Abstract
Objective
Glucocorticoids are widely used in clinical practice; however, they can cause side effects, such as osteoporosis. Acteoside (ACT) from Cistanche has been used to combat a variety of diseases. The study was conducted to evaluate the efficacy of ACT in glucocorticoid-induced osteoporosis (GIOP) and its potential mechanism.
Methods
Dexamethasone (Dex) was injected intramuscularly to induce osteoporosis in a rat model, and ACT was given orally. ACT was supplemented in vivo in Dex-stimulated osteoblastic MC3T3-E1 cells. RT-qPCR was performed to assess the mRNA levels of bone formation (Runx2, CoL1A1), and bone resorption (OPG and RANKL). A commercial ELISA kit was applied to assess serum OC and CTX levels. Western blot was performed to assess protein levels in the PI3K/AKT/mTOR signaling pathway. CCK-8 assay and flow cytometry were performed to assess osteoblast viability and apoptosis.
Results
ACT reduced Dex-induced bone microstructure deterioration, increased serum levels of OC, and decreased the levels of CTX (P < 0.05). In the MC3T3-E1 cells, Dex inhibited cell viability and promoted apoptosis; however, this effect was greatly attenuated by ACT (P < 0.05). Concurrently, ACT reversed the reduction in Runx2, osterix, CoL1A1, and OPG mRNA levels, ALP activity, and the promotion of RANKL by Dex. Additionally, ACT attenuated Dex-induced inhibition of p-AKT/AKT, p-mTOR/mTOR, and p-PI3K/PI3K protein levels by Dex (P < 0.05), while the PI3K/AKT/mTOR pathway inhibitor LY294002 diminished the potential effect of ACT (P < 0.05).
Conclusion
ACT from Cistanche may exert osteoprotective effects by activating the PI3K/AKT/mTOR signaling pathway to alleviate Dex-induced osteoporosis.
Introduction
Osteoporosis (OP) is a generalized skeletal disease characterized by low bone mass, destruction of bone tissue microstructure, and imbalance in bone homeostasis [Citation1]. OP causes more than 8.9 million fractures per year and osteoporotic fractures occur almost every 3 seconds, and leading to lifelong disability or death [Citation2]. Glucocorticoids (GCs) are commonly administered for the treatment of noninfectious inflammatory diseases (such as asthma and inflammatory bowel disease) as well as autoimmune conditions [Citation3]. However, long-term GC use predisposes to more than 30% of OP cases and more than 10% of osteonecrosis cases [Citation4]. GCs directly inhibit osteoblast proliferation and differentiation [Citation5], diminish maturation and activity, and induce apoptosis of osteoblasts, which in turn leads to the development of glucocorticoid-induced osteoporosis (GIOP) [Citation6]. Moreover, growing evidence suggests that osteoblast dysfunction is the major cause of bone loss. Therefore, an increase in the number and function of osteoblasts appears to be the main strategy to prevent of OP, especially GIOP.
Herbal medicines, especially those based on natural compounds, have been practiced for thousands of years for the treatment of various diseases [Citation7]. Cistanche is a precious herb recorded in the Chinese Pharmacopeia that grows in the southern area of the Xinjiang Uygur Autonomous Region and is known as desert ginseng [Citation8]. Cistanche has been proven to have various effects, such as immune enhancement, renal protection, anti-ageing, antioxidant, anti-inflammatory, and neuroprotective effects [Citation9]. Acteoside (ACT) is a phenyl ethanolic glucoside in Cistanche, which has biological activities, such as inhibition of neuronal apoptosis [Citation10], alleviation of liver damage [Citation11], anti-inflammation [Citation12], antioxidation [Citation13], prevention of diabetes [Citation14], and obesity [Citation15], as well as the degeneration of the articular cartilage [Citation16]. Furthermore, several studies have reported the pharmacokinetics of ACT and confirmed that the therapeutic effect exists even if the oral utilization is only 0.12%. ACT is considered a prodrug with active metabolites in vivo [Citation17].
Zhang et al. demonstrated a potential protective effect of ACT on ovariectomy-induced bone loss in rats [Citation18]. ACT modulates the IGF-1/PI3K/mTOR signaling pathway to prevent bone loss in diabetic rats [Citation19]. The PI3K/AKT/mTOR pathway has been reported to have critical regulatory roles in various diseases, including GIOP [Citation20]. Naringin alleviates GIOP by regulating the PI3K/AKT/mTOR signaling pathway [Citation21]. Additionally, this signaling pathway regulates osteoblast differentiation [Citation22], bone formation [Citation23], and fracture healing [Citation24]. However, the potential role of ACT on GIOP and whether it is regulated by the modulation of the PI3K/AKT/mTOR signaling pathway is unknown.
The present study aimed to understand the protective effects of ACT in a GIOP rat model and osteoblasts in vitro and to elucidate the underlying molecular mechanisms.
Materials and methods
Experimental animals
Forty 8-week-old male Sprague-Dawley (SD) rats weighing 280–340 g were obtained from Shanghai Laboratory Animal Center (CAS, Shanghai, China). The care procedures of the experimental animals were carried out following the protocol approved by The Third Affiliated Hospital of Qiqihar Medical University Animal Ethics Committee. And the study was conducted in agreement with the ARRIVE guidelines. Animals were housed in animal centers free of pathogens, 24 ± 2 °C, 12 h light and dark cycles, 50 ± 5% humidity, and with free access to food and water.
GIOP model construction
After 7 days of adaptive feeding, rats were randomly divided into control and model groups using the random number table method, Dexamethasone (Dex, Sigma-Aldrich St. Louis, MO, USA) 5 mg/kg twice a week for 6 weeks was injected intramuscularly to induce OP in rats in the model group [Citation25]. Rats in the control group (n = 10) were injected with an equal amount of saline. No significant changes in body weight were observed in the rats in both groups at 6 weeks. Subsequently, the rats in the model group were subdivided into the model group, ACT low dose group (Model + ACT-L) group, and ACT high dose group (Model + ACT-H) group. ACT (HPLC 98%) was obtained from Baoji Herbest Bio-tech., Ltd. (Baoji, China), and treated with an aqueous solvent suspension. A dose of 50 mg/kg/d and 100 mg/kg/d ACT was administered orally to rats in the low and high dose groups (8 rats per group), respectively, and was continued for 8 weeks. Among them, the sample size was calculated based on a previous study [Citation26]. Considering a two-sided significance level of 0.05 [95% confidence interval (α = 0.05)], 80% power, and an effect size of 0.5, power analysis estimated a needed sample size of 6 animals per group assuming a relevant effect level of 30% osteoporosis. Further, with a dropout rate of 20%, 8 animals per group (total of 32 rats) were considered ideal.
Rat serum samples
After 8 weeks, rats were anesthetized with 10% chloral hydrate (400 mg/kg, intraperitoneally, Solarbio, Beijing China), blood was collected from the abdominal aorta, clotted at room temperature, and centrifuged at 3500 rpm/min for 10 min. The serum was stored in 1.5 mL centrifuge tubes until further use.
Bone mineral density assessment
Bone mineral density (BMD) was using by a DAX small animal bone densitometer (Ranzhe Instrument Equipment Co., Shanghai, China). Briefly, the rats were placed in the supine position and the hind limbs were positioned outward. The right femur of the rats was scanned according to the manufacturer’s instructions, and the BMD values were recorded [Citation27].
Bone morphology analysis
At the end of the experiment, the rats were euthanized by intraperitoneal injection of excess chloral hydrate, and the femurs were fixed in 70% ethanol. Subsequently, scans were performed on a VivaCT 40μCT system as described in previous study and the bone volume/total volume (BV/TV), bone trabecular number (Tb.N), bone trabecular thickness (Tb.Tn), and bone trabecular separation (Tb.Sp) were assessed [Citation28]. For euthanasia, SD rats were anesthetized after the bone morphology analysis by an intraperitoneal injection of 10% chloral hydrate (350 mg/kg).
Enzyme-linked immunosorbent assay (ELISA)
Commercial ELISA kits were used for the detection of osteocalcin (OC, E-EL-R0243c, Elabscience) and C-terminal telopeptide (CTX, E-EL-R1405c, Elabscience) levels in the serum of rats. Briefly, the reagents were removed at 18-25 °C to equilibrate and prepare the solution for washing and standard working. Enzyme plates Plates were set up with standard, blank, and sample wells. About 100 μL sample diluent solution was added to the wells, incubated for 90 min at 37 °C, and replaced with a biotinylated antibody for 60 min at 37 °C. After washing, the enzyme-binding working solution was added and incubated for 30 min. The substrate solution (90 μL) was added, and incubated for 15 min with protection from light. When the blue gradient appeared in the standard wells, 50 μL of the termination solution was added and the change in OD450 was analyzed.
Reverse transcription-quantitative real-time PCR (RT-qPCR)
TRlzol LS reagent was added to the serum of rats and ACT-treated MC3T3-E1 cells, and total RNA was extracted by incubation at room temperature followed by the addition of chloroform. RNA concentration and purity were confirmed by measuring A260/280 absorbance in a Nanodrop ND-1000 spectrophotometer. The SuperScript II Reverse Transcriptase kit was used for the reverse transcription of RNA to cDNA. Subsequently, cDNA was applied as a template, a primer was added, and the SYBR-Green PCR master mix kit was mixed and supplemented with ddH2O to 20 μL, and the PCR amplification reaction was performed using the Applied biosystems AMI7500 Fast Real-time PCR system (ABI, CA, USA). GAPDH was used as an internal reference for normalization, and the relative expression levels were calculated using 2−ΔΔCt method and performed in triplicates. The primer sequence for Runt-related transcription factor 2 (Runx2), collagen type I α1 (CoL1A1), receptor activator of nuclear factor-κB ligand (RANKL), osterix, and osteoprotegerin (OPG) are presented in .
Table 1. Sequences of primers designed for amplification of genes.
Western-blot analysis
RIPA lysate containing 1% protease inhibitor was added to the cells, and total protein from each group was collected after lysis of the cells by shaking for 5 min at 4 °C. BCA assay kit was employed to quantify the concentration of proteins in each group. Following this, the protein samples were mixed with 10% sodium dodecyl sulfate (SDS) buffer in equal volume and boiled in a water bath at 95 °C for 5 min for protein denaturation. A 12% SDS-polyacrylamide vertical gel was prepared, and after the gel solidified, 30 μg of protein was sampled for the 120 mA 90 min gel electrophoresis separation. The protein was then transferred onto the PVDF membrane at 90 V for 120 min. After sealing with 5% bovine serum albumin, the PVDF membrane was cut based on the molecular weight of the marker and target protein band and incubated with the corresponding primary antibody overnight at 4 °C. Rabbit polyclonal antibodies against p-AKT, p-mTOR, and p-PI3K were diluted to ratio of 1:1000 (Proteintech, Wuhan, China). TBST was washed for 30 min and incubated with the corresponding horseradish peroxidase-labeled secondary antibody at room temperature for 1 h. The blot was visualized using enhanced chemiluminescence reagents. The density of protein bands was quantified using Image J. GAPDH was employed as a control to calculate relative protein levels.
Cell culture and grouping
Mouse pre-osteoblasts MC3T3-E1 were purchased from the Chinese Tissue Culture Collections (CTCC, China) and cultured in α-MEM containing 10% fetal bovine serum and 100 U/mL penicillin, and 100 mg/mL streptomycin in a humidified incubator at 37 °C and 5% CO2. For bone differentiation culture, osteogenic induction medium was made by mixing 10 mM-β-glycerophosphate and 50 mg/mL of ascorbic acid (Sigma-Aldrich) with α-MEM and added to the MC3T3-E1 cells to induce their differentiation. The medium was changed after 3 days. Cultures for 14 days were used for Alkaline phosphatase (ALP) viability. Furthermore, cells were pretreated with the PI3K inhibitor LY294002 (10 μM) for 30 min, followed by treatment with Dex and ACT.
Cell viability assay
The Cell Counting Kit-8 (CCK-8) assay was performed to assess the viability of osteoblasts treated with ACT. The MC3T3-E1 cells were inoculated into 96 well plates at 5 × 103 cells/well. A quantity of 1 μM of Dex was considered based on previous studies [Citation29] and co-incubated with gradient concentrations of ACT (10−8, 10−7, 10−6, and 10−5 M). The culture medium in the 96-well plates was replaced after adding a mixing medium and CCK-8 reagent in a 10: 1 ratio. After continued incubation for 1 h at 37 °C, the change in OD450 was assessed.
Osteoblast apoptosis number detection
The MC3T3-E1 cells that were subjected to different treatments were digested using EDTA-free trypsin and collected by centrifugation. After washing with a 1 × binding buffer, 5 μL Annexin V-FITC was added and incubated at room temperature for 5 min, supplemented with 5 μL PI and passed through a 200-mesh nylon net. The samples were analyzed using FACScan flow cytometry system.
ALP activity assay
The MC3T3-E1 cells were inoculated into 24-well plates, and ACT and Dex were added after plastering. The cell culture medium was replaced with an osteogenic differentiation medium for culture. After using lysis of cells by cell lysis solution (P0012J, Beyotime Biotechnology), the supernatant was collected by centrifugation, and the standard curve was plotted. An ALP kit (P0132S, Beyotime Biotechnology) was used to evaluate ALP activity and the OD450 was calculated.
Statistical analysis
After performing three independent experiments in triplicate, the data were analyzed using GraphPad Prism 6.0 software and expressed as mean ± standard deviation. ANOVA was employed to compare statistical differences between multiple groups. P-value < 0.05 was considered statistically different.
Results
ACT alleviates bone mineral density loss and histological changes in the rat GIOP model
BMD was typically reduced in the rat model of the Dex-induced OP rat model compared to the control group, and this reduction was significantly reversed after high-dose ACT treatment (P < 0.05, ). Subsequently, microstructural changes in the femur were assessed quantitatively. As illustrated in , BV/TV, Tb.N, and Tb.Th were markedly decreased in the GIOP rat model, while Tb.Sp was significantly increased (P < 0.05). The findings suggest severe deterioration of bone microarchitecture in GIOP. However, treatment with ACT improved this deterioration, with significant increases in BV/TV, Tb.N, and Tb.Th and a significant decrease in Tb.Sp (P < 0.05) compared to the model group.
Figure 1. Effect of ACT on BMD and bone microstructure in the GIOP rat model. A. ACT significantly reversed BMD in the Dex-induced OP rat model. B–E. Changes in the microarchitectural parameters of bone such as BV/TV, Tb.N, Tb.Th, Tb.Sp in response to ACT treatment and Dex induction. BV/TV, bone volume over total volume; Tb.N, trabecular number; Tb.Th, trabecular thickness; Tb.Sp, trabecular separation; ACT-L, 50 mg/kg/d ACT; ACT-H, 100 mg/kg/d ACT. N = 8, ***P < 0.001 vs. control; ##P < 0.01, ###P < 0.001 vs. Model.
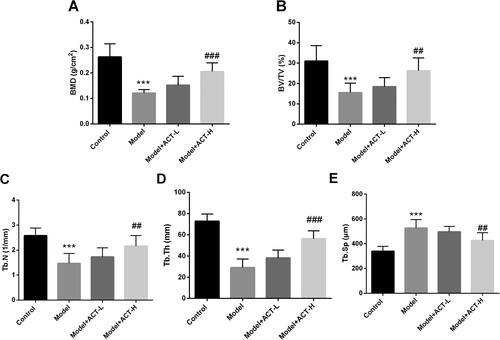
Regulation of osteoblast differentiation by ACT in the GIOP rat model
Compared with rats in the control group, Dex significantly reduced ALP activity and the serum levels of the bone formation biomarker OC in GIOP rats; however, this inhibition was reversed by ACT treatment (P < 0.05, ). In contrast, the levels of CTX, a biomarker of bone resorption, were markedly increased by Dex; however, the levels were reduced after the administration of ACT (P < 0.05, ). The mRNA levels of bone metabolism genes were assessed to further investigate the potential role of ACT in bone formation and resorption. Dex inhibited the levels of Runx2, osterix, and COL1A1 in the osteogenic differentiation-related genes, decreased OPG levels in bone resorption-related genes and increased the expression of the osteoclast activator RANKL (P < 0.05, ). However, ACT treatment significantly diminished the regulation of these bone metabolism genes by Dex (P < 0.05, ). Taken together, ACT inhibited the Dex-induced decrease in bone formation and increase in bone resorption.
Figure 2. Regulation of bone metabolism in the Dex-induced OP rat model by ACT. A. ALP activity assay kit was used to evaluate serum ALP levels. ELISA was performed to assess the levels of OC (B) and CTX (C) in the serum of rats with GIOP. RT-qPCR was performed to assess the expression of osteogenic differentiation-related genes (D) and bone resorption-related genes (E). N = 8, ***P < 0.001 vs. control; #P < 0.05, ##P < 0.01, ###P < 0.001 vs. Model.
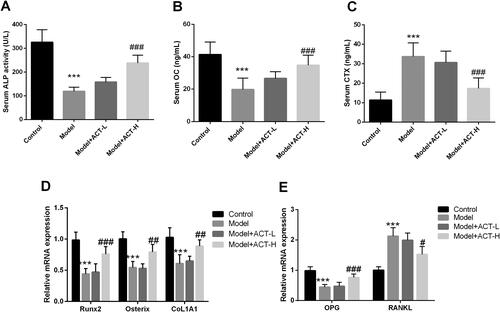
ACT restored the viability of osteoblasts and inhibited apoptosis induced by Dex
Dex-induced MC3T3-E1 Osteoblasts were used to construct an in vitro model. A significant decrease in osteoblast viability was observed with exposure to 0.1 μM and 1 μM Dex (P < 0.05, ), while cytotoxicity was only seen at a concentration of 10−5 M ACT (P < 0.05, ). Subsequently, to examine the protective effect of ACT, we treated osteoblasts exposed to 1 μM Dex with different concentrations of ACT. As shown in , ACT typically restored cell viability at concentrations of 10−7, 10−6, and 10−5 M. Therefore, 10−6 M, which has the greatest concentration of ACT and is nontoxic, was used in further experiments. Furthermore, ACT inhibited the extent of apoptosis induced by Dex (P < 0.05, ).
Figure 3. ACT significantly alleviates the effect of Dex on osteoblast activity and apoptosis. Resultst of the CCK-8 assay on Dex (A), ACT (B), and co-treatment with Dex and ACT (C) MC3T3-E1 viability in osteoblasts. D. Flow cytometry assays for the regulation of osteoblast apoptosis by ACT and Dex co-treatment. **P < 0.01, ***P < 0.001 vs. 0 group; ##P < 0.01, ###P < 0.001 vs. Dex 1 μM and 0 M ACT.
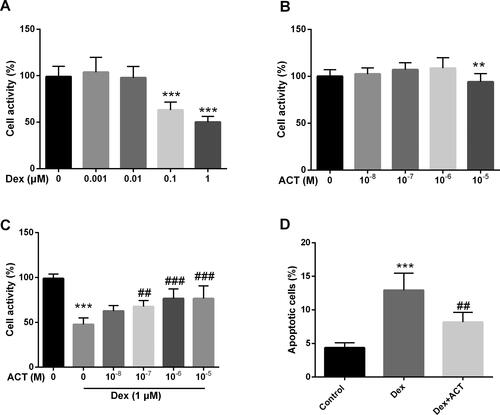
ACT exerts protective effects by activating the PI3K/AKT/mTOR signaling pathway
As shown in , ACT typically attenuated of Dex on ALP activity in MC3T3-E1 osteoblasts (P < 0.05). Simultaneously, ACT increased the levels of the osteogenic differentiation genes Runx2, osterix, and CoL1A1, and reduced the levels of the bone resorption-related genes RANKL and increased OPG levels (P < 0.05, ). Furthermore, Dex inhibited the protein levels of p-PI3K/PI3K, p-AKT/AKT and p-mTOR/mTOR in osteoblasts; however, ACT significantly restored the levels (P < 0.05, ).
Figure 4. Effects of ACT on Dex-induced bone transforming factor and key proteins of the PI3K/AKT/mTOR signaling pathway in osteoblasts. A. An ALP activity assay kit was employed to evaluate ALP levels in osteoblasts. RT-qPCR analysis of mRNA levels of genes related to osteogenic differentiation (B) and genes related to bone resorption (C). Western-blot analysis (D) and quantification of key protein levels (E) in the PI3K/AKT/mTOR signaling pathway. ***P < 0.001 vs. Control; #P < 0.05, ##P < 0.01, ###P < 0.001 vs. Dex.
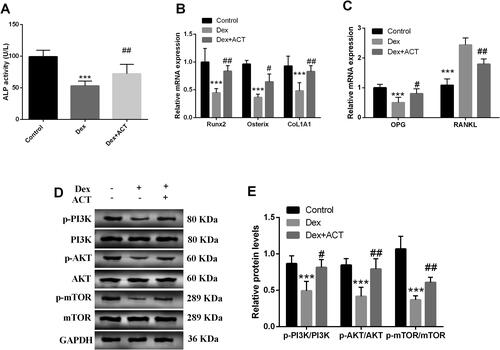
The PI3K/AKT/mTOR signaling pathway inhibitor reverses the protective effect of ACT on osteoblast activity, apoptosis, and osteogenic differentiation
The PI3K/AKT/mTOR signaling pathway inhibitor LY294002 was added to osteoblasts exposed to Dex and ACT. As shown in , LY294002 significantly reversed the levels of p-AKT/AKT, and p-mTOR/mTOR by ACT treatment compared with the Dex + ACT group (P < 0.05). LY294002 also impaired the restoration of Dex-induced osteoblast viability and the inhibition of apoptosis by ACT (P < 0.05, ). Finally, ACT alleviated the Dex-induced decrease in Runx2, osterix, COL1A1, and OPG levels, and ALP activity, as well as the increase in RANKL levels; however, this attenuation was significantly reversed by LY294002 (P < 0.05, ).
Figure 5. The PI3K inhibitor LY294002 reverses the protective effect of ACT on osteoblast activity andapoptosis. A and B. Western blot analysis of LY294002 action on the ACT-induced PI3K/AKT/mTOR signaling pathway key protein levels and quantification. The CCK-8 assay (C) and flow cytometry (D) to assess the effect of LY294002 on osteoblast activity and apoptosis. ***P < 0.001 vs. Control; #P < 0.05, ##P < 0.01, ###P < 0.001 vs. Dex, & P < 0.05 vs. Dex + ACT.
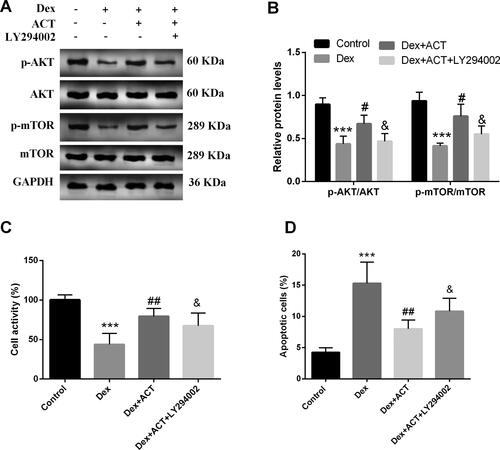
Figure 6. LY294002 reverses the regulation of osteoblast differentiation and bone resorption by ACT. An ALP activity assay kit was used to evaluate ALP levels in osteoblasts. RT-qPCR analysis of the expression of osteoblast differentiation genes and bone resorption-related genes in osteoblasts exposed to Dex and ACT with LY294002. ***P < 0.001 vs. Control; #P < 0.05, ##P < 0.01, ###P < 0.001 vs. Dex; & P < 0.05 vs. Dex + ACT.
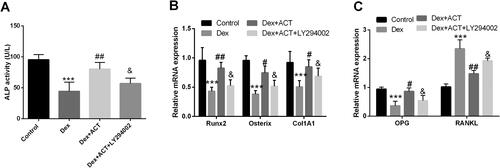
Discussion
Bisphosphonates, calcitonin, estrogen receptor modulators and parathyroid hormone (PTH) are now commonly prescribed for the management of OP; however, these agents are associated with adverse effects including gastrointestinal reactions, nephrotoxicity, hypocalcemia, and increased risk of cancer or other diseases. For example, bisphosphonates are commonly used in GIOP; however, they trigger osteoclast apoptosis and reach a bottleneck within 3–5 years of treatment and increase the risk of femur fracture [Citation30]. GCs are commonly used to treat conditions such as asthma, chronic obstructive pulmonary disease, rheumatoid arthritis, rheumatoid polyposis, and inflammatory bowel disease. However, OP is considered among the most devastating side effects of GC treatment and is the most frequent cause of secondary OP. Denosumab, a RANKL antibody that inhibits osteoclast maturation, lifespan, and activity, is approved for the treatment of GIOP. However, the occurrence of osteonecrosis of the jaw as well as rapid increases in bone remodeling is associated with an increased risk of cardiovascular events and fractures [Citation6]. The occurrence of these adverse effects has raised concerns about some active compounds derived from natural edible herbs. OP is characterized by a decrease in bone mass and deterioration of bone microarchitecture. Bone mass is lost and calcium homeostasis and bone remodeling are disrupted after GC treatment. Dex, an anabolic steroid, can induce OP in rabbits, mice, and rats. Consistent with previous studies, we created a rat model of GIOP using Dex and observed a significant decrease in BMD. We found that Dex-induced rats had typically lower BV/TV, Tb.N, and Tb.Th, and significantly higher Tb.Sp, confirming that GIOP causes severe deterioration of bone architecture. ACT ameliorated the deterioration of bone microarchitecture in the GIOP rat model and the reduction in BMD, thereby exerting a bone protective effect.
Disruption in the balance between bone formation and bone resorption plays a crucial role in GIOP. GCs have been shown to increase bone resorption and reduce osteoblast maturation. Alteration in bone activity within a short period can lead to bone loss, reduced bone strength, and increased fractures [Citation4]. OC is considered a biomarker of bone formation, and CTX is a marker of bone resorption. In our study, the level of OC was reduced and that of CTX was increased in the GIOP rat model. The findings suggest an imbalance between bone formation and bone resorption in GIOP. Maintenance of the balance requires osteoblasts for bone synthesis and osteoclasts for bone resorption. Insufficient bone formation by osteoblasts to compensate for osteoclastic bone resorption is the main cause of GIOP [Citation31]. Increasing the number and function of osteoblasts to promote bone formation appears to be a major strategy for the prevention and treatment of GIOP and has attracted the attention of many drug developers. Therefore, we selected Dex-induced osteoblasts for this study.
Osteoblast differentiation is essential for bone formation. Previous studies have demonstrated that ALP activity is a marker of osteoblast maturation and mineralization and may indirectly reflect the function of osteoblasts. Runx2 and osterix are key transcription factors for osteoblast differentiation, where Runx2 is an upstream regulatory element of osterix that activates the transcription of a series of downstream osteogenic genes and is the master switch for osteogenic factor regulation.COL1A1 is the most abundant matrix protein in bone tissue, accounting for approximately 90% of the organic matrix of bone, and a marker of bone differentiation and maturation [Citation32]. In our study, we found that Dex typically inhibited ALP activity and attenuated mRNA levels of Runx2, osterix, and COL1A1 in both in vivo rat model serum and in vitro osteoblast cell lines, suggesting that osteoblast differentiation was significantly inhibited in GIOP. In contrast, ACT significantly reversed this inhibition and restored osteogenic differentiation.
Bone resorption plays a key role in bone formation. Osteoblasts produce two key cytokines, OPG and RANKL, of which RANKL increases bone resorption by combining with the receptor RANK on osteoblasts, while OPG inhibits its receptor-related binding by binding to RANKL. Thus, an imbalance between the generation of RANKL and OPG determines the rate of bone resorption and maturation. We found a significant increase in the expression of RANKL and a significant decrease in that of OPG in both the Dex-induced OP rat model and osteoblasts exposed to Dex.The findings confirmed that GIOP increased bone resorption and decreased bone maturation. However, the increase in bone resorption was suppressed to some extent by ACT. Previous studies have reported that GC treatment reduces bone formation by increasing the apoptosis of osteoblasts and osteoclasts, which is one of the pathogenic mechanisms of GIOP [Citation33]. We found that Dex-induced apoptosis was significantly increased in osteoblasts, and treatment with ACT inhibited, the apoptosis-promoting effect of Dex. We also found that ACT significantly reduced the Dex-induced decrease in osteoblast viability.
We assessed the potential molecular mechanisms to further explore the protective role of ACT on bone formation and differentiation in GIOP Zhou et al. screened potential therapeutic targets for osteoporosis by informatics methods, and identified mTOR [Citation34]. HIF-1α regulates GIOP through the AKT/mTOR signaling pathway [Citation35]. More importantly, ACT derived from Radix Rehmanniae was shown to inhibit bone loss and structural deterioration and promote bone formation by acting on the PI3K/AKT/mTOR pathway [Citation19]. Erythrina Sativa activates autophagy via the PI3K/AKT/mTOR pathway through involvement in rat osteoblast differentiation [Citation22]. Resveratrol protects from OP by regulating the SIRT1 and PI3K/AKT/mTOR pathways [Citation25]. Therefore, we focused on the PI3K/AKT/mTOR pathway in this study. We confirmed that Dex inhibited the levels of key proteins in these signaling pathways. In contrast, ACT attenuated this inhibition, and the protective functions of ACT on osteoblast viability, apoptosis, osteogenic differentiation, and mineralization were reversed with the addition of a signaling pathway inhibitor. This study in fact has some limitations to consider when explaining the findings. Firstly, it is unfortunate that HE histology was not provided to confirm our experimental results, which was a flaw in the initial design of our experiments. Furthermore, an osteoblast-like cell line, MC3T3-E1 cells, which are also commonly used in osteoporosis, was used in this study, and we will use primary osteoblasts or bone marrow stromal cells for in vitro studies in subsequent more in-depth studies.
Taking everything into account, the present study confirmed that ACT may reduce the Dex-induced deterioration of bone microarchitecture, dysfunction of osteoblast differentiation, and apoptosis in GIOP by activating the PI3K/AKT/mTOR pathway. Our research suggests that ACT may be a potential drug candidate for the management of GIOP.
Disclosure statement
No potential conflict of interest was reported by the author(s).
Additional information
Funding
References
- Ji L, Gao J, Kong R, et al. Autophagy exerts pivotal roles in regulatory effects of 1alpha,25-(OH)2D3 on the osteoclastogenesis. Biochem Biophys Res Commun. 2019;511(4):1–9. doi:10.1016/j.bbrc.2019.02.114.
- Li N, Cornelissen D, Silverman S, et al. An updated systematic review of cost-effectiveness analyses of drugs for osteoporosis. Pharmacoeconomics. 2021;39(2):181–209. doi:10.1007/s40273-020-00965-9.
- Hu L, Liu F, Li L, et al. Effects of icariin on cell injury and glucocorticoid resistance in BEAS-2B cells exposed to cigarette smoke extract. Exp Ther Med. 2020;20(1):283–292. doi:10.3892/etm.2020.8702.
- Lane NE. Glucocorticoid-induced osteoporosis: new insights into the pathophysiology and treatments. Curr Osteoporos Rep. 2019;17(1):1–7. doi:10.1007/s11914-019-00498-x.
- Frenkel B, White W, Tuckermann J. Glucocorticoid-induced osteoporosis. Adv Exp Med Biol. 2015;872:179–215. doi:10.1007/978-1-4939-2895-8_8.
- Adhikary S, Choudhary D, Ahmad N, et al. Dietary flavonoid kaempferol inhibits glucocorticoid-induced bone loss by promoting osteoblast survival. Nutrition. 2018;53:64–76. doi:10.1016/j.nut.2017.12.003.
- Zhang JL, Qiu XM, Zhang N, et al. BuShenNingXin decoction suppresses osteoclastogenesis by modulating RANKL/OPG imbalance in the CD4+ T lymphocytes of ovariectomized mice. Int J Mol Med. 2018;42(1):299–308.
- Wong HS, Chen N, Leong PK, et al. Beta-Sitosterol enhances cellular glutathione redox cycling by reactive oxygen species generated from mitochondrial respiration: protection against oxidant injury in H9c2 cells and rat hearts. Phytother Res. 2014;28(7):999–1006. doi:10.1002/ptr.5087.
- Chen Y, Li YQ, Fang JY, et al. Establishment of the concurrent experimental model of osteoporosis combined with Alzheimer’s disease in rat and the dual-effects of echinacoside and acteoside from Cistanche tubulosa. J Ethnopharmacol. 2020;257:112834. doi:10.1016/j.jep.2020.112834.
- Pu X, Song Z, Li Y, et al. Acteoside from Cistanche salsa inhibits apoptosis by 1-methyl-4-phenylpyridinium ion in cerebellar granule neurons. Planta Med. 2003;69(1):65–66. doi:10.1055/s-2003-37029.
- Fu Z, Fan X, Wang X, et al. Cistanches Herba: an overview of its chemistry, pharmacology, and pharmacokinetics property. J Ethnopharmacol. 2018;219:233–247. doi:10.1016/j.jep.2017.10.015.
- Lai X, Xiong Y, Zhou J, et al. Verbascoside attenuates acute inflammatory injury in experimental cerebral hemorrhage by suppressing TLR4. Biochem Biophys Res Commun. 2019;519(4):721–726. doi:10.1016/j.bbrc.2019.09.057.
- Ji SL, Cao KK, Zhao XX, et al. Antioxidant activity of phenylethanoid glycosides on glutamate-induced neurotoxicity. Biosci Biotechnol Biochem. 2019;83(11):2016–2026. doi:10.1080/09168451.2019.1637243.
- Morikawa T, Ninomiya K, Imamura M, et al. Acylated phenylethanoid glycosides, echinacoside and acteoside from Cistanche tubulosa, improve glucose tolerance in mice. J Nat Med. 2014;68(3):561–566. doi:10.1007/s11418-014-0837-9.
- Wu X, He W, Zhang H, et al. Acteoside: a lipase inhibitor from the Chinese tea Ligustrum purpurascens kudingcha. Food Chem. 2014;142:306–310. doi:10.1016/j.foodchem.2013.07.071.
- Lim H, Kim DK, Kim TH, et al. Acteoside counteracts interleukin-1beta-induced catabolic processes through the modulation of mitogen-activated protein kinases and the NFkappaB cellular signaling pathway. Oxid Med Cell Longev. 2021;2021:8684725. doi:10.1155/2021/8684725.
- Cui Q, Pan Y, Zhang W, et al. Metabolites of dietary acteoside: profiles, isolation, identification, and hepatoprotective capacities. J Agric Food Chem. 2018;66(11):2660–2668. doi:10.1021/acs.jafc.7b04650.
- Yang L, Zhang B, Liu J, et al. Protective effect of acteoside on ovariectomy-induced bone loss in mice. Int J Mol Sci. 2019;20(12):2974.
- Gong W, Zhang N, Cheng G, et al. Rehmannia glutinosa libosch extracts prevent bone loss and architectural deterioration and enhance osteoblastic bone formation by regulating the IGF-1/PI3K/mTOR pathway in streptozotocin-induced diabetic rats. Int J Mol Sci. 2019;20(16):3964.
- Wang XY, Gong LJ, Huang JM, et al. Pinocembrin alleviates glucocorticoid-induced apoptosis by activating autophagy via suppressing the PI3K/Akt/mTOR pathway in osteocytes. Eur J Pharmacol. 2020;880:173212. doi:10.1016/j.ejphar.2020.173212.
- Ge X, Zhou G. Protective effects of naringin on glucocorticoid-induced osteoporosis through regulating the PI3K/Akt/mTOR signaling pathway. Am J Transl Res. 2021;13(6):6330–6341.
- Zhao B, Peng Q, Poon EHL, et al. Leonurine promotes the osteoblast differentiation of rat BMSCs by activation of autophagy via the PI3K/Akt/mTOR pathway. Front Bioeng Biotechnol. 2021;9:615191.
- Zhou H, Jiao G, Dong M, et al. Orthosilicic acid accelerates bone formation in human osteoblast-like cells through the PI3K-Akt-mTOR pathway. Biol Trace Elem Res. 2019;190(2):327–335. doi:10.1007/s12011-018-1574-9.
- Tang Y, Mo Y, Xin D, et al. Regulation of osteoblast autophagy based on PI3K/AKT/mTOR signaling pathway study on the effect of beta-ecdysterone on fracture healing. J Orthop Surg Res. 2021;16(1):719. doi:10.1186/s13018-021-02862-z.
- Yang X, Jiang T, Wang Y, et al. The role and mechanism of SIRT1 in resveratrol-regulated osteoblast autophagy in osteoporosis rats. Sci Rep. 2019;9(1):18424. doi:10.1038/s41598-019-44766-3.
- Cheong Y, Kim M, Kim N, et al. Effect of two-week continuous epidural administration of 2% lidocaine on mechanical allodynia induced by spinal nerve ligation in rats. Anesth Pain Med (Seoul). 2020;15(3):334–343. doi:10.17085/apm.20033.
- Montazeri-Najafabady N, Ghasemi Y, Dabbaghmanesh MH, et al. Exploring the bone sparing effects of postbiotics in the post-menopausal rat model. BMC Complement Med Ther. 2021;21(1):155. doi:10.1186/s12906-021-03327-w.
- Han H, Xiao H, Wu Z, et al. The miR-98-3p/JAG1/Notch1 axis mediates the multigenerational inheritance of osteopenia caused by maternal dexamethasone exposure in female rat offspring. Exp Mol Med. 2022;54(3):298–308. doi:10.1038/s12276-022-00743-x.
- Xie B, Zeng Z, Liao S, et al. Kaempferol ameliorates the inhibitory activity of dexamethasone in the osteogenesis of MC3T3-E1 cells by JNK and p38-MAPK pathways. Front Pharmacol. 2021;12:739326.
- Han Y, Jin Y, Lee SH, et al. Berberine bioisostere Q8 compound stimulates osteoblast differentiation and function in vitro. Pharmacol Res. 2017;119:463–475. doi:10.1016/j.phrs.2017.03.002.
- Jiang SD, Yang YH, Chen JW, et al. Isolated osteoblasts from spinal cord-injured rats respond less to mechanical loading as compared with those from hindlimb-immobilized rats. J Spinal Cord Med. 2013;36(3):220–224. doi:10.1179/2045772312Y.0000000071.
- Fan YS, Li Q, Hamdan N, et al. Tetrahydroxystilbene glucoside regulates proliferation, differentiation, and OPG/RANKL/M-CSF Expression in MC3T3-E1 cells via the PI3K/Akt pathway. Molecules. 2018;23(9):2306. doi:10.3390/molecules23092306.
- Yanbeiy ZA, Hansen KE. Denosumab in the treatment of glucocorticoid-induced osteoporosis: a systematic review and meta-analysis. DDDT. 2019;ume 13:2843–2852. doi:10.2147/DDDT.S148654.
- Zhou HN, Li HY, Xu WH, et al. Study on the action mechanism of wuling powder on treating osteoporosis based on network pharmacology. Chin J Nat Med. 2021;19(1):28–35. doi:10.1016/S1875-5364(21)60003-1.
- Xu WN, Zheng HL, Yang RZ, et al. HIF-1alpha regulates glucocorticoid-induced osteoporosis through PDK1/AKT/mTOR signaling pathway. Front Endocrinol (Lausanne). 2019;10:922.