Abstract
Aim
The aim of this study was to explore the epidermal barrier structure and function of re-harvested skin from non-scalp donor sites.
Methods
Six patients with large-area deep burns who met the inclusion and exclusion criteria were subjected to split-thickness skin excision three times on the same healthy non-scalp donor sites, with an interval of 14 days. The donor skin thus harvested was labeled as primary skin (S1), secondary skin (S2), and tertiary skin (S3). The transepidermal water loss (TEWL) and stratum corneum water content (SCH) of donor skin were detected before each surgery, and the donor skin was harvested during the surgery. The donor skin was stained with hematoxylin and eosin (HE) and involucrin, loricrin, filaggrin, small molecule proline-rich protein 3 (SPRR3), ZO-3, JAM-A, and JAM-C, or observed by transmission electron microscopy.
Results
The epidermal barrier function of the re-harvested skin from the non-scalp donor sites became impaired. The histopathological structure of the re-harvested skin from non-scalp donor sites became abnormal. The barrier of the epidermal stratum corneum of the re-harvested skin from non-scalp donor sites was damaged. The epidermal tight junction barrier in the re-harvested skin from non-scalp donor sites was damaged.
Conclusions
As the number of harvesting increases, the epidermal barrier function of the skin decreased, and the damage to the barrier structure increased. Hence, it is vitally important to restore the epidermal barrier function for re-harvesting in non-scalp donor sites.
Introduction
The fundamental challenge in treating large-area deep burns is the extreme lack of autologous skin.Citation1 Due to insufficient autologous donor skin, it is often impossible to effectively close the wound as soon as possible, resulting in a variety of serious complications and worsening the prognosis of patients. Therefore, how to use the remaining autologous skin effectively remains a critical concern in burn surgery. In addition to the repeated use of the scalp, the repeated use of healthy skin from other parts of the body (non-scalp donor skin) is also inevitable.Citation2
As early as 1942, Brown and McDowellCitation3,Citation4 re-harvested donor skin from the same non-scalp donor site five times to repair large-area deep burn wounds, saving the patient’s life and conceptualizing re-harvesting from non-scalp donor sites. To date, however, the research in non-scalp donor sites remains scant worldwide. On the other hand, in clinical practice, when the skin is re-harvested from the non-scalp donor sites, the split-thickness skin appears to be brittle, poorly elastic, and difficult to stretch and cut, making the processing and utilization of such skin a big challenge. Studies have established that skin elasticity and resistance to traction are closely associated with the barrier structure and function of the epidermis,Citation5,Citation6 but little is known about the epidermal barrier function of re-harvested skin from non-scalp donor sites.
This study attempts to fill the gap by probing the epidermal barrier structure and function of re-harvested skin from non-scalp donor sites in order to provide an alternative solution to insufficient donor skin in patients with large-area deep burns.
Materials and methods
Sample collection and ethical approval
Six patients with large-area deep burns treated in our hospital were enrolled in this study. Inclusion criteria: patients who met the diagnostic criteria for large-area deep burns; burn area: ≥30% total body surface area (TBSA); burn depth: II-IV degree; healthy skin on the trunk or limbs; admitted within 48 h post-injury; aged 18–59 years old; consented to participate in the study. Exclusion criteria: patients with blood system diseases, organ dysfunction diseases, immune system diseases, malignant tumors, diabetes before burns; not suitable for surgery; mental illness; unable to cooperate with treatment or recovery.
The enrolled patients were all male, with an average age of (32.33 ± 8.76) years old and an average burn area of (79.67 ± 14.09) %TBSA. Upon admission, the patients received active rehydration for anti-shock therapy to maintain hemodynamic stability, correct hypoalbuminemia, electrolyte balance disturbance, and acid-base imbalance, and protect vital organ functions. Early wounds were treated with silver sulfadiazine or bandaging and kept dry using a suspended bed; large-scale far-infrared irradiation was used to prevent early scab dissolution of necrotic tissue, in addition to active scab protection. Skin grafting was scheduled when the patients showed signs of stable conditions.
All patients underwent skin harvesting from the same non-scalp donor site at least three times. The donor sites for such re-harvesting among the six patients were: the abdomen (n = 2), back (n = 1), and lower limbs (3). The time interval between re-harvesting was two weeks. The donor site wound was treated with Vaseline gauze. Each time the skin was harvested, 4 cm2 of split-thickness skin was taken for following experiments, and the skin thus harvested three times was labeled as primary skin (S1), secondary skin (S2), and tertiary skin (S3).
This study was approved by the appropriate ethics committee of our hospital with an approval number (2021KY026-HS001). The trial was registered at the China Clinical Trial Center with a registration number (ChiCTR2200059432).
TEWL and SCH detection
As described in the instructions, the transepidermal water loss (TEWL) and stratum corneum water content (SCH) in the donor sites were detected by GPSkin Barrier (GPOWER Inc, Seoul, South Korea) at room temperature when the patient was in a calm state prior to surgery.
HE staining
The skin (2 cm2) harvested as described before was fixed in paraformaldehyde for 24 h, embedded with a paraffin-embedding machine, cut into 5 μm thickness with a microtome, and dewaxed using two Toluene, absolute ethanol, 95% ethanol solution, 80% ethanol solution, 75% ethanol solution, 95% ethanol solution and double distilled water in sequence. The dewaxed sections were stained with hematoxylin, separated with 1% hydrochloric acid-ethanol solution, rinsed with water, and then dehydrated using 70% ethanol solution and 95% ethanol solution in sequence. The dehydrated sections were stained with eosin, and a neutral gum mounting medium was added to the sections, which were then observed under an optical microscope and photographed.
IHC staining
After dewaxing, the sections were soaked in EDTA buffer for antigen recovery. After the sections were blocked in BSA for 30 min, filaggrin antibody (diluted 1:200; ab81468, Abcam, Cambridge, MA, USA), loricrin antibody (diluted 1:200; ab137533, Abcam), involucrin antibody (diluted 1:150; ab53112, Abcam), jam-a antibody (diluted 1:200; ab267407, Abcam), jam-c antibody (diluted 1:50; ab224327, Abcam), zo-3 antibody (diluted 1:200; ab181991, Abcam), small proline-rich region protein 3 (SPRR3, diluted 1:100; GTX65568, Genetex, San Antonio, TX) was added and incubated at 4 °C overnight. HRP-conjugated Goat Anti-Rabbit IgG diluted 1:200; 52200336, SeraCare, Milford, MA, USA)or HRP-conjugated Goat Anti-Mouse IgG (diluted 1:200; 52200341, SeraCare) was added, and incubated for 50 min. After the section was slightly dried, the DAB reagent was added to the circle. The sections were then rinsed with distilled water, counterstained with hematoxylin, differentiated with 1% hydrochloric acid alcohol, and rinsed with tap water before ammonia was added. Finally, the sections were dehydrated with absolute ethanol, sealed with xylene transparent neutral gum, observed, and photographed under an optical microscope. Mean optical density values (IOD) were analyzed with Image pro plus 6.0 (Media Cybernetics, MA, USA).
Transmission electron microscopy
The skin was taken and fixed at room temperature in a solution containing 2.5% glutaraldehyde and 2% paraformaldehyde for 48 h. The specimens were washed in PBS buffer three times (5 min each time). After further fixed in 1% osmic acid solution for two h, dehydrated with acetone, infiltrated, and embedded with epoxy resin, the specimens were made into 70 nm sections. The lamellar bodies in the spinous layer and the granular layer were observed and photographed under a transmission electron microscope.
Statistical analysis
All data were presented as mean ± SD and analyzed using SPSS 23.0. The one-way and two-way analysis of variance (ANOVA) was used for the overall comparison between the groups, and the LSD test was used for the pairwise comparison between the groups (the software automatically omitted this value). p < 0.05 was considered statistically significant.
Results
Impaired epidermal barrier function
This study explored the barrier function of the skin based on TEWL and SCH, the two most used indicators for evaluating epidermal barrier functions, and performed a statistical analysis. It was found that the TEWL of S2 and S3 were significantly increased, and the SCH was significantly decreased in S2 and S3 than in S1; the TEWL was significantly increased, and the SCH was significantly decreased in S3 compared with S2 (). This finding indicated that the barrier function of the skin was impaired as it was re-harvested at an interval of 14 days.
Figure 1. The TEWL and SCH of S1, S2, and S3. (A) TEWL. (B) SCH. This study explored the barrier function of the skin based on TEWL and SCH, the two most used indicators for evaluating epidermal barrier functions. S1, primary skin; S2, secondary skin; S3, tertiary skin; TEWL, transepidermal water loss; SCH, stratum corneum water content. Scale bars, 50 μm. *p < 0.05,**p < 0.01. n = 6. Data shown as mean ± SD.
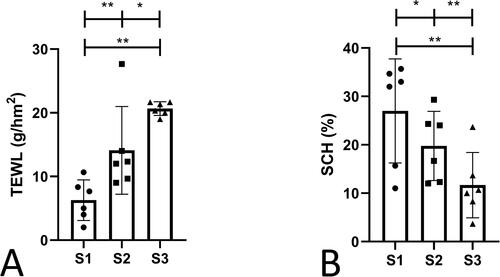
Abnormal histopathological structure
In this study, HE staining was used to evaluate the histopathological structure of the skin. S2 and S3 showed obvious epidermal hyperplasia, thickened granular layer, and acanthus layer, and a significant reduction in melanocytes in the basal layer compared with S1. Compared with S2, the cells in the basal layer and the spinous layer were widened, and intercellular edema appeared in S3 (). At the 14-day interval, the epidermal histopathological structure of the re-harvested skin was abnormal.
Damaged epidermal stratum corneum barrier
The stratum corneum barrier structure of the skin consists of a variety of proteins and structural lipids. In the present study, the distribution and expression of filaggrin, loricrin, involucrin, and SPRR3 were employed to evaluate the proteins, and the density of lamellar bodies was used to evaluate the structural lipids (). The immunohistochemical results revealed that the expression of filaggrin, loricrin, involucrin, and SPRR3 was significantly decreased in S2 and S3 than in S1, which was dramatically decreased in S3 compared with S2. Electron microscopy results showed that the number of epidermal lamellar bodies in S2 and S3 were significantly reduced compared with S1, which was remarkably reduced in S3 than in S2. These findings suggested that at the 14-day interval, the epidermal stratum corneum barrier was damaged, and the damage was aggravated as the skin was re-harvested.
Figure 3. The expressions of structural proteins indicating epidermal stratum corneum barriers and the structural lipids. A. filaggrin. B. involucrin. C. loricrin. D. SPRR3. E. observation of epidermal lamellar bodies. F. expression analysis of structural proteins indicating epidermal stratum corneum barriers. The stratum corneum barrier structure of the skin consists of a variety of proteins and structural lipids. The distribution and expression of filaggrin, loricrin, involucrin and SPRR3 were employed to evaluate the proteins, and the density of lamellar bodies was used to evaluate the structural lipids. S1, primary skin; S2, secondary skin; S3, tertiary skin. Brown staining indicates target protein expression. Red arrows indicate lamellar bodies. Scale bars are shown at the bottom of each figure. *p < 0.05, **p < 0.01. n = 6. Data shown as mean ± SD.
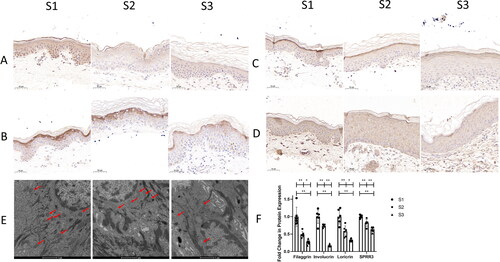
Impaired epidermal tight junction barrier
In this study, the tight junctions of the epidermis were also discussed. JAM-A, JAM-C, and ZO-3 were used to analyze the distribution and expression of tight junctions. IHC staining results showed that the expressions of JAM-A, JAM-C, and ZO-3 were significantly decreased in S2 and S3 compared with S1, which was remarkably reduced in S3 than in S2 ().
Figure 4. The expression of epidermal tight junction proteins and observation of epidermal tight junction. A. JAM-A. B. JAM-C. C. ZO-3. D. observation of tight junctions in the epidermis. E. the expression of epidermal tight junction proteins. JAM-A, JAM-C, and ZO-3 were used to analyze the distribution and expression of tight junctions. S1, primary skin; S2, secondary skin; S3, tertiary skin. Brown staining indicates target protein expression. Yellow arrows indicate vacuoles. Blue arrows indicate tight junction breaks. Scale bars are shown at the bottom of each figure. *p < 0.05, **p < 0.01. n = 6. Data shown as mean ± SD.
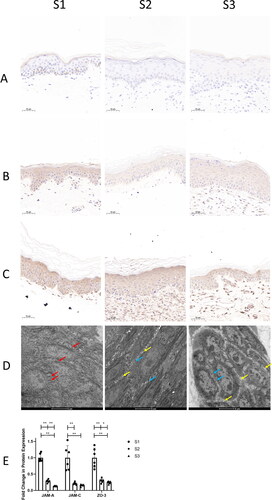
Observation under electron microscopy showed that there were dense tight junctions between the epidermal cells of the spinous layer in S1, which were in the shape of lines with intact structures; a small number of tight junctions between the epidermal cells in S2 were broken, and a small number of vacuoles appeared at the junction of the cytoplasm and the cell membrane; the gaps between the epidermal cells in S3 increased, with a number of broken tight junctions with incomplete structures ().
The findings indicated that at the 14-day interval, the epidermal tight junction barrier of the non-scalp donor site after healing was damaged, and the damage was aggravated with the increase in skin harvesting times.
Discussion
In the clinical treatment of patients with large-area deep burns, the scalp is considered to be a natural source of skin because it is inexhaustible. Previous studies have shown that after repeated scalp harvestings, the skin quality is still good, and no scars are left on the scalp after healing. Therefore, the scalp has become the first choice and the most important donor site for patients with extensive deep burns.Citation7 However, in practice, for some burn wounds that are too large, especially among patients with deep burns on the scalp, healthy skin other than the scalp has to be used to close the wound. Therefore, it is vitally important to understand the barrier structure and function of the skin from donor sites other than the scalp.
Researchers have shown that the epidermis is in direct contact with the external environment and is responsible for bearing external mechanical forces and regulating TEWL, thus assuming important functions as a barrier.Citation8 The epithelization and remodeling of the epidermis can be completed on the donor site wound two weeks after the split-thickness skin is harvested; hence, it is generally considered that the skin can be re-harvested at an interval of 14 days. However, the wounds on donor sites usually take up to six weeks to heal completely, which means that the skin has not fully returned to its normal physiological state two weeks after the last harvesting.Citation9 The healed skin is prone to rupture when re-harvested after such a short interval, and this is obviously associated with the changes in the skin-epidermal barrier function after re-harvesting. Since there is no evidence to validate this conclusion, the present study is tasked with doing so. To avoid the limitations common in exploring only one donor site, this study selected multiple sites, including the lower limbs, abdomen, and back according to the conditions of each patient. And three consecutive skin samples (S1, S2, and S3) were used for research, which is representative of examining the structural and functional changes in the skin re-harvested from these donor sites.
The epidermal barrier is mainly composed of the stratum corneum barrier and the tight junction barrier. Researchers use the brick wall model to describe the skin stratum corneum barrier. The cornified cell envelope (CE) formed by structural proteins are the bricks in the model, while the intercellular lipids are the mortar between the bricks.Citation10
The structural proteins of CE include filaggrin, loricrin, involucrin, SPRR, etc., which play a key role in providing resistance to external mechanical tension, preventing the invasion of pathogens and allergens, and preventing excessive water loss in the body.Citation11 Filaggrin is mainly expressed in the granular layer and the transparent layer of the epidermis, which interacts with keratin intermediate filaments and aggregates to form dense keratin fiber bundles, thereby forming a flat and tough scaffold structure of keratinocytes.Citation12 With the participation of SPRR, loricrin and involucrin cross-link with each other to form an abnormally water-insoluble CE, wrapping keratin fiber bundles and forming the unique stratum corneum barrier structure of the epidermis.Citation12 In this study, these proteins were analyzed by IHC staining. The results revealed that the expression of CE structural proteins in the skin tended to decrease as the skin was re-harvested. As a result, the connection between keratin proteins was weakened, thus impairing the barrier function.
Intercellular lipids are secreted by lamellar bodies in the spinous and granulosa cells, serving as part of the epidermal structure, which is metabolized together with the skin tissue. Lamellar bodies are membrane-enclosed secretory organelles, and lipids in them are exocytosed into the extracellular matrix at the interface between the granular layer and the stratum corneum to form the lamellar membrane.Citation13 A body of literature has shown that lamellar bodies play an important role in lipid transport in the epidermis, and their absence or dysfunction can lead to skin barrier disturbances.Citation14,Citation15 In the present study, the number of lamellar bodies in the skin tended to decrease as the skin was re-harvested, suggesting that the secretion of lipids into the extracellular matrix may be reduced, and the stratum corneum barrier may be damaged.
There are four intercellular junctions known to researchers: tight junctions, adhesive junctions, desmosome junctions, and gap junctions.Citation16 Among these intercellular junctions, tight junctions are very critical for maintaining the barrier function of epidermal cells, and the main functions of tight junctions are as follows: tight junctions can connect cells together tightly, making cells less susceptible to damage; tight junction structures and/or tight junction proteins can control molecular paracellular pathways, and are selective for molecular size, ion type, and cell permeability; tight junctions can separate the lipids of the basal part of the cell membrane to the top of the cell membrane to form two different membrane functional regions, thereby preventing the mutual diffusion between the two different functional regions, so as to maintain the exclusive function of each surface of the cell and maintain the cell’s polarity.Citation17 Tight junctions function through claudins, which are mainly composed of transmembrane proteins JAM, claudin, and cytoplasmic proteins zonula occludens (ZO). Abnormal expression of these tight junction-related proteins will lead to the breakdown of barrier functions, causing the penetration of harmful molecules, inducing the activation of the immune system, and resulting in persistent inflammation and skin damage.Citation18 It has been shown that JAM-A is a crucial membrane protein constituting tight junctions. JAM-A appears in the early stage of tight junction formation and is enriched in tight junctions between epithelial cells with proteins such as occludin and claudin.Citation19 In addition to constituting the tight junction barrier, JAM-A also impacts the proliferation of epidermal cells by regulating the β1-integrin and β-catenin pathways. Another critical membrane protein, JAM-C, is distributed in various tissues and organs and is expressed in endothelial cells and epithelial cells. Vertebrate epithelial cells and endothelial cells are highly polarized, and the cell membrane is divided into two parts, apical and basal, by tight junctions, which restrict the free flow of membrane proteins and lipids and play a role in fixation.Citation20 ZO-3 is a cytoplasmic protein closely associated with epidermal cell tight junctions and was found to be closely related to the barrier function and permeability regulation of the epidermis in a zebrafish model.Citation21 Accordingly, the expression of JAM-A, JAM-C, and ZO-3 was selected in this study, combined with ultrastructural observation to probe the tight junctions between epidermal cells. As a result, the tight junctions of the skin were damaged after re-harvesting, and the same with the stratum corneum barrier, the damage aggravated as the skin was re-harvested.
In the present study, TEWL and SCH, which are commonly used to reflect the function of the epidermal barrier, were detected, and the results also showed that the epidermal stratum corneum barrier and tight junction barrier were damaged, in line with the results of IHC staining and electron microscopy. Previous studies have shown that the donor site wound can be healed, and the skin can be re-harvested after two weeks of skin harvesting.Citation3,Citation4 According to the results of this study, nevertheless, despite the fact that donor site wounds have been epithelialized two weeks after injury, the barrier function of the skin has not recovered to a normal physiological status. When such skin is transplanted into the burn wound, it cannot effectively prevent water evaporation and microbial infestation. In addition, mechanical traction can easily break the grafted skin and affect healing. Obviously, the complete restoration of the skin barrier function requires a longer time of recovery. However, for patients with extensive deep burns, a long interval for skin harvesting always results in delayed treatment, hence an antagonistic relationship between the skin harvesting interval and the skin barrier function. However, the mechanism of skin re-harvesting leading to the damage of the barrier function of the skin is not clear, and further research is thus needed. It will also be interesting to examine whether there is any difference in the structure and function of re-harvested skin from the scalp and other donor sites.
A better understanding of the biological characteristics of skin re-harvesting will facilitate wound repair in patients with large-area deep burns. From the present study, it can be seen that the mainstream treatment method of the donor site wound covered with vaseline gauze cannot repair the skin quickly and well. Therefore, a more effective solution is urgently needed. With the continuous development of science and technology, a variety of new artificial dressings and biological dressings have been widely used in the repair of burn wounds and achieved good results, but the treatment of donor site wounds and corresponding research is scarce. More importantly, no researcher has ever explored the barrier function of re-harvested skin. This study provides new targets and research indicators for the treatment of wounds on the donor site. More research in this area may address the insufficient donor sites for extensive deep burns.
Disclosure statement
No potential conflict of interest was reported by the author(s).
Additional information
Funding
References
- Liu HF, Zhang F, Lineaweaver WC. History and advancement of burn treatments. Ann Plast Surg. 2017;78(2 Suppl 1):S2–S8.
- Stanton RA, Billmire DA. Skin resurfacing for the burned patient. Clin Plast Surg. 2002;29(1):29–51. doi:10.1016/s0094-1298(03)00085-3
- Brown JB, McDowell F. Massive repairs of burns with thick split-skin grafts: emergency "Dressings" with homografts. Ann Surg. 1942;115(4):658–674. doi:10.1097/00000658-194204000-00017
- Brown JB, McDowell F. Epithelial healing and the transplantation of skin. Ann Surg. 1942;115(6):1166–1181. doi:10.1097/00000658-194206000-00027
- Menon GK, Kligman AM. Barrier functions of human skin: a holistic view. Skin Pharmacol Physiol. 2009;22(4):178–189. doi:10.1159/000231523
- Nemes Z, Steinert PM. Bricks and mortar of the epidermal barrier. Exp Mol Med. 1999;31(1):5–19. doi:10.1038/emm.1999.2
- Oh SJ. Simultaneous two-layer harvesting of scalp split-thickness skin and dermal grafts for acute burns and postburn scar deformities. Arch Plast Surg. 2019;46(6):558–565. doi:10.5999/aps.2018.00990
- Ye C, Yi J, Lai W, Zheng Y. Skin barrier damaging and repairing process: A new application field of dermoscopy. J Cosmet Dermatol. 2021;20(3):897–905. doi:10.1111/jocd.13643
- Hinshaw JR, Miller ER. Histology of healing split-thickness, full-thickness autogenous skin grafts and donor sites. Arch Surg. 1965;91(4):658–670. doi:10.1001/archsurg.1965.01320160112027
- Kim Y, Lim KM. Skin barrier dysfunction and filaggrin. Arch Pharm Res. 2021;44(1):36–48. doi:10.1007/s12272-021-01305-x
- Proksch E, Brandner JM, Jensen JM. The skin: an indispensable barrier. Exp Dermatol. 2008;17(12):1063–1072. doi:10.1111/j.1600-0625.2008.00786.x
- Bouwstra JA, Helder RWJ, El Ghalbzouri A. Human skin equivalents: Impaired barrier function in relation to the lipid and protein properties of the stratum corneum. Adv Drug Deliv Rev. 2021;175(:113802. doi:10.1016/j.addr.2021.05.012
- Wertz P. Epidermal lamellar granules. Skin Pharmacol Physiol. 2018;31(5):262–268. doi:10.1159/000491757
- Menon GK, Lee SE, Lee SH. An overview of epidermal lamellar bodies: Novel roles in biological adaptations and secondary barriers. J Dermatol Sci. 2018;92(1):10–17. doi:10.1016/j.jdermsci.2018.03.005
- Menon GK, Orso E, Aslanidis C, Crumrine D, Schmitz G, Elias PM. Ultrastructure of skin from Refsum disease with emphasis on epidermal lamellar bodies and stratum corneum barrier lipid organization. Arch Dermatol Res. 2014;306(8):731–737. doi:10.1007/s00403-014-1478-2
- Arnold TR, Stephenson RE, Miller AL. Rho GTPases and actomyosin: Partners in regulating epithelial cell-cell junction structure and function. Exp Cell Res. 2017;358(1):20–30. doi:10.1016/j.yexcr.2017.03.053
- Basler K, Brandner JM. Tight junctions in skin inflammation. Pflugers Arch. 2017;469(1):3–14. doi:10.1007/s00424-016-1903-9
- Basler K, Bergmann S, Heisig M, Naegel A, Zorn-Kruppa M, Brandner JM. The role of tight junctions in skin barrier function and dermal absorption. J Control Release. 2016;242:105–118. doi:10.1016/j.jconrel.2016.08.007
- Brandner JM. Tight junctions and tight junction proteins in mammalian epidermis. Eur J Pharm Biopharm. 2009;72(2):289–294. doi:10.1016/j.ejpb.2008.08.007
- Garrido-Urbani S, Vonlaufen A, Stalin J, et al. Junctional adhesion molecule C (JAM-C) dimerization aids cancer cell migration and metastasis. Biochim Biophys Acta Mol Cell Res. 2018;1865(4):638–649. doi:10.1016/j.bbamcr.2018.01.008
- Hunziker W, Kiener TK, Xu J. Vertebrate animal models unravel physiological roles for zonula occludens tight junction adaptor proteins. Ann N Y Acad Sci. 2009;1165:28–33. doi:10.1111/j.1749-6632.2009.04033.x