Abstract
Purpose: Topical hemostatic agents are an important means of controlling or preventing bleeding. This study was performed to compare gelatin–thrombin matrix with smooth particles (SmGM) versus gelatin–thrombin matrix with stellate particles (StGM) in a porcine kidney bleeding model. Materials and methods: In male pigs, reproducible lesions (diameter and depth ∼10 mm) were created in the renal cortex. Each lesion was treated topically using either SmGM or StGM. Blood loss was quantified before and 2, 5 and 10 minutes after treatment. Dry mass, ultrastructural and histologic analyses were also performed. Results: Thirty-two lesions were treated with SmGM and 32 with StGM; median initial bleeding rates were 27.6 and 29.1 mL/min, respectively. Two minutes post-application, SmGM was associated with significantly less bleeding than StGM (0.574 vs 0.920 mL/min; p < .0001). This difference stemmed principally from lesions with initial blood loss >29 mL/min, where bleeding rates at 2 minutes were ∼3-fold higher with StGM (1.636 vs 0.567 mL/min; p ≥ 0.040). Dry mass per unit volume of hemostatic agent was significantly higher with SmGM versus StGM. SmGM formed discrete, smooth particles, while StGM particles were stellate and tended to coalesce. Histologic analysis showed more solid mass, larger particles and less intervening space with SmGM versus StGM. Conclusions: In a severe, high-volume bleeding model, residual bleeding at 2 minutes was significantly lower with SmGM versus StGM, and SmGM showed greater consistency across bleeding intensities. These findings may be attributable to dry mass per unit volume and/or ultrastructural differences between the two agents.
INTRODUCTION
Intraoperative bleeding is associated with an increased risk of morbidity and mortality across all surgical areas. Patients with major bleeding often require transfusions of allogeneic blood products, which are associated with a variety of safety concerns such as transmission of pathogens and allergic/immunogenic reactions.Citation1 Related financial considerations include the costs of blood components, burdens associated with managing complications, and the fact that hospitalization times are longer in patients who receive transfusions.Citation2,Citation3 Overall, there is a strong rationale for minimizing intraoperative and perioperative bleeding.
Topical hemostatic agents are an important means of controlling or preventing bleeding where conventional methods are inefficient or impractical. A variety of these agents are available, and there are several ways of classifying them. One approach is based on three different categories: active, non-active, and agents with combinations of both active and non-active characteristics.Citation4 Active hemostatic agents contain thrombin, and are therefore suitable for use in patients with coagulopathy other than hypofibrinogenemia. Non-active hemostatic agents function via mechanical or synthetic components; they do not contain any clotting factors. Agents with both non-active and active characteristics include thrombin plus mechanical hemostatic agents (sometimes described as ‘flowable hemostatic agents’) and fibrin sealants.
Flowable hemostatic agents are typically combinations of extracellular matrix materials (e.g. gelatin, collagen) and thrombin. These components serve as a barrier against blood flow, an activator of platelets and a trigger for the conversion of fibrinogen to fibrin at the site of application. Gelatin and thrombin are the most commonly used topical hemostatic agents,Citation5 and gelatin–thrombin matrices have been proven effective as adjuncts to hemostasis.Citation6–9
Gelatin–thrombin matrix with smooth particles (SmGM) is provided as a kit with bovine gelatin matrix and thrombin derived from human plasma. Gelatin–thrombin matrix with stellate particles (StGM) is similarly provided as a kit with gelatin and thrombin; unlike SmGM, the gelatin matrix is porcine-derived, while the thrombin is human in both agents. Both hemostatic agents can be prepared quickly (2–3 min) ensuring minimum delay when bleeding needs to be controlled.
Results from a porcine spleen model of bleeding indicated that there were no significant differences between SmGM and StGM in the time taken for hemostasis to be achieved.Citation10 However, other studies have reported differences between the two agents. In vitro data have shown increased ability of SmGM to retain thrombin and red blood cells and, in a porcine liver abrasion model, SmGM provided superior control of bleeding versus StGM.Citation11 Results from another porcine liver study (a trauma model) showed that SmGM induced clotting in 3/3 wounds versus 1/3 with StGM; it was suggested that coagulation was initiated immediately with SmGM but not with StGM.Citation12
Based on the results above, there is some uncertainty as to whether there are any clinically relevant differences between SmGM and StGM. We sought to provide further evidence by comparing the hemostatic efficiency of these agents in a challenging porcine kidney bleeding model. Dry mass and ultrastructure analyses of the matrices were also performed, to investigate whether these aspects may affect hemostatic performance.
MATERIALS AND METHODS
In Vivo Testing
The in vivo part of the study was performed in male pigs (n = 4), each weighing approximately 35 kg. An animal experiment permit was issued by the municipal government of Vienna, and all experimental methods were consistent with the Guide for the Care and Use of Laboratory Animals of the US National Research Council.Citation13
Before surgery, each animal was anesthetized with tiletamine/zolazepam (Zoletil; Virbac, Bury St Edmunds, Suffolk, UK; ∼7 mg/kg bodyweight). The pigs were then intubated and further inhalation anesthesia (isoflurane and oxygen) was administered. Intraoperatively the animals received acetate-buffered crystalloid solution (Elomel; Fresenius Kabi, Graz, Austria; 10 mL/kg/h), sufentanil 0.008 mg/kg/h and rocuronium bromide 5 mg/kg/h. A mean arterial blood pressure between 80 and 110 mmHg was maintained using L-noradrenaline and hydroxyethyl starch (Voluven; Fresenius Kabi, Graz, Austria). At the end of the experiment, animals were euthanized under deep anesthesia with a lethal dose of thiopental sodium and embutramide/mebezonium iodine/tetracaine hydrochloride (T61; Hoechst Marion Roussel, Brussels, Belgium).
The animals were placed in dorsal recumbency, and biopsy punches with 10 mm diameter were used to create reproducible lesions (approximately 10 mm deep) in the renal cortex (). A total of eight lesions were created in each kidney. Each lesion was treated topically using either SmGM (FloSeal 5 mL kit; Baxter Healthcare, Deerfield, Illinois, United States) or StGM (Surgiflo; Ethicon, Somerville, New Jersey, United States), with sufficient material to fill the cavity. An alternating pattern was used (the first lesion was treated with SmGM, the next with StGM, and so on) to ensure that the two agents were applied to lesions with similar characteristics. No systemic heparinization was applied.
FIGURE 1 Examples of lesions in the porcine renal cortex, 10 mm in diameter and depth (created using a biopsy punch). Image taken after application of the hemostatic agents. A: lesions treated with gelatin–thrombin matrix with smooth particles (SmGM); B: lesions treated with gelatin–thrombin matrix with stellate particles (StGM).
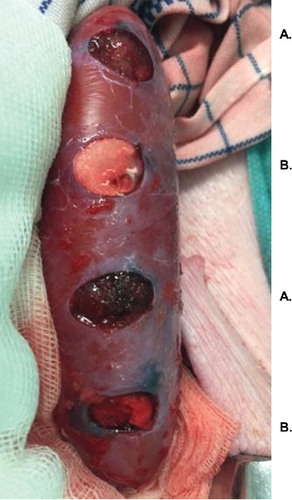
SmGM (lot number VNY2R009-AA, expiry date October 2017) and StGM (lot number 248994, expiry date March 2018) were reconstituted and applied according to the instructions for use. After tissue lesions had been filled up using the hemostatic agent, a wet gauze was manually applied for 2 minutes. Pulsatile bleeding occurring after application of hemostatic agent was considered to represent application failure. After 5 minutes, sodium chloride solution was used to irrigate excess material from the lesion.
Blood loss was quantified before application of hemostatic agent (pre-weighed dry gauze applied to the lesion for 3 seconds) and 2, 5 and 10 minutes post-application (dry gauze applied for 30 seconds). The amount of blood taken up by the gauze during application to the lesion was assessed by weight.
Blood loss data were initially analyzed for all lesions. Subsequently, to characterize the impact of bleeding intensity (before treatment) on hemostatic performance, a bleeding rate threshold of 29 mL/min was introduced. Bleeding rates were then analyzed for lesions initially bleeding more than or less than the threshold. The 29 mL/min threshold was chosen because this was the median initial bleeding rate for lesions treated with StGM.
Initial bleeding severity was also assessed by the surgeon using an established Validated Intraoperative Bleeding Scale (VIBe SCALE): Grade 0, no bleeding (visually estimated rate of blood loss: ≤ 1.0 mL/min); Grade 1, ooze or intermittent flow (>1.0–5.0 mL/min); Grade 2, continuous flow (>5.0–10.0 mL/min); Grade 3, controllable spurting and/or overwhelming flow (>10.0–50.0 mL/min); Grade 4, unidentified or inaccessible spurting or gush (>50.0 mL/min).Citation14
Mean arterial blood pressure may vary during surgery, potentially affecting bleeding rates. In order to ascertain consistency, blood pressure was assessed for each lesion before application of the hemostatic agent. To enable these assessments, the carotid artery was cannulated after surgical preparation and the mean arterial blood pressure was monitored via a clinical monitor (Dräger Infinity Delta, Dräger Austria, Austria).
Weight Measurements
Weight analyses were performed using freshly reconstituted SmGM and StGM (three samples of each). Wet mass per mL was determined by dividing the mass of freshly reconstituted material by total volume. Dry mass was determined as the mass of the entire hemostatic matrix reconstituted with thrombin solution after drying at 120°C for 2 hours. The percentage of solid content of each agent was determined by the ratio of dry mass to the volume of the freshly reconstituted (wet) material.
Matrix Ultrastructure
Both hemostatic agents were prepared according to the manufacturer's instructions. After reconstitution of the gelatin component with thrombin solution and thorough mixing (“swooshing”) of the formulation, both hemostatic agents were evenly distributed over a 10 cm polystyrene cell culture dish (Starlab, Hamburg, Germany) and thinned out with a spatula. Morphological analysis was performed using a Keyence VHX-1000 digital light microscope (Keyence International, Mechelen, Belgium) in high dynamic range (16 bit) mode, immediately after reconstitution.
Histology
Cross-sections of lesions after application of hemostatic agent were analyzed histologically. A single, representative sample for each hemostatic agent was stained with Martius, Scarlet and Blue, fixed in formalin for 24 hours and immersed in 50% alcohol for 1 hour, before storage in 70% alcohol. Microscopic assessment was subsequently performed.
Statistics
Statistical analysis was performed with GraphPad Prism (version 7). The primary study endpoint was blood loss per minute (2, 5 and 10 minutes after application of the hemostatic agent). Data are presented as median values with interquartile ranges. The D'Agostino Pearson test was used to determine whether data were distributed normally. All groups except one were not normally distributed, and data were analyzed using the Mann-Whitney test, with Welch's correction in case of non-comparable standard deviations. Blood loss was initially measured in grams; the density of blood was approximated to 1 g/mL to facilitate presentation of the data with milliliters per minute (mL/min) as the unit.
RESULTS
In Vivo
Observer-Assessed Bleeding (Before Application of Hemostatic Agent)
According to VIBe SCALE assessments, initial bleeding was the same for lesions subsequently treated with either SmGM or StGM. Twenty-seven out of 32 SmGM-treated lesions were considered to be Grade 3, and 27/32 StGM-treated lesions were also Grade 3. All remaining lesions were Grade 2.
Blood Loss Measurements
Initial bleeding rates (median [interquartile range]) were 27.6 (23.1–31.8) and 29.1 (24.1–36.4) mL/min in lesions treated with SmGM and StGM, respectively. Mean arterial blood pressures, measured before application of hemostatic agent, were 88.9 mmHg (standard deviation [SD]: 9.70 mmHg) and 89.7 mmHg (SD: 7.49 mmHg). Sustained, pulsatile bleeding was observed at the 2-minute time-point following two applications of StGM and one application of SmGM. These applications were considered to be failures and were therefore removed from the study.
At the 2-minute time-point, lesions treated with SmGM showed significantly less bleeding than those treated with StGM (p < .0001; ). At 5 and 10 minutes, blood loss remained lower with SmGM, but the differences between the two hemostatic agents were no longer statistically significant. In general, StGM showed a higher variability in blood loss particularly at the 2-minute time-point. With SmGM, one outlier was detected at 2 minutes and this was not rated as a failure because of lack of pulsatile blood loss. Bleeding from this lesion had stopped by the 5-minute time-point.
FIGURE 2 Comparison of blood loss in a porcine kidney standardized puncture model: 2, 5 and 10 minutes after application of gelatin–thrombin matrix with smooth particles (SmGM) or gelatin–thrombin matrix with stellate particles (StGM). Horizontal lines and error bars represent median values and interquartile ranges, respectively. One outlying data point (SmGM, 2 minutes post-application; bleeding rate of 7.0 mL/min) is not shown. *p < .0001 (SmGM versus StGM).
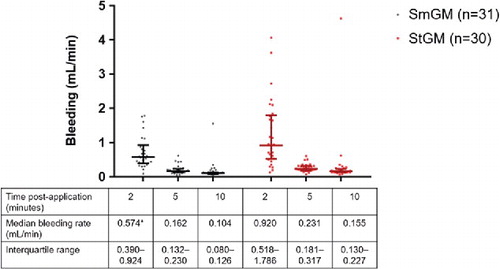
Blood Loss Results with Stratification by Initial Bleeding Intensity
In lesions with initial blood loss <29 mL/min, differences between the two agents in the rate of blood loss were minor at all time-points, although statistically significant reductions with SmGM versus StGM were evident at the 5- and 10-minute time-points (). In lesions with high initial bleeding (≥29 mL/min), SmGM performed significantly better than StGM at the 2-minute time-point (0.567 vs 1.636 mL/min; p = 0.040; ). No significant difference was seen at later time-points.
FIGURE 3 Comparison of blood loss in a porcine kidney standardized puncture model: lesions with initial blood rates < 29 mL/min (2, 5 and 10 minutes after application of gelatin–thrombin matrix with smooth particles [SmGM] or gelatin–thrombin matrix with stellate particles [StGM]). Horizontal lines and error bars represent median values and interquartile ranges, respectively. One outlying data point (SmGM, 2 minutes post-application; bleeding rate of 7.0 mL/min) is not shown. *p < .01; **p < .0001 (SmGM versus StGM).
![FIGURE 3 Comparison of blood loss in a porcine kidney standardized puncture model: lesions with initial blood rates < 29 mL/min (2, 5 and 10 minutes after application of gelatin–thrombin matrix with smooth particles [SmGM] or gelatin–thrombin matrix with stellate particles [StGM]). Horizontal lines and error bars represent median values and interquartile ranges, respectively. One outlying data point (SmGM, 2 minutes post-application; bleeding rate of 7.0 mL/min) is not shown. *p < .01; **p < .0001 (SmGM versus StGM).](/cms/asset/525e50cc-bb47-4153-bec8-bc59f3fa45c9/iivs_a_1447619_f0003_c.jpg)
FIGURE 4 Comparison of blood loss in a porcine kidney standardized puncture mode: lesions with initial blood rates ≥29 mL/min (2, 5 and 10 minutes after application of gelatin–thrombin matrix with smooth particles [SmGM] or gelatin–thrombin matrix with stellate particles [StGM]). Horizontal lines and error bars represent median values and interquartile ranges, respectively. *p = 0.040 (SmGM versus StGM).
![FIGURE 4 Comparison of blood loss in a porcine kidney standardized puncture mode: lesions with initial blood rates ≥29 mL/min (2, 5 and 10 minutes after application of gelatin–thrombin matrix with smooth particles [SmGM] or gelatin–thrombin matrix with stellate particles [StGM]). Horizontal lines and error bars represent median values and interquartile ranges, respectively. *p = 0.040 (SmGM versus StGM).](/cms/asset/b53bfffc-f195-4c45-8c23-7484b6a746ad/iivs_a_1447619_f0004_c.jpg)
With SmGM, only small differences in median rates of post-application blood loss were observed between lesions initially bleeding above or below the 29 mL/min threshold. For example, at 2 minutes, median values were 0.567 vs. 0.561 mL/min, respectively. In contrast, blood loss with StGM at the 2-minute time-point was considerably higher in lesions initially bleeding above versus below the 29 mL/min threshold (median values 1.636 vs. 0.528 mL/min).
Initial bleeding intensity was missing for three lesions (one treated with SmGM and two with StGM), and these three were omitted from the results with stratification.
Additional Observations
Special care was required with StGM as material tended to stick to gauze upon removal. In general, StGM exhibited a more foamy and soft texture than SmGM at the 2-minute time-point.
Weight Measurements
Dry mass per unit volume of hemostatic agent was significantly higher with SmGM compared to StGM (). This finding is related to the fact that the reconstituted volume of SmGM is 5 mL, whereas that of StGM is 8 mL. Accordingly, wet mass per unit volume of SmGM was higher than with StGM.
TABLE 1 Weight measurement analysis of gelatin–thrombin matrix with smooth particles (SmGM) and gelatin–thrombin matrix with stellate particles (StGM).
Matrix Ultrastructure
SmGM formed discrete particles with a smooth profile, while StGM particles coalesced with a stellate appearance (). Structural differences between the two agents were evident at different magnifications.
Histology
Histologic analysis showed both hemostatic agents adapted well to the wound bed, completely filling the cavities. Compared with StGM, SmGM exhibited more solid mass, larger particles and less intervening space (). Therefore, an increased amount of blood was able to infiltrate StGM. Blood penetration decreased towards the top of the lesion with SmGM because coagulation had begun to occur.
FIGURE 6 Histology of the two hemostatic agents. Images from light microscopy, with Martius-Scarlet-Blue staining. Yellow areas represent fresh fibrin, erythrocytes are stained yellow, and connective tissue is shown in blue. A and C: gelatin–thrombin matrix with smooth particles (SmGM); B and D: gelatin–thrombin matrix with stellate particles (StGM).
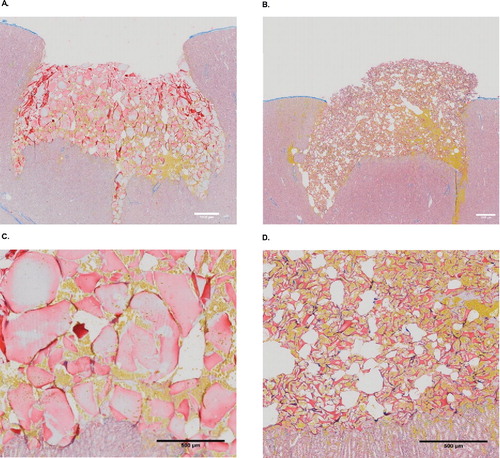
DISCUSSION
The aim of this study was to compare the hemostatic efficacy of SmGM and StGM in a porcine model of bleeding. To represent severe high-volume bleeding, a kidney punch model was chosen, using 10 mm deep punch lesions with a diameter of 10 mm in the cortex of the kidney. Most of the lesions had Grade 3 bleeding severity according to the VIBe SCALE. Residual bleeding after treatment with SmGM was lower than that following with StGM, particularly at the 2-minute time-point. For surgeons, arresting bleeding as early as possible is relevant as is reducing the total amount of bleeding during the intervention.
The difference between SmGM and StGM in hemostatic efficacy at the 2-minute time-point was driven principally by lesions with high pre-treatment bleeding rates (≥29 mL/min). This suggests that the hemostatic differences reported here between SmGM and StGM are likely to be most relevant in surgical settings where high rates of bleeding are expected (e.g. cardiac, orthopedic and hepatic procedures). A study of data from > 600 hospitals across the USA reported that surgical subgroups with the highest percentages of patients developing bleeding-related complications and/or receiving a blood product transfusion were cardiac (47.4%), non-cardiac thoracic (34.3%), vascular (31.5%) and knee/hip replacement (29.8%).Citation15 Similarly, in a French study, surgical groups with highest percentages of patients with bleeding-related consequences were transplantations (20.6%), vascular replacement (19.2%), revision and multiple orthopedic surgery (18.8%) and coronary bypass (15.2%).Citation16 Based on the results presented here, SmGM is most likely to be recommended in preference to StGM in these settings.
Our data indicate that SmGM performs more reliably across a range of bleeding rates than StGM. For surgeons, this could be considered as a major benefit because when the decision to use a hemostatic agent is made, the full intensity of bleeding may not be apparent.
Lack of consistency with StGM in the treatment of bleeding may have economic consequences. Multiple health economic and outcome research investigations have reported advantages with SmGM. A European analysis comparing SmGM and StGM in spine surgery reported reduced operating room time and reduced volume with SmGM, resulting in overall cost savings compared with StGM.Citation17 Similarly, US studies of cardiac surgery and spine surgery have shown that, compared with StGM, SmGM can reduce rates of blood product transfusions as well as operating time, again resulting in cost savings.Citation18,Citation19
These two hemostatic agents have previously been compared in humans. One study of patients undergoing partial nephrectomy showed no significant differences between SmGM and StGM in ischemia time or blood loss.Citation20 The results of a comparison in patients undergoing cranial and spinal surgery suggested that SmGM and StGM are hemostatically equivalent.Citation21 A third study, performed in thoracic and lumbar spine surgery, reported very similar hemostasis times with both agents.Citation22 It is possible that pre-treatment bleeding intensities in these studies were too low, or that the patient populations were not sufficiently homogeneous, for hemostatic differences between the two agents to be apparent.
The dry mass per unit volume of hemostatic agent was significantly higher with SmGM. This difference, which is related to greater air content in reconstituted StGM, was probably a contributor to the hemostatic differences between the two agents. Comparing the total mass per unit volume of both hemostatic agents (), the percentage of air in reconstituted StGM was estimated to be approximately 38% (∼3 mL of air per 8 mL product). Application of comparable volumes (rather than comparable masses) of each hemostatic agent is therefore expected to give different matrix densities post-application. This was borne out in the histologic analysis, with StGM exhibiting a more porous structure (). The concentration of gelatin has previously been hypothesized to have an impact on hemostatic efficacy of gelatin–thrombin matrix.Citation10 In addition, our findings show that ultrastructural differences after reconstitution are maintained after application in vivo, resulting in different extents of blood penetration and differing rates of coagulation. Our microscopic observations reflect the findings of previous studies, which also reported increased hemostatic efficacy with SmGM versus StGM.Citation11,Citation12 The authors of these studies suggested that the difference in hemostatic efficacy may have been related to increased retention of thrombin with the smoother structure of SmGM particles. It is possible that thrombin retention becomes more important with increased bleeding intensity; this might explain why the difference between the two hemostatic agents was driven by lesions with initial bleeding above 29 mL/min. The microscopic structures of the two hemostatic agents could also have contributed to the tendency of StGM but not SmGM to adhere to the gauze, resulting in a need for increased care when removing the gauze from StGM-treated lesions. The structural differences between SmGM and StGM are likely to stem from the production processes of the two agents.
This study was performed in standardized lesions in one organ to ensure high consistency and high sensitivity for measuring differences between the two hemostatic agents. These conditions would not be achievable in clinical settings. A further strength of the study was the accurate assessment of bleeding, which can be difficult to achieve in clinical trials. A potential limitation of this pre-clinical study is that it does not account for factors resulting from inter-individual differences co-morbidities or use of anticoagulant medications as found in clinical patients. To cover such aspects a clinical study would be advisable. This is especially relevant in cardiac surgical procedures where heparinization is commonly performed, which may lead to additional bleeding-related complications.
In conclusion, SmGM showed increased hemostatic efficacy compared with StGM in a severe, high-volume bleeding model where VIBe SCALE bleeding severity was mainly Grade 3. This difference was amplified in lesions with high pre-treatment bleeding intensity (≥29 mL/min), where the bleeding rate with StGM was almost three times that with SmGM at the 2-minute time-point. SmGM performed more consistently than StGM across different bleeding intensities. These findings may be related to differences between the two agents in dry mass per unit volume and/or particulate ultrastructure. It could be hypothesized from the present work that the efficacy differences between SmGM and StGM have a clinical impact on patients and an economic impact of relevance to healthcare providers, but further clinical research is necessary to obtain a final conclusion in this aspect.
DECLARATION OF INTEREST
The authors report conflicts of interest. HR and PS are CEOs of Trauma Care Consult GmbH, the organization that received the funding to conduct the study. DS and HG are full time employees of Baxter Medical Products GmbH. All other authors have no conflicts of interest to declare. The studies reported here were designed and performed using established scientific methods with impartial data collection and analysis. The authors are responsible for the content of the article. Meridian HealthComms Ltd provided writing assistance, which was paid for by Baxter Healthcare Corporation.
ACKNOWLEDGMENTS
We would like to thank Kevin M. Lewis DVM for reviewing the manuscript, Dr Sylvia Nürnberger for her support with histology, and Jernej Hren DVM for surgical support.
Additional information
Funding
REFERENCES
- Vamvakas EC, Blajchman MA. Transfusion-related mortality: the ongoing risks of allogeneic blood transfusion and the available strategies for their prevention. Blood. 2009;113(15):3406–3417. doi: 10.1182/blood-2008-10-167643.
- Shander A. Financial and clinical outcomes associated with surgical bleeding complications. Surgery. 2007;142(4 Suppl):S20–5. doi: 10.1016/j.surg.2007.06.025.
- Shander A, Hofmann A, Gombotz H, Theusinger OM, Spahn DR. Estimating the cost of blood: past, present, and future directions. Best Pract Res Clin Anaesthesiol. 2007;21(2):271–289. doi: 10.1016/j.bpa.2007.01.002.
- Bracey A, Shander A, Aronson S, et al. The use of topical hemostatic agents in cardiothoracic surgery. Annals Thoracic Surg. 2017;104(1):353–360. doi: 10.1016/j.athoracsur.2017.01.096.
- Tackett SM, Calcaterra D, Magee G, Lattouf OM. Real-world outcomes of hemostatic matrices in cardiac surgery. J Cardiothorac Vasc Anesth. 2014;28(6):1558–1565. doi: 10.1053/j.jvca.2014.05.010.
- Echave M, Oyaguez I, Casado MA. Use of Floseal®, a human gelatine-thrombin matrix sealant, in surgery: a systematic review. BMC Surg. 2014;14:111. doi: 10.1186/1471-2482-14-111.
- Pursifull NF, Morey AF. Tissue glues and nonsuturing techniques. Curr Opin Urol. 2007;17(6):396–401. doi: 10.1097/MOU.0b013e3282f0d683.
- Oz MC, Cosgrove DM, 3rd, Badduke BR, et al. Controlled clinical trial of a novel hemostatic agent in cardiac surgery. The Fusion Matrix Study Group. Annals Thoracic Surg. 2000;69(5):1376–1382. doi: 10.1016/S0003-4975(00)01194-2.
- Weaver FA, Hood DB, Zatina M, Messina L, Badduke B. Gelatin-thrombin-based hemostatic sealant for intraoperative bleeding in vascular surgery. Ann Vasc Surg. 2002;16(3):286–293. doi: 10.1007/s10016-001-0073-0.
- Hutchinson RW, Werrlein S, Johns DB, Zhang G, Clymer JW, Kocharian R. An in vivo comparison of hemostatic gelatin matrix products in a Porcine Spleen biopsy-punch model. Surg Technol Int. 2015;27:53–57.
- Lewis KM, Atlee HD, Mannone AJ, et al. Comparison of two gelatin and thrombin combination hemostats in a porcine liver abrasion model. J Invest Surg. 2013;26(3):141–148. doi: 10.3109/08941939.2012.724519.
- Coenye KE, Bourgain C, Keibl C, Nurnberger S, van Griensven M. A qualitative morphological comparison of two haemostatic agents in a porcine liver trauma model. Surg Sci. 2013;4:359–364. doi: 10.4236/ss.2013.48071.
- US National Research Council. Guide for the care and use of laboratory animals. 8th ed. Washington¸ DC: National Academies Press (US); 2011.
- Lewis KM, Li Q, Jones DS, et al. Development and validation of an intraoperative bleeding severity scale for use in clinical studies of hemostatic agents. Surgery. 2017;161(3):771–781. doi: 10.1016/j.surg.2016.09.022.
- Stokes ME, Ye X, Shah M, et al. Impact of bleeding-related complications and/or blood product transfusions on hospital costs in inpatient surgical patients. BMC Health Serv Res. 2011;11:135. doi: 10.1186/1472-6963-11-135.
- Ye X, Lafuma A, Torreton E, Arnaud A. Incidence and costs of bleeding-related complications in French hospitals following surgery for various diagnoses. BMC Health Serv Res. 2013;13:186. doi: 10.1186/1472-6963-13-186.
- Faivre P, Laplante S, Kreuwel H. Multi European country cost consequence comparison of Floseal matrix and Surgiflo thrombin in major and severe spine surgeries. Value Health. 2015;18(7):A370. doi: 10.1016/j.jval.2015.09.748.
- Makhija D, Rock M, Ikeme S, et al. Cost-consequence analysis of two different active flowable hemostatic matrices in spine surgery patients. J Med Econ. 2017;20(6):606–613. doi: 10.1080/13696998.2017.1292916.
- Makhija D, Rock M, Xiong Y, et al. Cost-consequence analysis of different active flowable hemostatic matrices in cardiac surgical procedures. J Med Econ. 2017;20(6):565–573. doi: 10.1080/13696998.2017.1284079.
- Nogueira L, Katz D, Pinochet R, Kurta JM, Coleman JA. Comparison of gelatine matrix-thrombin sealants used during laparoscopic partial nephrectomy. BJU Int. 2008;102(11):1670–1674. doi: 10.1111/j.1464-410X.2008.07926.x.
- Gazzeri R, Galarza M, Alfier A. Safety biocompatibility of gelatin hemostatic matrix (Floseal and Surgiflo) in neurosurgical procedures. Surg Technol Int. 2012;22:49–54.
- Landi A, Gregori F, Marotta N, Delfini R. Efficacy, security, and manageability of gelified hemostatic matrix in bleeding control during thoracic and lumbar spine surgery: FloSeal versus Surgiflo. J Neurol Surg A Cent Eur Neurosurg. 2016;77(2):139–143.