Abstract
Biofilms are commonly associated with an increased risk of patient infection. In peritoneal dialysis (PD), catheter associated infection, especially peritonitis, remains a clinically relevant problem. Although the presence of a biofilm is recognized in relapsing, repeat, and catheter-related peritonitis, it remains poorly characterized. In this review, an update on the role of biofilms in PD infections is presented. The emerging concept that host cells and tissue associated biofilms, in addition to the biofilms on the catheters themselves, contribute to the recalcitrance of infections is discussed. Furthermore, the evidence of biofilms on PD catheters, their developmental stages, and the possible influence of the PD environment are reviewed. The focus is given to ex vivo and in vitro studies that contribute to the elucidation of the interplay between host, microbial, and dialysis factors. The key issues that are still to be answered and the challenges to clinical practice are discussed.
Introduction
Peritoneal dialysis (PD) is a mode of renal replacement therapy with increasing acceptance due to its recognized economic benefits as well as improved well-being of the patients (Kutner et al. Citation2005; Rodrigues et al. Citation2006). This dialytic modality (Figure ) takes advantage of the peritoneal cavity as a compartment to accommodate the dialysate. The access to the peritoneal cavity is accomplished through a permanent catheter that allows the flux of fresh (unused)/spent (drained after a dwell period) dialysate from the external environment to the peritoneal cavity and vice versa (Figure a). The tip of the catheter is located intraperitoneally, the subcutaneous segment transverses the abdominal wall and the external segment emerges at an appropriately located exit-site (Figure b). Additionally, a pivotal element in the PD process is the peritoneal membrane that lines the visceral organs and the walls of the abdomen and pelvis (Figure b). Specifically, the peritoneal membrane is composed of a layer of mesothelial cells, an underlying basement membrane, an interstitial space that comprises peritoneal capillaries and some lymphatic elements, and an endothelium basement. During the dwell, ie the period in which the PD dialysate remains inside the peritoneal cavity, the solutes and water move from the blood, in the peritoneal capillaries, to the dialysate, across the peritoneal membrane by diffusion, ultrafiltration, and convection. The dwell time is variable but it is repeated periodically (Teitelbaum & Burkart Citation2003).
Figure 1 PD technique. (a) A patient is shown during an exchange. The patient is connected to the tubing set and the spent dialysate is drained out. Then, the peritoneal cavity is filled with fresh dialysate and the tubing set is disconnected. After a dwell, the process is repeated. (b) A schematic diagram indicating the relationship between the PD catheter and the adjacent tissues. The potential pathways of peritoneal cavity contamination are indicated: 1-intraluminal, 2-periluminal, and 3-transmural/hematogenous/transvaginal (adapted from Spencer (Citation1988)).
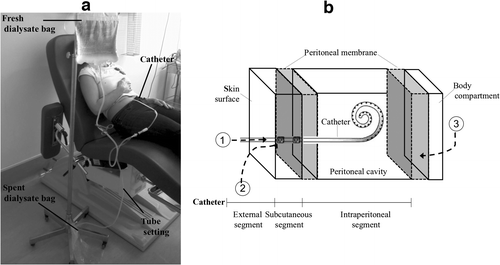
The ability of microorganisms to adhere to human tissues or biomaterials and develop aggregated communities enmeshed in an extracellular matrix of microbial and host-derived components, ie a biofilm, is commonly associated with an increased risk of patient infection. Compared to single microbial cells in suspension (ie planktonic cells), biofilms afford protection from antimicrobials and immune system effectors, and may spawn systemic infection (Hall-Stoodley & Stoodley Citation2009).
In PD, catheter associated infection, and especially peritonitis, remains a challenging cause of technique failure, ie the permanent cessation of the therapy. Cumulative clinical experience and technical advances have resulted in an improvement in patients’ survival, but only modest changes in the risk of PD technique failure are documented (Perl et al. Citation2012; Rocha et al. Citation2012). Technical failure is often associated with episodes of catheter-related, relapsing, and repeated peritonitis. The usual criteria for a diagnosis of peritonitis include at least two of the following three: a cloudy dialysate, confirmed by a white blood cell count >100 μl−1 with at least 50% of polymorphonuclear neutrophils; clinical symptoms, such as abdominal pain/tenderness; and a positive microbiological culture from the dialysate. In some cases, the dialysate culture is negative and the other two criteria are met. These cases of peritonitis are referred to as ‘sterile’ (Li et al. Citation2010). Catheter-related peritonitis is defined as peritonitis combined with an exit-site or tunnel infection (clinically evidenced by a purulent drainage from the exit-site), typically associated with the same microbial agent. A relapsing peritonitis is the return of an infection < 4 weeks after stopping the therapy for the initial episode. It occurs with the identification of the same microbial species or sometimes with a sterile episode. Contrastingly, a repeat peritonitis is defined as the repetition of the infection > 4 weeks after the completion of the therapy for the prior episode and is caused by the same microbial species (Li et al. Citation2010). Although repeat episodes of peritonitis generally have a satisfactory response to antimicrobial therapy, they are associated with a considerable risk of developing a relapsing episode (Szeto et al. Citation2011). Coagulase negative staphylococci (CNS) are the most common cause of these events, presenting low complete cure rates in comparison with other infections (54.4% vs 76.6%; Szeto et al. Citation2008). Notably, the biofilm on the catheter is presumed to be associated with the occurrence of relapsing and repeat peritonitis (Piraino et al. Citation2011), but this hypothesis remains poorly characterized. Inadequate treatment of peritonitis, particularly through low levels of antibiotics in the dialysate, which may not be adequate to reach biofilms (Dasgupta et al. Citation1992), elevates the risk of technique failure. Therefore, understanding the characteristics and development of biofilms is important to promote preventive and adjuvant therapy strategies in PD.
In the 1990s, with the introduction of improvements in the PD technique, a drop in the rate of infections caused by Gram-positive microorganisms associated with the formation of biofilm on the catheter was observed (Li & Chow Citation2012; Rocha et al. Citation2012), probably discouraging the scientific community from proceeding further with research on biofilms. However, the trends in microbiological infections point to an increase in episodes of peritonitis caused by Gram-negative bacteria and repeat/relapsing episodes, whose poor outcomes are associated with the presence of biofilm (Li & Chow Citation2012). So, research into biofilms associated with PD is increasingly relevant. In this review, an update on PD biofilms on host tissues and catheters will be presented. The focus will be ex vivo and in vitro studies that contribute to the elucidation of the interplay between host, microbial, and dialysis factors. Through the integration of these concepts, key research objectives and challenges to clinical practice will be highlighted.
Potential sources of peritoneal cavity contamination
As the peritoneal cavity is an ‘open system,’ microbial entry may occur by one of five pathways (Figure b). In the intraluminal and periluminal pathways, environmental or commensal microorganisms enter the lumen and the cutaneous/subcutaneous catheter tract, respectively, through touch, cracks in the tubing/catheter, or the dialysate. In the transmural pathway, enteric microorganisms reach the peritoneal cavity as a consequence of gastrointestinal tract inflammation/perforation or constipation. Alternatively, in the transvaginal pathway, microorganisms of gynecologic origin contaminate the peritoneal cavity, as a result of vaginal leaks, vaginal delivery, or hysteroscopy. Finally, in the hematogenous pathway, bacteria associated with dental procedures, intravascular devices, or ascites are the main source of contamination (von Graevenitz & Amsterdam Citation1992; Vas & Oreopoulos Citation2001; Piraino et al. Citation2011). The intraluminal and periluminal pathways are considered the most common routes of infection (Vas & Oreopoulos Citation2001), being frequently associated with commensal microorganisms, namely Staphylococcus sp.
One of the first challenges in clinical practice is to minimize the contamination of the PD environment. Currently, infection prevention relies mainly on high quality training protocols and exit-site management. Furthermore, the eradication of Staphylococcus aureus in nasal carriers is recommended once the patient has been identified as a carrier, preferably before catheter implantation (Piraino et al. Citation2005). However, the benefit of screening and treating for nasal S. aureus is under debate (Li et al. Citation2010). In fact, in some cases there is a discrepancy between the strains in carriage and those causing infection. For example, it was found that 3/17 patients with S. aureus and 4/10 patients with CNS peritonitis had different strains of the same species at the exit-site cf. the nares or nails (Amato et al. Citation2001); and that 6/9 (Eisenberg et al. Citation1987) and 3/6 (Horsman et al. Citation1986) patients with CNS peritonitis had different strains in the dialysate and skin. This inconsistency may be related to carriage at other sites than the nares, such as the pharynx, or possibly because of transience in nasal carriage (Eisenberg et al. Citation1987). Although microbial carriage is not well understood, it has been proposed that it is a multistep process involving the differential expression of host and microbial factors (Weidenmaier et al. Citation2012), but the role of biofilm in S. aureus nasal carriage remains controversial (Krismer & Peschel Citation2011). Specifically, the evaluation of the adaptations of S. aureus nasal strains by ex vivo transcriptomics (Burian et al. Citation2010) and in vitro exoproteomics (Muthukrishnan et al. Citation2011) suggested that strains isolated from carrier patients are able to evade the immune system (Burian et al. Citation2010, Muthukrishnan et al. Citation2011), a tendency that is augmented in the biofilm mode of growth (Muthukrishnan et al. Citation2011).
Nevertheless, upon microbial entry into the PD environment, it may be that an immediate infection frequently does not occur (Vas & Oreopoulos Citation2001; Silverstein & Wilcox Citation2010), because of microbial resistance mechanisms, such as biofilm formation.
Contribution of microbial biofilms to PD infections
Host cell/tissue associated biofilms
It is being increasingly recognized that microbial species such as S. aureus, traditionally considered extracellular, can enter and replicate within host cells, adopting an intracellular life-cycle (Fraunholz & Sinha Citation2012; Silva Citation2012). In the PD setting, there is some evidence of microorganisms having an intracellular component. Specifically, in vitro studies have shown that Staphylococcus epidermidis is able to survive and grow within leucocytes (Bronswijk et al. Citation1990). Furthermore, microorganisms have been observed within leucocytes of spent dialysates (Ludlam et al. Citation1988; Taylor Citation1994), specifically S. aureus has been described within neutrophils in the spent dialysate of patients with relapsing peritonitis (Buggy et al. Citation1984). Furthermore, indirect evidence of S. aureus and Enterobacter agglomerans sequestration by leucocytes was provided by the successful treatment of a refractory peritonitis with intraperitoneal clindamycin (Cohen & Bailey Citation1984), an antimicrobial that is able to penetrate leucocytes (Jacobs & Wilson Citation1983). Therefore, diagnostic (Keane et al. Citation2000) and therapeutic practices (Li et al. Citation2010) were modified taking into account a possible intracellular life-cycle, thus contributing to the lack of strong scientific evidence to support the role of this biological process in chronic infections. The literature suggests that the intracellular survival of S. aureus is regulated by microbial oxidative stress responses, adhesion, production of toxins and exoproteins, and regulation of the cell death mechanisms of phagocytes (Thwaites & Gant Citation2011). These findings point towards the intracellular lifestyle as a mechanism to evade immune and antimicrobial agents, at least for some microorganisms. However, the contribution of intracellular dissemination of pathogens in PD infections is controversial as the phagocytic and killing activity of cells is diminished due to the patients’ uremic state, the use of fresh dialysate (Cameron Citation1995), and because of clearance of phagocytes during the PD exchanges.
Additionally, damaged tissues, that can be found in the PD environment, have ligands and nutrients that support microbial attachment and further biofilm development (Gristina Citation1987). The principal evidences of microbial adhesion on the peritoneal membrane, mesothelial cells, and exit-site epithelium come from in vitro studies. First, the adherence of staphylococci to mesothelial cells has been shown (Haagen et al. Citation1990; Betjes et al. Citation1992; Glancey et al. Citation1993), although S. aureus adhered to a greater extent (Haagen et al. Citation1990; Betjes et al. Citation1992) and elicited higher cytotoxic effects (Haslinger-Loffler et al. Citation2006) than S. epidermidis. Second, strains of S. aureus recovered from patients with exit-site infection (ESI) were found to adhere to epithelial cells, although the degree of adherence did not correlate with the severity of the infection (Kreft et al. Citation1998). Furthermore, a study using a rat model showed that S. aureus and Pseudomonas aeruginosa, but not S. epidermidis and Candida albicans, were able to form adhesions around the tunnel, which may contribute to the process of infection (Findon & Miller Citation1995). Although fibronectin binding proteins have been implicated indirectly in the staphylococci–host cell interactions (Glancey et al. Citation1993), this process may involve other factors (reviewed in Heilmann Citation2011) whose contribution in PD is not yet known.
Although the role of microbial-phagocytic and microbial non-phagocytic cell adhesion, and eventually intracellular biofilm-like communities are under-recognized in PD, in other research areas pathogen interaction with host cells has been the focus of increasing attention with respect to the microbial phenotypes associated with infection chronicity. One area of increasing interest is the role of the small colony variant (SCV) phenotype adopted by bacteria within non-phagocytic host cells (Sendi & Proctor Citation2009). From the better studied pathogen, S. aureus, it has been shown that the SCV phenotype is characterized by the increased expression of fibronectin binding proteins and decreased expression of exotoxins, that facilitate microbial adherence and residence within host cells, and the concomitant low metabolic activity, that is associated with diminished aminoglycosides susceptibility (Sendi & Proctor Citation2009; Tuchscherr et al. Citation2011). Furthermore, it has been hypothesized that the SCV are selected from biofilms under stress conditions, thus being associated with biofilm recalcitrance to antimicrobials (Garcia et al. Citation2013).
Abscesses have also been referred to recently as ‘reminiscent of biofilm communities formed in vitro’ (Cheng et al. Citation2011). Specifically, S. aureus was found within a pseudocapsule and was still able to replicate (Cheng et al. Citation2011). It should be noted that S. aureus, P. aeruginosa (Vas & Oreopoulos Citation2001), and fungal (C. albicans, Candida tropicalis and Rhodotorula rubra; Eisenberg et al. Citation1986) peritonitis have been associated with abscess formation; and that abscesses have been observed in the cuffs retrieved from patients with relapsing peritonitis (Dimitriadis et al. Citation1990), although an association with a biofilm phenotype has yet to be established.
PD catheter associated biofilms
The microorganisms that enter the PD environment invariably come into contact with the catheter (Figure b). In the past 30 years, although researchers have evaluated the microbial biofilms on PD catheters (Table ), this topic remains poorly investigated, as can be inferred by the low number of recent publications (since 2010 only two works have been published). Furthermore, it should be noted that these studies have a few caveats: (1) insufficient characterization of the microbial populations, dialysis procedures, and established antimicrobial therapies; (2) the disparity in the indications for catheter removal; (3) the small size of populations; (4) the absence of standardization in the collection of catheters; and (5) the lack of standardization in the microbiological procedures. However, the recent clinical focus on repeat/relapsing peritonitis may bring a new interest to the investigation and role of biofilms in PD.
Table 1. Summary of studies carried out on PD catheters supporting the presence of a microbial biofilm.
The process of biofilm formation on catheters is complex and depends on multiple factors. The initial event is the formation of a conditioning film, which is determined by the surface properties of the material (silicone, for Tenckhoff catheters) and the immune response to the catheter itself. Animal studies have shown that the implantation of a catheter induces peritoneal structural changes and inflammation, with the catheter surface being covered by a conditioning film of host-derived cells that parallel those found in the peritoneum, ie mesothelial cells, T cells, fibroblastic cells (fibroblasts and myofibroblasts), macrophages, and dendritic cells (Flessner et al. Citation2010; Peters et al. Citation2011). Additionally, the initiation of PD may contribute to the changes in the catheter surface due to the dialysate (Gorman et al. Citation1993). Indeed, host-derived cells, such as leucocytes, erythrocytes, macrophages, mesothelial cells, and fibroblasts (Dasgupta et al. Citation1987; Swartz et al. Citation1991; Gorman et al. Citation1994; Bayston et al. Citation1999; Guerra e Silva et al. Citation2011) and proteins, such as albumin, transferrin, IgG, fibrinogen, fibronectin, von Willebrand factor, vitronectin, and thrombospondin, have been observed (Yanagisawa et al. Citation2004). Furthermore, scanning electron microscopy (SEM) analyses of transfer sets that have been in use for 6 months in two separate patients showed a film that appears to have a variable thickness comprising calcium, carbon, oxygen, and chloride, as indicated by energy-dispersive X-ray analysis (EDX; Figure a-i vs b-i). In addition, the deposition of calcium encrustations was observed occasionally (Figure a-ii and b-ii), probably arising from the dialysate. Therefore, an important consideration is that the conditioning film can markedly modify the catheter surface including roughness, hydrophobicity, and physico-chemical properties.
Figure 2 Example of SEM and EDX analyses performed on the intraluminal surface of transfer sets removed from two patients (a and b). Biofilm overview (i) and specific encrustations (ii) observed by SEM are shown as inserts to the corresponding EDX spectrum illustrating the elemental composition. Au (from sputtering-coating of samples) and Si (from the catheter wall) are not elements originating from the biofilms.
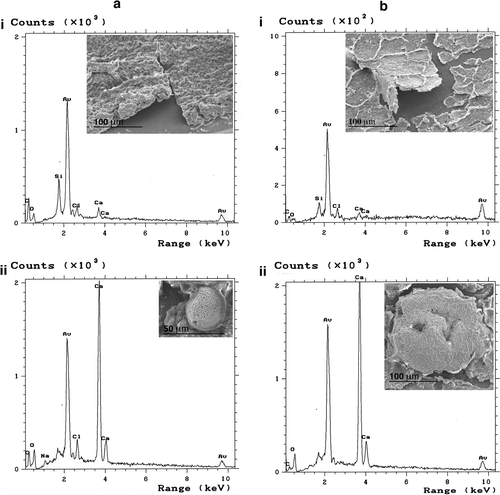
However, microbial cells are often isolated from catheters (Table ), providing evidence of the ability of the microorganisms to overcome the surface physico-chemical constraints and the environmental conditions, namely the dialysate. The dialysate may affect the growth of microbial cells. The fresh dialysates, namely the conventional ones, have been shown to be bacteriostatic against P. aeruginosa (Shalit et al. Citation1985), Streptococcus sanguinis (Hermsen et al. Citation2003; Hotchkiss et al. Citation2004), S. aureus (Buggy et al. Citation1984; Hermsen et al. Citation2003; Hotchkiss et al. Citation2004), and S. epidermidis (Reid et al. Citation1990; Hermsen et al. Citation2003; Hotchkiss et al. Citation2004). In S. aureus (Buggy et al. Citation1984), but not S. epidermidis (MacDonald et al. Citation1986), this has been attributed to the low pH of the dialysate. However, it should be noted that the pH of the dialysate increases over the course of the exchange (Cameron Citation1995). In addition, the biocompatible dialysate formulations (lactate-buffered glucose, lactate/bicarbonate glucose, icodextrin, amino acid, and low calcium) have been shown to inhibit the growth of S. aureus (Clouse et al. Citation2007; Tobudic et al. Citation2011, Citation2012), and S. epidermidis, Enterococcus faecalis, P. aeruginosa, and C. albicans (Tobudic et al. Citation2011). However, the icodextrin and amino acid dialysates were suggested to be less detrimental than the others (Tobudic et al. Citation2011, Citation2012). Notably, the spent dialysate from conventional solutions may support the growth of microorganisms, namely P. aeruginosa (Shalit et al. Citation1985) and S. epidermidis (MacDonald et al. Citation1986), but it is not known how biocompatible spent dialysates affect microbial cells.
The dialysate may also affect microbial adhesion and further biofilm development, as demonstrated by some in vitro studies. First, it was shown that the adherence of staphylococci to silicone coated with spent dialysate is lower than to silicone coated with phosphate-buffered saline (Gorman et al. Citation1993, Citation1997), but higher than to silicone coated with fresh dialysate (Yanagisawa et al. Citation2004). Second, while the ability of S. epidermidis to form biofilms in spent dialysate is greater than that in fresh dialysate, the ability of P. aeruginosa and Escherichia coli to form biofilm is not affected differentially (Dasgupta et al. Citation1992), suggesting a species dependent effect. Importantly, the effect of the biocompatible solutions in microbial adhesion and biofilm formation is still unknown.
Despite all these constraints, biofilms are frequently observed on PD catheters (Table ), with staphylococci and Pseudomonas spp. being the most frequently recovered Gram-positive and Gram-negative species by culturable methods (Table ). In contrast, fungi are rarely observed. Notably, the infections associated with these microorganisms are associated with poor outcomes (Li & Chow Citation2012). It should be stressed that to the authors’ knowledge, broad-spectrum polymerase chain reaction (PCR) or quantitative bacterial DNA PCR techniques have not been used yet to characterize the biofilm on PD catheters. S. aureus was consistently associated with catheters removed due to ESI, whether associated with peritonitis or not (Table ). Furthermore, the range of bacteria and fungal species isolated from catheters of patients with peritonitis was broader than with ESI, but staphylococci were more frequently reported. Importantly, the ability of CNS to form biofilm in vitro does not correlate with infection, or its resolution (Horsman et al. Citation1986; Kristinsson et al. Citation1986; Barretti et al. Citation2009, Citation2012). Accordingly, staphylococci were commonly observed associated with catheters explanted from patients without infection (Table ). Although in the population of patients without infection, a major occurrence of negative cultures was observed, the percentage within each study ranged from 16% (Dasgupta et al. Citation1987) to 100% (Verger et al. Citation1987), being 40% in the most recent study (Pihl et al. Citation2013). However, it should be remarked that negative cultures were also observed on catheters explanted from patients with peritonitis (Dasgupta et al. Citation1986, Citation1987). This can, in part, be explained by the effect of active antimicrobial therapies and by the culture methods employed, which may not recover efficiently the microorganisms from the catheter, or the cultivation conditions that may not be adequate for the growth of viable but not culturable biofilm microorganisms (Hall-Stoodley et al. Citation2012). Finally, it may be stressed that polymicrobial cultures are not reported frequently, although it has been observed that in a population from which the catheter has been removed for non-infectious reasons (renal transplant), polymicrobial cultures can attain 41% of the total positive cultures (Gorman et al. Citation1994), suggesting an influence of the immune system in the colonization of the catheter.
Table 2. Microbiological findings on PD catheters.
Within biofilms, microbial cells are in a structured community held together by the extracellular matrix, that is variously referred to as ‘amorphous accretion’ (Marrie et al. Citation1983), ‘cementing material’ (Locci et al. Citation1984) or ‘film matrix’ (Gorman et al. Citation1994). Biofilms have been found on the intra- and extraluminal surfaces of the catheter (Dasgupta et al. Citation1987; Gorman et al. Citation1994; Guerra e Silva et al. Citation2011; Pihl et al. Citation2013) with an uneven distribution, presenting areas with different degrees of colonization along the catheter (Marrie et al. Citation1983; Dasgupta et al. Citation1987). A tendency to accumulate at the catheter pores was reported (Gorman et al. Citation1994), which may in part be explained by the hydrodynamic and shear forces inherent to the PD exchanges. Interestingly, there was no major correlation between the features of the biofilm and the catheter dwell time (0.5, 1, 1.5, and 8 years), as assessed by SEM (Guerra e Silva et al. Citation2011). The microbial cell density within biofilms on PD catheters has not been specified, albeit in one study (Bayston et al. Citation1999) the number of culturable cells recovered from the flushed fluid in the catheter lumen ranged from 1 to 107–109 colony forming units ml−1. The microbial density may impact significantly on the outcome of the infection (Hotchkiss et al. Citation2004). Overall, these studies (Table ) demonstrate the presence of a microbial biofilm on the catheter surface, despite the absence of infection, suggesting that it is not the infectious process that gives rise to colonization, but rather that biofilms may act as a reservoir of microorganisms. Indeed, it has been hypothesized that the development of peritonitis depends on the initiation of dialysis (Read et al. Citation1989; McDermid et al. Citation1993) and on the ability of microorganisms to disperse from the biofilm (Verger et al. Citation1987; Dasgupta et al. Citation1992). However, further studies are required to elucidate the biofilm-associated infections in PD, as it was found that the microorganisms colonizing the catheter may not parallel those causing the peritonitis (Marrie et al. Citation1983; Rodriguez-Carmona et al. Citation2000).
The contribution of the host’s immunity in protection against biofilm microbial cells is considered an independent variable for the elimination of microorganisms. Studies performed with several microorganisms suggest that cells within biofilms may be tolerant to phagocytosis, phagocytic killing, and antibodies (Arciola et al. Citation2012). Accordingly, the inability of the host immune system to clear P. aeruginosa biofilm infections in the peritoneal cavity has been shown, even in pre-immunized animals (Ward et al. Citation1992). Furthermore, biofilms of S. epidermidis grown in spent dialysate were found to induce a reduction in the production of gamma-interferon, cf. planktonic cells (Dasgupta Citation1996). These findings suggest that the biofilm phenotype may hamper several lines of the immune defenses, but the full picture is far from being understood.
The antimicrobial tolerance of biofilm cells
The use of antimicrobials is the most common management strategy for PD infections and is based on the minimal inhibitory concentration (MIC) of the drug for planktonic cells (a complete list of the antimicrobials and dosages commonly prescribed can be found in Li et al. (Citation2010)). However, the activity of antimicrobials may be influenced by several factors such as the mode of microbial growth or the PD environment, and as for peritonitis treatment the intraperitoneal administration of antimicrobials is commonly recommended (Li et al. Citation2010). In general, planktonic cells are more sensitive to antimicrobial agents than their counterparts in a biofilm, although some isolates exhibit resistance (Salzer Citation2005; Borras Citation2009; Pihl et al. Citation2013). Antimicrobial resistance does not seem to be an uncontrolled threat in PD at the present time, but it has been suggested that the widespread use of antimicrobials may accelerate its occurrence (Li et al. Citation2010). However, when the antimicrobial sensitive planktonic populations grow in biofilm form, they become resistant or tolerant to antimicrobials, a phenomenon recently designated by phenotypic ‘tolerance’ to distinguish it from the heritable ‘resistance’ (Nathan Citation2012). Yet, not fully understood, biofilm tolerance is multifactorial and for bacterial biofilms it has been suggested to be linked with the decreased penetration of some antimicrobials (Davies Citation2003; Kalan & Wright Citation2011), ‘quorum sensing related systems’, ie regulation of gene expression in response to critical cell density, faster exchange of genetic material (del Pozo & Patel Citation2007), and the presence of persister cells (a subpopulation of cells with a reduced metabolism and growth rate; Davies Citation2003; del Pozo & Patel Citation2007). For fungal biofilms, namely Candida spp., the increased tolerance to antifungals has been associated with the expression of drug resistance genes and persister cells (Seneviratne et al. Citation2008). The antimicrobial susceptibility of biofilm strains isolated from the PD dialysate has been evaluated in vitro (Dasgupta et al. Citation1991, Citation1997; Sepandj et al. Citation2003, Citation2004; Girard et al. Citation2010). As expected, these studies showed that generally the antimicrobial minimum biofilm eradication concentrations (MBEC) are within the drug resistance range for biofilms, in contrast to the MIC for planktonic cells that fall within the sensitivity levels. Thus, these results suggest that the routine antimicrobial sensitivity tests underestimate the antimicrobial tolerance of biofilm cells. Furthermore, it should be noted that neither MIC/MBEC values take into account the high drug concentration of antibiotics administered intraperitoneally or the effect of the dialysate on the bioavailability and bioeffectiveness of drugs (Sepandj et al. Citation2003; de Vin et al. Citation2009).
Studies of planktonic cell populations showed that, generally, several antimicrobials used against planktonic P. aeruginosa (Shalit et al. Citation1985; Zelenitsky et al. Citation2002) staphylococci, and Streptococcus sp. (Ludlam et al. Citation1992; Zelenitsky et al. Citation2002; Hermsen et al. Citation2003; Clouse et al. Citation2007) were less active in dialysate than in broth medium. Yet, the activity of daptomycin against Gram-positive organisms seems to be less affected (Hermsen et al. Citation2003; Tobudic et al. Citation2012). The literature suggests that the effect of the dialysate may not depend on its pH (fresh vs spent; Hermsen et al. Citation2003) or composition (Zelenitsky et al. Citation2002; Tobudic et al. Citation2012) although carbon dioxide levels may have an influence (Wilcox et al. Citation1990). The effect of the dialysate against the antimicrobial susceptibility of biofilm cells has only been addressed on CNS (Richards et al. Citation1992; Wilcox et al. Citation1992; Gagnon et al. Citation1993). These studies indicate that the tolerance of biofilm cells in the presence of dialysate depends on the composition of the dialysate (fresh vs spent) and the antimicrobial compound. Notably, rifampicin, which has been assumed to be one of the most effective antimicrobial agents against biofilms of S. epidermidis, does not eliminate the microbial cells when a broth medium is used. However, in the presence of dialysate (fresh or spent), rifampicin was effective in killing biofilm cells of S. epidermidis (Richards et al. Citation1992; Gagnon et al. Citation1993).
It is of note that the use of antimicrobials is associated with an increased risk of fungal peritonitis, due to fungal overgrowth concurrent to disturbances in the bacterial flora and diminished peritoneal defenses. As this represents the most severe type of infection in PD patients (Li & Chow Citation2012), particular attention should be given to the prolonged or repeated use of antimicrobials. Thus, to overcome the consequences of long antimicrobial therapies and to increase the killing of bacterial growth and limit the onset of drug resistance (Zhao & Drlica Citation2001), the use of antimicrobial combinations has been recommended (Li et al. Citation2010). Indeed, certain antibiotic combinations can be more effective against biofilm cells than monotherapies (Sepandj et al. Citation2003, Citation2004; Girard et al. Citation2010). However, further investigation is required as a study by Torella et al. (Citation2010) based on a population genetic model, instead suggested that combinational therapy may actually increase the risk of multi-drug resistance.
Alternatively, the microbial ‘adjunctive’ therapies are seen as promising strategies to improve antimicrobial efficacy and to reduce the development of drug resistance. These therapies are based on the use of agents that may or may not have antimicrobial properties but target the extracellular matrix components of biofilms, such as fibrin (Kumar et al. Citation2010). In PD, the empirical use of these agents, namely heparin, urokinase, streptokinase, and tissue plasminogen activator has been suggested for catheter function restoration (Dombros et al. Citation2005), but there is no agreement with respect to their beneficial effects on peritonitis treatment (Li et al. Citation2010). Due to their potential benefits, antibiofilm therapeutics requires further investigation, including rigorous clinical trials.
Conclusions
The pathogenesis of PD infections is multifactorial, depending on microbial, host, and dialytic factors. Particularly, the outcome of relapsing/refractory peritonitis is poor, and often the recommendation is the removal and replacement of the catheter to protect the peritoneal membrane. The studies reviewed here suggest that the formation of biofilm on host cells, tissues, and catheters are not associated with acute infections, per se, but rather act as a secondary source of infection contributing to their recalcitrance. However, determinants of biofilm-associated infections are mainly under-recognized, hampering preventive and adjuvant therapy strategies.
It is indisputable that the strategies developed over the years to prevent peritoneal cavity contamination have reduced the incidence of infections. However, considering the contribution of the intraluminal pathway, new approaches may encompass the control of skin microorganisms.
The current treatment options aim to reduce the burden of planktonic cells and do not take into account the biofilm lifestyle. The under-recognized intracellular lifestyle and catheter associated biofilms may significantly impact infection management, as cells are protected against host immunity and antimicrobial agents. Therefore, the determination of drug MBEC has been suggested in cases of relapsing and refractory peritonitis (Sepandj et al. Citation2004). From the studies reviewed, further progress in the management of biofilm-associated infections requires additional knowledge of biofilms in PD, including the identification and characterization of tissue associated biofilms, the elucidation of the interplay of microbial, host, and dialysis factors contributing to colonization, infection, and tolerance to environmental conditions.
So, while definitive diagnostic guidelines for biofilm-associated infections are not available, the patient’s clinical history of chronic or recurrent infection and the lack of response to antimicrobial treatment have been suggested as criteria (Hall-Stoodley et al. Citation2012). Further knowledge of this area will impact on PD clinical outcomes but will also be relevant in other modalities of dialysis and fields of medicine using internal devices.
Acknowledgments
M. Martins is financially supported by a fellowship from the Portuguese Foundation for Science and Technology (contract SFRH/BPD/73663/2010), financed by the European Social Fund and national funds from the Ministry of Education and Science. The authors are grateful to the Portuguese Society of Nephrology for financial support. The authors are indebted to the staff of the Department of Nephrology of Hospital de Santo António, Centro Hospitalar do Porto, namely Olívia Santos, for sample collection.
Notes
Professor Rosário Oliveira passed away just after accomplishing the design and revision of this manuscript. She will always be remembered as an exceptional scientist, teacher, and a true friend. She dedicated her scientific life to biofilm science being the founder and the soul of the UMinho Biofilm Group.
References
- Amato D, Ventura MJ, Miranda G, Leanos B, Alcantara G, Hurtado ME, Paniagua R. 2001. Staphylococcal peritonitis in continuous ambulatory peritoneal dialysis: colonization with identical strains at exit site, nose, and hands. Am J Kidney Dis. 37 : 43–48.
- Arciola CR, Campoccia D, Speziale P, Montanaro L, Costerton JW. 2012. Biofilm formation in Staphylococcus implant infections: a review of molecular mechanisms and implications for biofilm-resistant materials. Biomaterials. 33 : 5967–5982.
- Barretti P, Montelli AC, Batalha JE, Caramori JC, Cunha ML. 2009. The role of virulence factors in the outcome of staphylococcal peritonitis in CAPD patients. BMC Infect Dis. 9 : 212.
- Barretti P, Moraes TM, Camargo CH, Caramori JC, Mondelli AL, Montelli AC, Cunha ML. 2012. Peritoneal dialysis-related peritonitis due to Staphylococcus aureus: a single-center experience over 15 years. PLoS ONE. 7 : e31780.
- Bayston R, Andrews M, Rigg K, Shelton A. 1999. Recurrent infection and catheter loss in patients on continuous ambulatory peritoneal dialysis. Perit Dial Int. 19 : 550–555.
- Betjes MG, Tuk CW, Struijk DG, Krediet RT, Arisz L, Beelen RH. 1992. Adherence of staphylococci to plastic, mesothelial cells and mesothelial extracellular matrix. Adv Perit Dial. 8 : 215–218.
- Borras M. 2009. Antibiotic resistance in Gram-negative peritonitis. Perit Dial Int. 29 : 274–276.
- Bronswijk, H, Verbrugh, HA, Oe, PL, Beelen, RHJ, Verhoef, J, Donker, AJM 1990. Survival and growth of Staphylococcus epidermidis within phagocytes in relation to recurrent peritonitis in continuous ambulatory peritoneal dialysis patients. In: Morrell MA, Giordano C, editors. Ambulatory peritoneal dialysis. New York: Springer; p. 189–193.
- Buggy BP, Schaberg DR, Swartz RD. 1984. Intraleukocytic sequestration as a cause of persistent Staphylococcus aureus peritonitis in continuous ambulatory peritoneal dialysis. Am J Med. 76 : 1035–1040.
- Burian M, Wolz C, Goerke C. 2010. Regulatory adaptation of Staphylococcus aureus during nasal colonization of humans. PLoS ONE. 5 : e10040.
- Cameron JS. 1995. Host defences in continuous ambulatory peritoneal dialysis and the genesis of peritonitis. Pediatr Nephrol. 9 : 647–662.
- Cheng AG, DeDent AC, Schneewind O, Missiakas D. 2011. A play in four acts: Staphylococcus aureus abscess formation. Trends Microbiol. 1 : 225–232.
- Clouse FL, Hovde LB, Rotschafer JC. 2007. In vitro evaluation of the activities of telavancin, cefazolin, and vancomycin against methicillin-susceptible and methicillin-resistant Staphylococcus aureus in peritoneal dialysate. Antimicrob Agents Chemother. 51 : 4521–4524.
- Cohen L, Bailey D. 1984. Efficacy of intraperitoneal clindamycin refractory peritonitis-report of two cases. Perit Dial Int. 4 : 92–94.
- Dasgupta MK. 1996. Biofilm causes decreased production of interferon-gamma. J Am Soc Nephrol. 7 : 877–882.
- Dasgupta MK, Anwar H, Costerton JW. 1992. Bacterial biofilms and peritonitis in continuos ambulatory peritoneal dialysis. Int Biodeterior Biodegrad. 30 : 167–176.
- Dasgupta MK, Bettcher K, Ulan RA, Burns V, Lam K, Oossetor JB, Costerton JW. 1987. Relationship of adherent bacterial biofilms to peritonitis in chronic ambulatory peritoneal dialysis. Perit Dial Bull. 7 : 168–173.
- Dasgupta MK, Kowalewska-Grochowska K, Larabie M, Costerton JW. 1991. Catheter biofilms and recurrent CAPD peritonitis. Adv Perit Dial. 7 : 165–168.
- Dasgupta MK, Shishido H, Salama S, Singh R, Larabie M, Micetich RG. 1997. The effects of macrolide and quinolone antibiotics in methicillin-resistant Staphylococcus aureus biofilm growth. Adv Perit Dial. 13 : 214–217.
- Dasgupta MK, Ulan RA, Bettcher K, Bums V, Lam K, Dossetor JB, Costerton JW. 1986. Effect of exit site infection and peritonitis on the distribution of biofilm encased adherent bacterial microcolonies (BABM) on Tenckhoff (T) catheters in patients undergoing continuous ambulatory peritoneal dialysis (CAPD). Adv Perit Dial. 4 : 102–109.
- Davies D. 2003. Understanding biofilm resistance to antibacterial agents. Nat Rev Drug Discov. 2 : 114–122.
- de Vin F, Rutherford P, Faict D. 2009. Intraperitoneal administration of drugs in peritoneal dialysis patients: a review of compatibility and guidance for clinical use. Perit Dial Int. 29 : 5–15.
- del Pozo JL, Patel R. 2007. The challenge of treating biofilm-associated bacterial infections. Clin Pharmacol Ther. 82 : 204–209.
- Dimitriadis A, Antoniou S, Toliou T, Papadopoulos C. 1990. Tissue reaction to deep cuff of Tenckhoff’s catheter and peritonitis. Adv Perit Dial. 6 : 155–158.
- Dombros, N, Dratwa, M, Feriani, M, Gokal, R, Heimburger, O, Krediet, R, Plum, J, Rodrigues, A, Selgas, R, Struijk, D et al. 2005. European best practice guidelines for peritoneal dialysis. 3 Peritoneal access. Nephrol Dial Transplant. 20:ix8–ix12.
- Eisenberg ES, Ambalu M, Szylagi G, Aning V, Soeiro R. 1987. Colonization of skin and development of peritonitis due to coagulase-negative staphylococci in patients undergoing peritoneal dialysis. J Infect Dis. 156 : 478–482.
- Eisenberg ES, Leviton I, Soeiro R. 1986. Fungal peritonitis in patients receiving peritoneal dialysis: experience with 11 patients and review of the literature. Rev Infect Dis. 8 : 309–321.
- Findon G, Miller T. 1995. Bacterial peritonitis in continuous ambulatory peritoneal dialysis: effect on dialysis on host defense mechanisms. Am J Kidney Dis. 26 : 765–773.
- Flessner MF, Li X, Potter R, He Z. 2010. Foreign-body response to sterile catheters is variable over 20 weeks. Adv Perit Dial. 26 : 101–104.
- Fraunholz M, Sinha B. 2012. Intracellular Staphylococcus aureus: live-in and let die. Front Cell Infect Microbiol. 2 : 43.
- Gagnon RF, Richards GK, Kostiner GB, Morcos R. 1993. Impact of dialysis fluid on the susceptibility of Staphylococcus epidermidis biofilms to rifampin. Adv Perit Dial. 9 : 183–186.
- Garcia LG, Lemaire S, Kahl BC, Becker K, Proctor RA, Denis O, Tulkens PM, Van Bambeke F. 2013. Antibiotic activity against small-colony variants of Staphylococcus aureus: review of in vitro, animal and clinical data. J Antimicrob Chemother. 68 : 1455–1464.
- Girard LP, Ceri H, Gibb AP, Olson M, Sepandj F. 2010. MIC versus MBEC to determine the antibiotic sensitivity of Staphylococcus aureus in peritoneal dialysis peritonitis. Perit Dial Int. 30 : 652–656.
- Glancey G, Cameron JS, Ogg C, Poston S. 1993. Adherence of Staphylococcus aureus to cultures of human peritoneal mesothelial cells. Nephrol Dial Transplant. 8 : 157–162.
- Gorman SP, Adair CG, Mawhinney WM. 1994. Incidence and nature of peritoneal catheter biofilm determined by electron and confocal laser scanning microscopy. Epidemiol Infect. 112 : 551–559.
- Gorman SP, Jones DS, Mawhinney WM, McGovern JG, Adair CG. 1997. Conditioning fluid influences on the surface properties of silicone and polyurethane peritoneal catheters: implications for infection. J Mater Sci: Mater Med. 8 : 631–635.
- Gorman SP, Mawhinney WM, Adair CG, Issouckis M. 1993. Confocal laser scanning microscopy of peritoneal catheter surfaces. J Med Microbiol. 38 : 411–417.
- Gristina AG. 1987. Biomaterial-centered infection: microbial adhesion versus tissue integration. Science. 237 : 1588–1595.
- Guerra e Silva A, Lima MGMS, Takiya CM, Andrade LR. 2011. Patient’s cells colonize the biofilm of Tenckhoff catheters used in peritoneal dialysis. Biofouling. 27 : 603–608.
- Guerra e Silva A, Takiya CM, Lima MG, Ribeiro C, Kaufman A, Pereira LC, Andrade LR. 2006. Early detection of Aspergillus terreus in a Tenckhoff catheter. Perit Dial Int. 26 : 723–724.
- Haagen IA, Heezius HC, Verkooyen RP, Verhoef J, Verbrugh HA. 1990. Adherence of peritonitis-causing staphylococci to human peritoneal mesothelial cell monolayers. J Infect Dis. 161 : 266–273.
- Hall-Stoodley L, Stoodley P. 2009. Evolving concepts in biofilm infections. Cell Microbiol. 11 : 1034–1043.
- Hall-Stoodley L, Stoodley P, Kathju S, Hoiby N, Moser C, Costerton JW, Moter A, Bjarnsholt T. 2012. Towards diagnostic guidelines for biofilm-associated infections. FEMS Immunol Med Microbiol. 65 : 127–145.
- Haslinger-Loffler B, Wagner B, Bruck M, Strangfeld K, Grundmeier M, Fischer U, Volker W, Peters G, Schulze-Osthoff K, Sinha B. 2006. Staphylococcus aureus induces caspase-independent cell death in human peritoneal mesothelial cells. Kidney Int. 70 : 1089–1098.
- Heilmann C. 2011. Adhesion mechanisms of staphylococci. Adv Exp Med Biol. 715 : 105–123.
- Hermsen ED, Hovde LB, Hotchkiss JR, Rotschafer JC. 2003. Increased killing of staphylococci and streptococci by daptomycin compared with cefazolin and vancomycin in an in vitro peritoneal dialysate model. Antimicrob Agents Chemother. 47 : 3764–3767.
- Horsman GB, MacMillan L, Amatnieks Y, RifKin O, Vas SI. 1986. Plasmid profile and slime analysis of coagulase-negative Staphylococci from CAPD patients with peritonitis. Perit Dial Int. 6 : 195–198.
- Hotchkiss JR, Hermsen ED, Hovde LB, Simonson DA, Rotschafer JC, Crooke PS. 2004. Dynamic analysis of peritoneal dialysis associated peritonitis. ASAIO J. 50 : 568–576.
- Huang JW, Chu TS, Wu MS, Peng YS, Hsieh BS. 2000. Visible Penicillium spp. colonization plaques on a Tenckhoff catheter without resultant peritonitis in a peritoneal dialysis patient. Nephrol Dial Transplant. 15 : 1872–1873.
- Jacobs RF, Wilson CB. 1983. Intracellular penetration and antimicrobial activity of antibiotics. J Antimicrob Chemother. 12 : 13–20.
- Kalan L, Wright GD. 2011. Antibiotic adjuvants: multicomponent anti-infective strategies. Expert Rev Mol Med. 13 : e5.
- Keane WF, Bailie GR, Boeschoten E, Gokal R, Golper TA, Holmes CJ, Kawaguchi Y, Piraino B, Riella M, Vas S. 2000. Adult peritoneal dialysis-related peritonitis treatment recommendations: 2000 update. Perit Dial Int. 20 : 396–411.
- Kreft B, Ilic S, Ziebuhr W, Kahl A, Frei U, Sack K, Trautmann M. 1998. Adherence of Staphylococcus aureus isolated in peritoneal dialysis-related exit-site infections to HEp-2 cells and silicone peritoneal catheter materials. Nephrol Dial Transplant. 13 : 3160–3164.
- Krismer B, Peschel A. 2011. Does Staphylococcus aureus nasal colonization involve biofilm formation? Future Microbiol. 6 : 489–493.
- Kristinsson KG, Spencer RC, Brown CB. 1986. Clinical importance of production of slime by coagulase negative staphylococci in chronic ambulatory peritoneal dialysis. J Clin Pathol. 39 : 117–118.
- Kumar A, Pulicherla KK, Ram KS, Rao KRSS. 2010. Evolutionary trend of thrombolytics. Int J Bio Sci Bio Technol. 2 : 51–68.
- Kutner NG, Zhang R, Barnhart H, Collins AJ. 2005. Health status and quality of life reported by incident patients after 1 year on haemodialysis or peritoneal dialysis. Nephrol Dial Transplant. 20 : 2159–2167.
- Li PK, Chow KM. 2012. Infectious complications in dialysis-epidemiology and outcomes. Nat Rev Nephrol. 8 : 77–88.
- Li PK, Szeto CC, Piraino B, Bernardini J, Figueiredo AE, Gupta A, Johnson DW, Kuijper EJ, Lye WC, Salzer W, et al. 2010. Peritoneal dialysis-related infections recommendations: 2010 update. Perit Dial Int. 30 : 393–423.
- Locci R, Romagnoni M, Beccari M, Faiolo S, Granello E, Lischetti MG, Quaroni S, Scalia P. 1984. Massive colonization of an in-dwelling catheter by Penicillium pinophilum without peritonitis. Perit Dial Int. 4 : 243–244.
- Ludlam H, Johnston L, Hopkins P. 1992. Susceptibility testing of bacteria recovered from patients with peritonitis complicating continuous ambulatory peritoneal dialysis. Antimicrob Agents Chemother. 36 : 1097–1101.
- Ludlam HA, Price TN, Berry AJ, Phillips I. 1988. Laboratory diagnosis of peritonitis in patients on continuous ambulatory peritoneal dialysis. J Clin Microbiol. 26 : 1757–1762.
- MacDonald WA, Watts J, Bowmer MI. 1986. Factors affecting Staphylococcus epidermidis growth in peritoneal dialysis solutions. J Clin Microbiol. 24 : 104–107.
- Marrie TJ, Noble MA, Costerton JW. 1983. Examination of the morphology of bacteria adhering to peritoneal dialysis catheters by scanning and transmission electron microscopy. J Clin Microbiol. 18 : 1388–1398.
- McDermid KP, Morck DW, Olson ME, Boyd ND, Khoury AE, Dasgupta MK, Costerton JW. 1993. A porcine model of Staphylococcus epidermidis catheter-associated infection. J Infect Dis. 168 : 897–903.
- McNeely DJ, Vas SI, Dombros N, Oreopoulos DG. 1981. Fusarium peritonitis: an uncommon complication of continuos ambulatory peritoneal dialysis. Perit Dial Int. 1 : 94–96.
- Muthukrishnan G, Quinn GA, Lamers RP, Diaz C, Cole AL, Chen S, Cole AM. 2011. Exoproteome of Staphylococcus aureus reveals putative determinants of nasal carriage. J Proteome Res. 10 : 2064–2078.
- Nodaira Y, Ikeda N, Kobayashi K, Watanabe Y, Inoue T, Gen S, Kanno Y, Nakamoto H, Suzuki H. 2008. Risk factors and cause of removal of peritoneal dialysis catheter in patients on continuous ambulatory peritoneal dialysis. Adv Perit Dial. 24 : 65–68.
- Ortiz A, Gallegos G, Esteban J, Gadea I, Reyero A, Fortes JR, Caramelo C. 1999. Peritoneal catheter colonization by Penicillium sp.: case report and review of Penicillium-related complications in peritoneal dialysis. Am J Kidney Dis. 33 : E5.
- Perl J, Wald R, Bargman JM, Na Y, Jassal SV, Jain AK, Moist L, Nessim SJ. 2012. Changes in patient and technique survival over time among incident peritoneal dialysis patients in Canada. Clin J Am Soc Nephrol. 7 : 1145–1154.
- Peters T, Potter R, Li X, He Z, Hoskins G, Flessner MF. 2011. Mouse model of foreign body reaction that alters the submesothelium and transperitoneal transport. Am J Physiol Renal Physiol. 300 : F283–F289.
- Pihl M, Davies JR, Johansson AC, Svensater G. 2013. Bacteria on catheters in patients undergoing peritoneal dialysis. Perit Dial Int. 33 : 51–59.
- Piraino B, Bailie GR, Bernardini J, Boeschoten E, Gupta A, Holmes C, Kuijper EJ, Li PK, Lye WC, Mujais S, et al. 2005. Peritoneal dialysis-related infections recommendations: 2005 update. Perit Dial Int. 25 : 107–131.
- Piraino B, Bernardini J, Brown E, Figueiredo A, Johnson DW, Lye WC, Price V, Ramalakshmi S, Szeto CC. 2011. ISPD position statement on reducing the risks of peritoneal dialysis-related infections. Perit Dial Int. 31 : 614–630.
- Read RR, Eberwein P, Dasgupta MK, Grant SK, Lam K, Nickel JC, Costerton JW. 1989. Peritonitis in peritoneal dialysis: bacterial colonization by biofilm spread along the catheter surface. Kidney Int. 35 : 614–621.
- Reid G, Schwarz-Faulkner S, McGroarty JA, Bruce AW. 1990. Bacterial aggregation in relation to peritoneal dialysis. Perit Dial Int. 10 : 21–24.
- Richards GK, Kostiner GB, Gagnon RF. 1992. The modulation by fresh and spent peritoneal dialysis fluids of antibiotic kinetics directed against Staphylococcus epidermidis biofilms. Adv Perit Dial. 8 : 246–249.
- Rocha A, Rodrigues A, Teixeira L, Carvalho MJ, Mendonça D, Cabrita A. 2012. Temporal trends in peritonitis rates, microbiology and outcomes: the major clinical complication of peritoneal dialysis. Blood Purif. 33 : 284–291.
- Rodrigues AS, Matos CB, Silva F, Fonseca I, Nogueira C, Santos J, Silva AS, Cabrita A. 2006. Long-term peritoneal dialysis experience in Portugal. Int J Artif Organs. 29 : 1109–1116.
- Rodriguez-Carmona A, Perez FM, Garcia FT, Valdes CF. 2000. Prevalence of microbial colonization in removed peritoneal catheters: a prospective study. Adv Perit Dial. 16 : 276–279.
- Salzer W. 2005. Antimicrobial-resistant gram-positive bacteria in PD peritonitis and the newer antibiotics used to treat them. Perit Dial Int. 25 : 313–319.
- Sekkarie M, Holmes C, Ranjit U, Kauffman CA. 1991. Grossly visible fungal colonization of a Tenckhoff catheter: a case report and literature review. Perit Dial Int. 11 : 85–87.
- Sendi P, Proctor RA. 2009. Staphylococcus aureus as an intracellular pathogen: the role of small colony variants. Trends Microbiol. 17 : 54–58.
- Seneviratne CJ, Jin L, Samaranayake LP. 2008. Biofilm lifestyle of Candida: a mini review. Oral Dis. 14 : 582–590.
- Sepandj F, Ceri H, Gibb AP, Read RR, Olson M. 2003. Biofilm infections in peritoneal dialysis-related peritonitis: comparison of standard MIC and MBEC in evaluation of antibiotic sensitivity of coagulase-negative staphylococci. Perit Dial Int. 23 : 77–79.
- Sepandj F, Ceri H, Gibb A, Read R, Olson M. 2004. Minimum inhibitory concentration (MIC) versus minimum biofilm eliminating concentration (MBEC) in evaluation of antibiotic sensitivity of Gram-negative bacilli causing peritonitis. Perit Dial Int. 24 : 65–67.
- Shalit I, Welch DF, San Joaquin VH, Marks MI. 1985. In vitro antibacterial activities of antibiotics against Pseudomonas aeruginosa in peritoneal dialysis fluid. Antimicrob Agents Chemother. 27 : 908–911.
- Silva MT. 2012. Classical labeling of bacterial pathogens according to their lifestyle in the host: inconsistencies and alternatives. Front Microbiol. 3 : 71.
- Silverstein DM, Wilcox JE. 2010. Outcome of accidental peritoneal dialysis catheter holes or tip exposure. Pediatr Nephrol. 25 : 1147–1151.
- Spencer RC. 1988. Infections in continuous ambulatory peritoneal dialysis. J Med Microbiol. 27 : 1–9.
- Swartz R, Messana J, Holmes C, Williams J. 1991. Biofilm formation on peritoneal catheters does not require the presence of infection. ASAIO Trans. 37 : 626–634.
- Szeto CC, Kwan BC, Chow KM, Lau MF, Law MC, Chung KY, Leung CB, Li PK. 2008. Coagulase negative staphylococcal peritonitis in peritoneal dialysis patients: review of 232 consecutive cases. Clin J Am Soc Nephrol. 3 : 91–97.
- Szeto CC, Kwan BC, Chow KM, Law MC, Pang WF, Leung CB, Li PK. 2011. Repeat peritonitis in peritoneal dialysis: retrospective review of 181 consecutive cases. Clin J Am Soc Nephrol. 6 : 827–833.
- Taylor PC. 1994. Routine laboratory diagnosis of continuous ambulatory peritoneal dialysis peritonitis using centrifugation/lysis and saponin-containing media. Eur J Clin Microbiol Infect Dis. 13 : 249–252.
- Teitelbaum I, Burkart J. 2003. Peritoneal dialysis. Am J Kidney Dis. 42 : 1082–1096.
- Thwaites GE, Gant V. 2011. Are bloodstream leukocytes Trojan horses for the metastasis of Staphylococcus aureus? Nat Rev Microbiol. 9 : 215–222.
- Tobudic S, Kratzer C, Poeppl W, Vychytil A, Burgmann H. 2011. Impact of various peritoneal dialysis solutions on the growth of common bacterial and yeast pathogens. Perit Dial Int. 31 : 688–692.
- Tobudic S, Poeppl W, Kratzer C, Vychytil A, Burgmann H. 2012. Comparative in vitro antimicrobial activity of vancomycin, teicoplanin, daptomycin and ceftobiprole in four different peritoneal dialysis fluids. Eur J Clin Microbiol Infect Dis. 31 : 1327–1334.
- Torella JP, Chait R, Kishony R. 2010. Optimal drug synergy in antimicrobial treatments. PLoS Comput Biol. 6 : e1000796.
- Tuchscherr L, Medina E, Hussain M, Volker W, Heitmann V, Niemann S, Holzinger D, Roth J, Proctor RA, Becker K, et al. 2011. Staphylococcus aureus phenotype switching: an effective bacterial strategy to escape host immune response and establish a chronic infection. EMBO Mol Med. 3 : 129–141.
- Vas S, Oreopoulos DG. 2001. Infections in patients undergoing peritoneal dialysis. Infect Dis Clin North Am. 15 : 743–774.
- Verger C, Chesneau AM, Thibault M, Bataille N. 1987. Biofilm on Tenckhoff catheters: a possible source for peritonitis. Perit Dial Int. 7 : 174–178.
- von Graevenitz A, Amsterdam D. 1992. Microbiological aspects of peritonitis associated with continuous ambulatory peritoneal dialysis. Clin Microbiol Rev. 5 : 36–48.
- Ward KH, Olson ME, Lam K, Costerton JW. 1992. Mechanism of persistent infection associated with peritoneal implants. J Med Microbiol. 36 : 406–413.
- Wegmann F, Heilesen AM, Horn T. 1988. Tenckhoff catheter penetrated by Aspergillus fumigatus: a case report. Perit Dial Int. 8 : 281–284.
- Weidenmaier C, Goerke C, Wolz C. 2012. Staphylococcus aureus determinants for nasal colonization. Trends Microbiol. 20 : 243–250.
- Wilcox MH, Geary I, Spencer RC. 1992. In vitro activity of imipenem, in comparison with cefuroxime and ciprofloxacin, against coagulase-negative staphylococci in broth and peritoneal dialysis fluid. J Antimicrob Chemother. 29 : 49–55.
- Wilcox MH, Smith DG, Evans JA, Denyer SP, Finch RG, Williams P. 1990. Influence of carbon dioxide on growth and antibiotic susceptibility of coagulase-negative staphylococci cultured in human peritoneal dialysate. J Clin Microbiol. 28 : 2183–2186.
- Yanagisawa N, Li DQ, Ljungh A. 2004. Protein adsorption on ex vivo catheters and polymers exposed to peritoneal dialysis effluent. Perit Dial Int. 24 : 264–273.
- Zelenitsky S, Franczuk C, Fine A, Ariano R, Harding G. 2002. Antibiotic tolerance of peritoneal bacterial isolates in dialysis fluids. J Antimicrob Chemother. 49 : 863–866.
- Zhao X, Drlica K. 2001. Restricting the selection of antibiotic-resistant mutants: a general strategy derived from fluoroquinolone studies. Clin Infect Dis. 33 : S147–S156.