jayaraman , VK and Idicula-Thomas , S . 2010 . CAMP: a useful resource for research on antimicrobial peptides . Nucleic Acids Res , 38 : D774 – D780 .
- Thompson , M , Ellison , SLR and Wood , R . 2002 . Harmonized guidelines for single-laboratory validation of methods of analysis . Pure Appl Chem , 74 : 835 – 855 .
- Tian , C , Gao , B , Fang , Q , Ye , G and Zhu , S . 2010 . Antimicrobial peptide-like genes in Nasonia vitripennis: a genomic perspective . BMC Genomics , 11 : 187
- Tomasinsig , L and Zanetti , M . 2005 . The cathelicidins – structure, function and evolution . Curr Protein Peptide Sci , 6 : 23 – 34 .
- Tong , Z , Zhou , L , Jiang , W , Kuang , R , Li , J , Tao , R and Ni , L . 2011 . An in vitro synergetic evaluation of the use of nisin and sodium fluoride or chlorhexidine against Streptococcus mutans . Peptides , 32 : 2021 – 2026 .
- Torrent , M , Di , Tommaso P , Pulido , D , Victòria , Nogués M , Notredame , C , Boix , E and Andreu , D . 2011 . AMPA: an automated web server for prediction of protein antimicrobial regions . Bioinformatics , 28 : 130 – 131 .
- Tremouilhac , P , Strandberg , E , Wadhwani , P and Ulrich , AS . 2006 . Synergistic transmembrane alignment of the antimicrobial heterodimer PGLa/magainin . J Biol Chem , 281 : 32089 – 32094 .
- Tsai , P-W , Yang , C-Y , Chang , H-T and Lan , C-Y . 2011 . Human antimicrobial peptide LL-37 inhibits adhesion of Candida albicans by interacting with yeast cell-wall carbohydrates . PLoS One , 6 : e17755
- Vooturi , SK and Firestine , SM . 2010 . Synthetic membrane-targeted antibiotics . Curr Med Chem , 17 : 2292 – 2300 .
- Wakabayashi , H , Yamauchi , K , Kobayashi , T , Yaeshima , T , Iwatsuki , K and Yoshie , H . 2009 . Inhibitory effects of lactoferrin on growth and biofilm formation of Porphyromonas gingivalis and Prevotella intermedia . Antimicrob Agents Ch , 53 : 3308 – s3316 .
- Walters , MC , Roe , F , Bugnicourt , A , Franklin , MJ and Stewart , PS . 2003 . Contributions of antibiotic penetration, oxygen limitation, and low metabolic activity to tolerance of Pseudomonas aeruginosa biofilms to ciprofloxacin and tobramycin . Antimicrob Agents Ch , 47 : 317 – 323 .
- Wang , G , Li , X and Wang , Z . 2009 . APD2: the updated antimicrobial peptide database and its application in peptide design . Nucleic Acids Res , 37 : D933 – D937 .
- Wang , J , Wong , ESW , Whitley , JC , Li , J , Stringer , JM , Short , KR , Renfree , MB , Belov , K and Cocks , BG . 2011a . Ancient antimicrobial peptides kill antibiotic-resistant pathogens: Australian mammals provide new options . PLoS One , 6 : e24030
- Wang , L , Zhou , M , McClelland , A , Reilly , A , Chen , T , Gagliardo , R , Walker , B and Shaw , C . 2008 . Novel dermaseptin, adenoregulin and caerin homologs from the Central American red-eyed leaf frog, Agalychnis callidryas, revealed by functional peptidomics of defensive skin secretion . Biochimie , 90 : 1435 – 1441 .
- Wang , P , Hu , L , Liu , G , Jiang , N , Chen , X , Xu , J , Zheng , W , Li , L , Tan , M Chen , Z . 2011b . Prediction of antimicrobial peptides based on sequence alignment and feature selection methods . PLoS One , 6 : e18476
- Wang , SY , Wu , JH , Ng , TB , Ye , XY and Rao , PF . 2004 . A non-specific lipid transfer protein with antifungal and antibacterial activities from the mung bean . Peptides , 25 : 1235 – 1242 .
- Wang , W , Tao , R , Tong , Z , Ding , Y , Kuang , R , Zhai , S , Liu , J and Ni , L . 2012 . Effect of a novel antimicrobial peptide chrysophsin-1 on oral pathogens and Streptococcus mutans biofilms . Peptides , 33 : 212 – 219 .
- Wei , G-X , Xu , X and Wu , CD . 2011 . In vitro synergism between berberine and miconazole against planktonic and biofilm Candida cultures . Arch Oral Biol 56:565–572 ,
- Westerhoff , HV , Zasloff , M , Rosner , JL , Hendler , RW , De Waal , A , Gomes , AV , Jongsma , APM , Riethorst , A and Juretić , D . 1995 . Functional synergism of the magainins PGLa and magainin-2 in Escherichia coli, tumor cells and liposomes . Eur J Biochem , 228 : 257 – 264 .
- Whitchurch , CB , Tolker-Nielsen , T , Ragas , PC and Mattick , JS . 2002 . Extracellular DNA required for bacterial biofilm formation . Science , 295 : 1487
- Whitmore , L and Wallace , BA . 2004 . The Peptaibol Database: a database for sequences and structures of naturally occurring peptaibols . Nucleic Acids Res , 32
- Wikipedia . 2012 . List of antibiotics. Wikipedia – The Free Encyclopedia; [cited 2012 Sep 24]. Available from: http://en.wikipedia.org/wiki/List_of_antibiotics
- Wikler , MA , Cockerill , FR , Bush , K , Dudley , MN , Eliopoulos , GM , Hardy , DJ , Hecht , DW , Hindler , JF , Patel , JB Powell , M . 2009a . Performance standards for antimicrobial disk susceptibility tests; approved standard – 10th ed. Wayne (PA): Clinical and Laboratory Standards Institute (CLSI). p. 1–53
- Wikler , MA , Cockerill , FR , Bush , K , Dudley , MN , Eliopoulos , GM , Hardy , DJ , Hecht , DW , Hindler , JF , Patel , JB Powell , M . 2009b . Methods for dilution antimicrobial susceptibility tests for bacteria that grow aerobically; approved standard – 8th ed. Wayne (PA): Clinical and Laboratory Standards Institute (CLSI). p. 1–65
- Wimley , W and Hristova , K . 2011 . Antimicrobial peptides: successes, challenges and unanswered questions . J Membrane Biol , 239 : 27 – 34 .
- Wimpenny , J , Manz , W and Szewzyk , U . 2000 . Heterogeneity in biofilms . FEMS Microbiol Rev , 24 : 661 – 671 .
- Yala , J-F , Thebault , P , Héquet , A , Humblot , V , Pradier , C-M and Berjeaud , J-M . 2011 . Elaboration of antibiofilm materials by chemical grafting of an antimicrobial peptide . Appl Microbiol Biot , 89 : 623 – 634 .
- Yan , H and Hancock , REW . 2001 . Synergistic interactions between mammalian antimicrobial defense peptides . Antimicrob Agents Ch , 45 : 1558 – 1560 .
- Yan , X , Zhong , J , Liu , H , Liu , C , Zhang , K and Lai , R . 2011 . The cathelicidin-like peptide derived from panda genome is a potential antimicrobial peptide . Gene , 492 : 368 – 374 .
- Zairi , A , Tangy , F , Bouassida , K and Hani , K . 2009 . Dermaseptins and magainins: antimicrobial peptides from frogs' skin – new sources for a promising spermicides microbicides – a mini review . J Biomed Biot , 2009 : 452–567
- Zamyatnin , AA . 1991 . EROP-Moscow: specialized data bank for endogenous regulatory oligopeptides . Protein Seq Data Anal , 4 : 49 – 52 .
- Zamyatnin , AA , Borchikov , AS , Vladimirov , MG and Voronina , OL . 2006 . The EROP-Moscow oligopeptide database . Nucleic Acids Res , 34 : D261 – D266 .
- Zasloff , M . 2002 . Antimicrobial peptides of multicellular organisms . Nature , 415 : 389 – 395 .
- Zhang , R , Zhou , M , Wang , L , McGrath , S , Chen , T , Chen , X and Shaw , C . 2010 . Phylloseptin-1 (PSN-1) from Phyllomedusa sauvagei skin secretion: a novel broad-spectrum antimicrobial peptide with antibiofilm activity . Mol Immunol , 47 : 2030 – 2037 .
- Zhang , T . 2001 . An introduction to support vector machines and other kernel-based learning methods . AI Mag , 22 : 103 – 104 .
- Zilberman , M and Elsner , JJ . 2008 . Antibiotic-eluting medical devices for various applications . J Control Release , 130 : 202 – 215 .