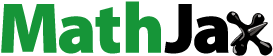
Abstract
Background: Sepsis-induced cardiomyopathy (SIC) is a severe myocardial dysfunction secondary to septicemia. It is a major concern owing to the high mortality and morbidity, which are greatly influenced by ferroptosis. Resveratrol (RSV) is a naturally existing agonist of the silent information regulator 1 (Sirt1). It has cardioprotective effects against sepsis-induced myocardial injury, However, the detailed mechanism is unknown.
Methods: In this study, cecal ligation and puncture (CLP)-induced septic rats were employed to assess the changes in ferroptosis with RSV administration. According to the different treatments the rats were divided into the following groups: (1) the Sham, (2) CLP, (3) CLP + RSV at various doses (10, 30, and 50 mg/kg), and (4) CLP + Fer-1(a ferroptotic inhibitor) groups. After 24 h, the structure and function of the cardiac system in rats were evaluated, and mitochondrial morphology, ferroptosis-related biomarkers, and the levels of Sirt1/Nrf2 were assessed.
Results: The rats that underwent CLP had suffered cardiac dysfunction, accompanied with myocardial damage, impaired mitochondria, elevated lipid peroxidation, and reduced Sirt1/Nrf2 expression in the myocardium. High-dose RSV successfully improved heart function, reversing the abnormalities in a dose-dependent manner. We then used EX527, a selective Sirt1 inhibitor, to further identify the intermediate signaling targets of RSV that regulate ferroptosis. EX527 diminished the curative effects of high-doses RSV.
Conclusions: Summarily, our findings suggest a novel mechanism of RSV in reducing SIC: ferroptosis inhibition via upregulation of Sirt1/Nrf2 signaling pathways. This may be an effective therapeutic approach against organ failure in sepsis, particularly SIC.
Introduction
Sepsis is a life-threatening organ malfunction caused by a dysregulated host response to infection [Citation1]. It is a major healthcare problem, as recognized by the World Health Organization as a major healthcare problem. Sepsis-induced cardiomyopathy (SIC) is a common complication of severe sepsis and septic shock, resulting in decreased left ventricular dilatation and impaired ejection fraction, which is associated with a high mortality [Citation2,Citation3]. Ferroptosis occurs in numerous cardiac pathologies, including diabetes-related cardiopathies, doxorubicin-induced cardiotoxicity, iron overload heart failure, and septic cardiomyopathy, according to an increasing body of research [Citation4–7]. However, the specific mechanism of cardiac damage by ferroptosis remains unknown.
Ferroptosis differs with other forms of regulated cell death in genetic, biochemical, and morphological aspects [Citation8]. The extent of ferroptosis is a reflection of the peroxidation of polyunsaturated fatty acids (PUFAs), the lack of antioxidant ability, and cellular buildup of free iron ions- these are characteristic features of ferroptosis [Citation9,Citation10]. Acyl-CoA synthetase long-chain family member 4 (ACSL4) and glutathione peroxidase 4 (GPX4) can regulate PUFAs metabolism and biosynthesis of glutathione (GSH), respectively. These proteins play a role in the biobehavioral processes regulating ferroptosis [Citation11]. In addition, transferrin receptor (TFR) and ferritin heavy chain-1 (FTH-1) are involved in intracellular transport, metabolism and storage of iron ions. Therefore, their disorders can largely contribute to the dysregulation of iron pool homeostasis, ultimately promoting buildup of reactive oxygen species (ROS) from the Fenton reaction [Citation9,Citation12]. Vulnerable lipid membranes with phospholipids react with excess intracellular ROS to produce lipid peroxides, such as malondialdehyde (MDA) and 4-hydroxynonenal (4-HNE), which inactivate key structural and functional proteins within cells [Citation13,Citation14]. Ferrostatin-1 (Fer-1) is a potent ferroptotic inhibitor: it performs this role through screening of massive molecules and inhibition of ROS accumulation [Citation8]. Li et al. created a sepsis model in mice by intraperitoneal injection of lipopolysaccharides (LPS) and found that Fer-1, and dexrazoxane, an iron chelator, reduced myocardial ferroptosis in septic mice [Citation5]. The underlying mechanism of myocardial dysfunction requires further study.
Resveratrol (3, 5, 4′-trans-trihydroxystilbene; RSV), a natural activator of silent information regulator 1 (Sirt1), are present in grapes, berries, and red wine. It is renowned for its antioxidant and anti-inflammatory characteristics [Citation15,Citation16]. Regarding its role in combating various pathological states, RSV is a potent antioxidant. Moreover, in vivo and in vitro, it decreases CLP-induced oxidative damage and lipid peroxidation. This is most likely via activation of the Sirt1-mediated pathway, which results in activation of the antioxidant system [Citation17–21]. Increasing evidence has shown that low or high Sirt1 expression can affect disease states or physiological functions by affecting nuclear factor erythroid 2-related factor 2 (Nrf2) [Citation22–24], which results in regulation of ferroptosis. By influencing the iron, lipid, and glutathione equilibrium downstream, Nrf2 signaling is connected to several molecular features of ferroptosis [Citation12,Citation13,Citation25,Citation26], serving as a buffer against cardiac damage. Therefore, the Sirt1/Nrf2 signaling pathway may be important in several biological processes.
It has been reported that the application of RSV has a protective effect in sepsis-induced cardiac dysfunction [Citation27,Citation28]. However, its specific protective mechanism in sepsis remains unclear. Herein, we explored the association between RVS and myocardial injury in sepsis and further investigated the role of ferroptosis in sepsis-induced cardiomyopathy via increased Sirt1/Nrf2 expression with RSV treatment. This study may offer a novel approach to preventing and treating sepsis-induced cardiomyopathy.
Materials and methods
Materials and animals
RSV () was purchased from Macklin Biochemical Technology Co. (Shanghai, China). Fer-1 and the specific Sirt1 inhibitor EX527, (6-chloro-2, 3, 4, 9-tetrahydro-1H-carbazole-1-carboxamide), was purchased from Rhawn Reagent Co. (Shanghai, China). The drug was dissolved in DMSO and diluted with 0.9% saline to the desired concentration.
Sprague–Dawley male rats (6–8 weeks old and weighing 180–220 g) were procured from the Animal Experiment Center of Xinjiang Medical University (Urumqi, China). The National Institutes of Health’s guide for the care and use of laboratory animals was followed when conducting the study (NIH Publications No. 8023, revised 1978). The rats were fed in individual cages with 12-hour light-dark cycles and were acclimated for one week before the experiment. This research was authorized by the Shihezi University Institutional Animal Care and Use Committee and the Shihezi Research Institute of Animal Experiments.
Cecal ligation and puncture
Cecal ligation and puncture (CLP) were performed in accordance with the guidelines [Citation29]. The rats were fixed in a supine position after general anesthesia. The skin was prepared and disinfected, and a mid-line abdominal incision of 1–1.5 cm in length was created. The cecum was moved out of the abdominal cavity, ligated with a No. 4 silk thread 1/4 from the root, and stabbed twice with an 18-gauge needle through the intestinal wall. Based on previous experience, after closing the abdomen layer by layer, we administered 40 mL/kg of sodium lactate Ringer solution subcutaneously to the rats immediately after surgery. The rats in the sham-operated group were given the same treatment but without CLP. After surgery the rats were transferred to their cages. Food intake was prohibited, and water was made available.
Experimental protocol
The rats were then separated at random into seven groups:
In the Sham group, the rats underwent laparotomy without CLP procedures.
In the CLP group, the rats underwent CLP after 30 minutes of receiving vehicle (2 mL).
In the low RSV group, the rats underwent to CLP after 30 minutes of receiving RSV (2 mL, 10 mg/kg).
In the medium RSV group, the rats underwent to CLP after 30 minutes of receiving RSV (2 mL, 30 mg/kg).
In the high RSV group, the rats underwent CLP after 30 minutes of receiving RSV (2 mL, 50 mg/kg).
In the Fer-1 group, the rats underwent CLP after 30 minutes of receiving Fer-1 (2 mL, 5 mg/kg).
In the RSV + EX527 group, the rats underwent to CLP after 30 minutes of receiving RSV (2 mL, 50 mg/kg) and EX527 (2 mL, 5 mg/kg).
All the rats experienced tachypnea, tachycardia, depression, erect hair, huddling, decreased food intake, and reduced activity after CLP modeling. Twenty-four hours after surgery, echocardiography was used to evaluate the cardiac function of each group of rats. The rats were anesthetized with sodium pentobarbital and euthanized by cervical dislocation when they were immobile, unresponsive and had diarrhea or urinary incontinence, abdominal infection or septicemia, or reached the end of the observation timeline, i.e. 24 h after surgery. Death was confirmed by cardiac and respiratory arrest, muscle relaxation, and lack of reflexes. 4 mL of abdominal aortic blood was collected during anesthesia, was centrifuged for 15 min (3000 r/min), and the obtained serum was kept in a refrigerator at −80 °C for subsequent testing. The heart tissue was obtained immediately after rat euthanization, and part was placed in 4% paraformaldehyde. The remaining tissue was stored at −80 °C for other experiments.
Cell culture
Procell Life Science & Technology Co. Ltd. provided H9c2 rat cardiomyocytes for this study (Wuhan, China). The cells were grown at 37 °C and 5% CO2 in flasks containing 90% Dulbecco’s Modified Eagle medium (DMEM; Gibco, Thermo Fisher Scientific, USA), 10% fetal bovine serum (Thermo Fisher Scientific, USA), and 1% 10,000 U/mL penicillin/streptomycin (Thermo Fisher Scientific, USA). The cells were then pretreated with RSV (1, 10, 25, 50, 75, and 100 M) for 1 hour at 37 °C before being stimulated with LPS (Sigma, USA; 1, 3, 5, 10 and 20 µg/mL) for 24 hours at 37 °C. The H9c2 cells were separated into three groups: the control, LPS, and LPS + RSV groups.
CCK-8 assay
To determine cell viability, the CCK-8 kit (Dojindo, Japan) was used. In a 96-well plate, the H9c2 cells were seeded and administered LPS and RSV. After treatment, 10 µL of the CCK-8 solution was added to each well, and the plates underwent 2-hour incubation at 37 °C. The absorbance of the microplate reader (Bio-Rad, USA.) was set at 450 nm.
Detection of reactive oxygen species (ROS)
The level of intracellular ROS was evaluated by measuring the fluorescence of DCFH-DA (Solarbio, China). After cultivation in a 6-well plate and three PBS washes, the H9c2 cells were incubated with 10 M DCFH DA for 30 min at room temperature in the dark. Photos were taken using a fluorescence microscope at the excitation/emission wavelength of 504/529 nm (Olympus, Japan).
Mitochondrial Membrane potential (MMP) assay
MMP is a key indicator of mitochondrial function assessment. Staining with 5,5′,6,6′-tetrachloro-1,1′,3,3′-tetraethylbenzimidazolylcarbocyanine iodide (JC-1; Solarbio, China) was performed to analyze the MMP changes. The H9c2 cells were treated with RSV, stained with 10 μM JC-1 for 30 min at room temperature in the dark, and observed by the fluorescence microscope (Olympus, Japan). The red/green fluorescence ratio calculated using Image-Pro Plus 6.0 was used to detect the mitochondrial function of cells.
Echocardiography
Cardiac function assessment of each rat group was completed using echocardiography. The rats were anesthetized at the 24th hour after CLP, and cardiac ultrasonography was performed immediately after skin preparation. To take M-mode echocardiographic measurements, an ultrasound machine (PHILIPS EPIQ7C, USA) with a 15-MHz transducer probe was employed. The left ventricular end diastolic volume (LVEDV), left ventricular end systolic volume (LVESV), as well as the left ventricular internal dimension diastole (LVIDd) and systole (LVIDs) were measured. Additionally, the following algorithms were used to compute the markers of left ventricular systolic function, left ventricular ejection fraction (LVEF) and left ventricular shortening fraction (LVFS):
Histopathology analysis
The hearts that were placed in 4% paraformaldehyde were removed, embedded in paraffin, and cut into 4 μm sections. The tissue sections were deparaffinized and rehydrated before being stained with hematoxylin and eosin (H&E). The histological images were viewed at 200x magnification with a microscope (Olympus, Tokyo, Japan). The histological scoring was as follows: 0, no myocardial lesion; +1, lesions involving 25% of the myocardium; +2, lesions involving 25% to 50% of the myocardium; +3, lesions involving 50% to 75% of the myocardium; and +4, lesions involving 75% of the myocardium (Kishimoto scores) [Citation30]. Five portions from each group were examined.
Transmission electron microscopy of mitochondria
The samples of left ventricular myocardium were quickly removed (2 × 2 × 2mm) and the samples were preserved in a 0.1 M phosphate buffer with 4% paraformaldehyde and 2.5% glutaraldehyde for 2 hours. Afterwards, the tissues were dehydrated, embedded in 1% agarose, and sectioned into ultrathin (70 nm) slices using an ultramicrotome (Leica EM UC7, Germany). Sections were stained for 15 minutes with uranyl acetate in pure ethanol, then for 15 minutes with lead citrate. At least five images per group were captured using a transmission electron microscope (HITACHI HT7700, Tokyo, Japan) operating at 80 kV. The severity of mitochondrial damage was graded using the Flameng scores [Citation31]. For each microstructure image, grades ranging from 0 to 4 were assigned based on the severity of mitochondrial damage.
Immunohistochemical staining
Sections were deparaffinized, rehydrated, and subjected to heat-induced epitope extraction before being blocked with 10% goat serum for 10 minutes at room temperature. Immunohistochemical staining was performed using Nrf2 antibodies (Abcam, Cambridge, USA) incubated overnight at 4 °C. After that, immunoreactivity was assessed using an HRP-DAB system (Servicebio, Wuhan, China), and hematoxylin re-staining was performed. As a benchmark, goat anti-rabbit immunoglobulin G (IgG) was used. For microscopy, these sections were photographed under light (Olympus, Tokyo, Japan). Five pictures were taken randomly in a bright area. The image analysis program Image-Pro Plus 6.0 was used to quantify the images.
Immunofluorescent staining
Rat myocardial tissues were cut into frozen slices, thawed at room temperature for 0.5 hours, incubated for 20 minutes with 0.1% Triton X-100, and then washed three times with PBS for 5 minutes each time. The sections were pre-incubated with 4-HNE (1:100, Abcam, Cambridge, USA) primary antibodies overnight at 4 °C after being blocked with 10% goat serum for 10 minutes at room temperature. The following morning, the sections were incubated for 2 hours at room temperature with Alexa Fluor 594 goat anti-mouse IgG secondary antibodies (1:250, Solarbio, Beijing, China). Finally, DAPI was incubated with the slices for 30 minutes at 4 °C. Finally, a fluorescent microscope was used to capture the photographs (Olympus, Tokyo, Japan). The fluorescence intensity was evaluated using Image-Pro Plus 6.0.
Lactate dehydrogenase and creatine kinase isoenzyme assay
Lactate dehydrogenase (LDH) and creatine kinase isoenzyme (CK-MB) were measured using the LDH (Jiancheng, Nanjing, China) and CK-MB assay kits (Jiancheng, Nanjing, China). An appropriate amount of serum from centrifuged abdominal aortic blood was used to detect changes in the LDH and CK-MB levels. The concentrations of both were calculated using a the standard curve.
MDA assay
A thiobarbituric acid reactive substance (TBARS) Parameter Kit (Solarbio, Beijing, China) was used to measure the MDA concentration for oxidative stress. For each group, 100 mg of hearts were sliced and promptly homogenized with cold saline. The supernatant was collected to assess the MDA concentrations after a 10-minute period of centrifugation. All the reagents were prepared according to the instructions of the manufacturer. To determine the MDA concentration, absorbance was measured at wavelengths of 532 and 600 nm.
Iron and GSH assay
The method described above was used to homogenize heart tissues, and centrifugation was used to obtain the supernatant. The iron assay kit (Applygen, Beijing, China) and micro reduced glutathione GSH Assay Kit (Jiancheng, Nanjing, China) were used to measure the levels of iron and GSH. The absorbance of iron and the GSH assay kit were read at wavelengths of 550 and 405 nm, respectively. The concentrations of both were calculated using a standard curve.
Western blotting analysis
Myocardial tissues were homogenized on ice and lysed by adding RIPA tissue lysis solution for 30 min. The supernatant was collected after centrifugation at 12000 r/min. After adding the load buffer, gel electrophoresis was performed. An 8–12% SDS–PAGE gel was used to load ten micrograms of proteins, which was then transferred to polyvinylidene fluoride membranes. After blocking in a 5% BSA solution for 2 hours at room temperature, the polyvinylidene fluoride membranes were incubated overnight at 4 °C with primary antibodies (1:1000) against Sirt1 (Abcam, Cambridge, USA), Nrf2 (Abmart, Shanghai, China), GPX4 (Abmart, Shanghai, China), ACSL-4 (Abcam, Cambridge, USA), TFR (Abmart, Shanghai, China), FTH-1 (Abcam, Cambridge, USA) and β-actin (Zsbio, Beijing, China), following incubation with the corresponding isotype-matched secondary antibody (1:10000) for 1 h at room temperature. The membrane was developed by adding ECL luminescent solution dropwise. Finally, we used image J software to quantify the immunoblots.
Statistical analysis
Standard deviation (SD) format performed using Prism 8.0 software (GraphPad, CA, USA).Paired-samples t test and one way analysis of variance (ANOVA) followed by a post hoc (Bonferroni) test were used to compare the groups after homoscedasticity was confirmed. The level of statistical significance was at p < 0.05.
Results
RSV ameliorates cardiac pathological damage in rat with sepsis
As shown in , to assess whether RSV ameliorates cardiac pathological damages in septic rats, prior to CLP at low, medium, and high RSV doses (10, 30, and 50 mg/kg, respectively) were administered at intervals of 30 minutes. Both LDH and CK-MB are significant biomarkers of myocardial damage after SIC. In the CLP group, myocardial enzyme release was considerably higher in the Sham group, and heart function was severely impaired. In contrast, the release of myocardial enzymes was lower in the RSV groups than in the CLP groups (). H&E staining of the heart samples of the CLP group had a greater extent of inflammatory cell infiltration and anatomical tissue distortion conformation, including lymphocytic infiltration and flaky degeneration of cardiomyocytes, than the Sham group (). The natural architecture of the myocardium was well protected with the administration of Fer-1 and high-dose RSV, while low and medium dosages of RSV were ineffective. These results indicated that high doses of RSV were most effective in preventing CLP-induced cardiac dysfunction at the doses tested.
Figure 2. Protective effect of resveratrol on myocardium in septic rats. (A) Experimental process. Drugs were injected intraperitoneally 30 min prior to CLP. (B)-(C) The effect of a range doses of RSV on the serum CK-MB and LDH levels in rats (n = 5 per group). (D) The representative images of H&E staining of myocardium (Kishimoto scores), n = 5 per group. CLP induced blurring or rupture of myocardium concomitant with lymphocytic infiltration and erythrocytic congestion (red arrows), scar bar = 50 μm. Sham: Sham group, CLP: CLP group, L-RSV: CLP + low-dose (10mg/kg) RSV group, M-RSV: CLP + medium-dose (30mg/kg) RSV group, H-RSV: CLP + high-dose (50mg/kg) RSV group, Fer-1: CLP + Fer-1 (5mg/kg) group. Data are expressed as mean ± SD. *p < 0.05 versus Sham. #p < 0.05 versus CLP.
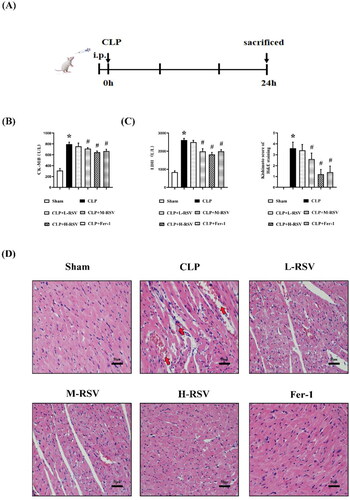
RSV improves cardiac function in rats with sepsis
To assess whether RSV can improve cardiac dysfunction in sepsis, we measured the cardiac function of rats using echocardiography 24 hours after CLP. As shown in , LVEF and LVFS, the major indexes of cardiac function, were decreased in the CLP group: these decreases were significantly inhibited by high doses of RSV. Moreover, the LVEDV, LVESV and LVIDs were increased in the CLP group, and these changes were significantly inhibited by high doses of RSV. Also, Fer-1 attenuated all alterations brought by CLP. However, no significant difference in LVIDd was observed among the six groups (). These results revealed that RSV can improve CLP-induced cardiac dysfunction in a dose-dependent manner.
Figure 3. RSV attenuated CLP-induced cardiac dysfunction. (A) The representative M-mode images of echocardiography for each group, n = 4 per group. (B) Statistical analysis of cardiac function indexes LVEF, n = 4 per group. (C) Statistical analysis of cardiac function indexes LVFS. n = 4 per group. (D) Statistical analysis of LVIDd, n = 4 per group. (E) Statistical analysis of LVIDs. n = 4 per group. (F) Statistical analysis of LVEDV, n = 4 per group. (G) Statistical analysis of LVESV, n = 4 per group.
Data are expressed as mean ± SD. *p < 0.05 versus Sham. #p < 0.05 versus CLP. &p < 0.05 versus CLP + H-RSV.
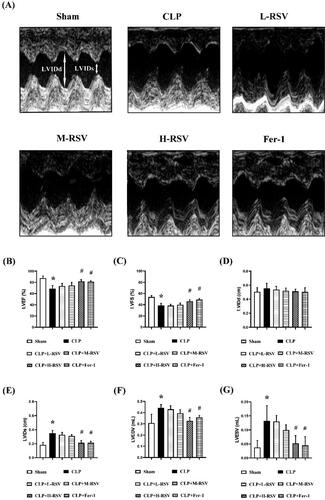
RSV mitigates mitochondrial damage and septic cardiac ferroptosis
Mitochondrial dysfunction is a pivotal contributor to septic cardiomyopathy and an important indicator of myocardial ferroptosis. Herein, we measured the extent of mitochondrial damage scores in septic myocardium using the Flameng scores. CLP perturbed the mitochondrial structure and reduced the mitochondrial membrane integrity and cristae density in SD rats. However, RSV reduced mitochondrial impairment in a dose-dependent manner compared to CLP controls. Pretreatment with Fer-1 had similar results with RSV ().
Figure 4. Effect of resveratrol on mitochondria and ferroptosis in the myocardium of septic rats. (A) The representative transmission electron microscopic images of heart at 24 hours after CLP (Flameng scores), scar bar = 2 μm, n = 5 per group. The morphological features of ferroptosis appear as shrunken mitochondria with increased membrane density or outer membrane rupture (red arrows). (B)–(C) Relative protein levels of GPX4 and ACSL4, n = 3 per group. (D) The concentrations of iron in the myocardium tissues, n = 5 per group. (E) The concentrations of MDA in the myocardium tissues, n = 5 per group. Data are expressed as mean ± SD. *p < 0.05 versus Sham. #p < 0.05 versus CLP.
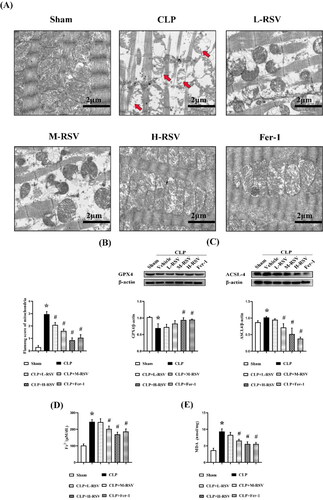
The roles of GPX4 and ASCL4 have critical implications in ferroptosis sensitivity. GPX4 transforms reduced GSH to oxidized GSSG and reduces excess oxidized lipids by the involvement of ROS in the reaction [Citation32], ASCL4, a the positive regulator of ferroptosis, activates long-chain fatty acids for lipid synthesis, which in turn increases cellular ferroptotic susceptibility [Citation33]. Western blotting revealed that GPX4 expression was considerably lower in the CLP group than in the Sham group, and administration of all three doses of RSV elevated its level: the extent of increase increased with increasing RSV dose (). In contrast, ACSL4 expression had opposite result to those of GPX4 (). The CLP group had a 50% higher iron concentration and 60% higher MDA levels than the Sham group (). Furthermore, all the aforementioned ferroptotic alterations were restored after treatment with the ferroptotic inhibitor Fer-1.
RSV decreases sepsis-induced oxidative stress and mitochondrial damage in cardiomyocytes
The effects of LPS and RSV on cell viability were assessed to determine the appropriate dose (). When treated with 5 µg/mL LPS, significant improvement in cell viability was observed with RSV. However, the effects of RSV were independent of concentration. Cell viability was decreased when RSV concentration was ≥50 µM. RSV at 25 µM produced the greatest treatment effect; therefore, it was used in subsequent tests.
Figure 5. Effect of resveratrol on oxidative stress and mitochondrial function in H9c2 cells. (A) Viability of H9c2 cells after LPS stimulation under different concentrations (0, 1, 3, 5, 10 and 20 µg/mL), n = 3 per group. (B) Viability of H9c2 cells after RSV treatment for 24 h (1, 10, 25, 75, and 100 μM), n = 3 per group. (C) ROS in H9c2 cells with 5 µg/mL LPS and 25 µM RSV, n = 3 per group. (D) The changes of MMP were measured by JC-1 probes, n = 3 per group. Data are expressed as mean ± SD. *p < 0.05 versus control. #p < 0.05 versus LPS.
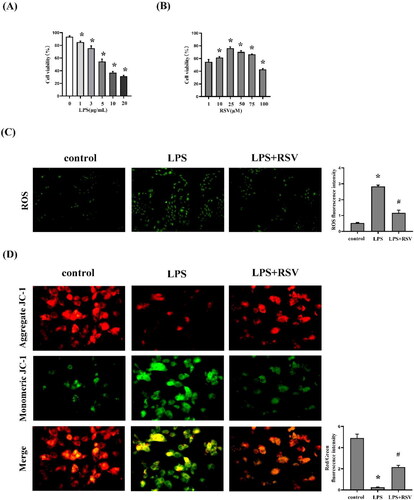
In the following study, we found that LPS stimulation led to an imbalance between oxidative and antioxidant effects in H9c2 cells and treatment with RSV significantly reversed this phenomenon. MMP is a key indicator of mitochondrial function assessment. The decreased mitochondrial JC-1 polymer (red fluorescence) and increased JC-1 monomer (green fluorescence) in the LPS group demonstrated that the MMP decreased, which suggested mitochondrial dysfunction. The red/green ratio was significantly higher in the RSV group than in the LPS group; the RSV group had an increased MMP level (). These data indicated that RSV protected the cardiomyocytes from oxidative stress injury and mitochondrial dysfunction.
Blockade of the Sirt1 signaling pathway reduces the protective effect of RSV in septic cardiomyopathy
Then, we investigated the effect of EX527, a selective Sirt1 inhibitor, on the cardioprotective effects of RSV in rats that underwent CLP to further reveal the intermediate signaling targets of RSV regulation of ferroptosis. As shown in , EX527 reduced the cardioprotective effects of RSV. The LVEF and LVFS that increased following RSV were reduced by EX527 (). In addition, CK-MB and LDH levels that were increased by RSV were decreased by EX527 (). Furthermore, EX527 aggravated myocardial structure damage (). These results suggested that Blockade of the Sirt1 signaling pathway reduces the protective effect of RSV in septic cardiomyopathy.
Figure 6. EX527 pretreatment suppressed the cardioprotective effects of RSV and augmented myocardial damage. (A) The representative M-mode images of echocardiography for each group, statistical analysis of cardiac function indexes LVEF and LVFS, n = 4 per group. (B)-(C)the serum CK-MB and LDH levels in rats, n = 4 per group.(D) The representative images of H&E staining of myocardium (Kishimoto scores), n = 4 per group. Sham: Sham group, CLP: CLP group, H-RSV: CLP + high-dose (50mg/kg) RSV group, H-RSV + EX527: CLP + H-RSV + EX527 (5mg/kg) group. Data are expressed as mean ± SD. *p < 0.05 versus Sham. #p < 0.05 versus CLP. &p < 0.05 versus CLP + H-RSV.
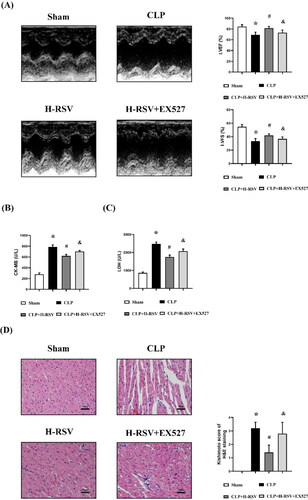
The upregulation of Sirt1 leads to an increase in Nrf2 protein level in rats with SIC
A molecular docking analysis provided a visual explanation of the interaction between RSV and its potential interactions associated with Sirt1. We used Chem3D software to draw the 3 D structure of resveratrol and exported it in the mol*2 format. The protein structure of Sirt1 (PDB ID: 5BTR) was downloaded from the RCSB Protein Data Bank (http://www.pdb.org/) and modified using Autodock 1.5.6 software. Images were generated using Pymol. The binding energy of the ligand and receptor was calculated by running the Vina script. Their docking energies were below −5.0 kcal/mol, indicating that they could form a stable dock. Furthermore, hydrogen bonding and hydrophobic function were the main forms of interaction between RSV and Sirt1. These interactions offered rationale for the possibility that RSV regulates Sirt1 ().
Figure 7. Effect of resveratrol on the activation of Sirt1/Nrf2 signaling pathway in sepsis-induced cardiomyopathy. (A) Molecular docking of RSV to Sirt1. Binding mode between RSV and Sirt1 (Left) and detailed view of SIRT1 (Right). (B)-(C) Relative protein levels of Sirt1 and Nrf2, n = 3 per group. (D) The representative images of immunohistochemical staining using heart tissues with anti-Nrf2, scar bar = 50 μm, n = 5 per group. Data are expressed as mean ± SD. *p < 0.05 versus Sham. #p < 0.05 versus CLP. &p < 0.05 versus CLP + H-RSV.
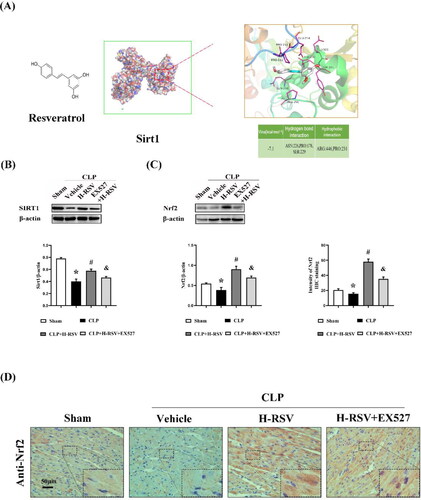
The contribution of Nrf2 in the maintenance of proper oxidation-reduction homeostasis is well established. In turn, it is pivotal in mediating other critical biometabolic pathways, which include the metabolism of iron/hemoglobin, carbohydrates, and lipids metabolism [Citation13]. In the CLP group, we identified a considerable reduction in Sirt1 and Nrf2 expression in the heart (). Along the same line, the immunohistochemical staining analysis also demonstrated that high-dose RSV could significantly increase the expression of Nrf2 after CLP (), yet the Sirt1 inhibitor (Ex527) eliminated the protective effect of H-RSV. These results suggested that the upregulation of Sirt1 induced an elevation in the Nrf2 level in rats with SIC.
Activation of the Sirt1/Nrf2 pathway with RSV alleviates ferroptosis in rats with SIC
Considering the powerful antioxidant effects of Nrf2 in ferroptosis have been studied in numerous previous researches. To identify the potential regulatory mechanisms and mode of functioning of RSV, we conducted experiments to quantify the pivotal regulatory proteins related to ferroptosis, status of iron metabolism, or aldehyde products of lipid peroxidation—MDA and 4-HNE—after being subjected to different oxidative stress conditions with or without RSV. In our experiments, we investigated the possibility that RSV could alleviate lipid peroxidation associated with ferroptosis and normalize iron metabolic homeostasis after CLP. As the results indicated, GPX4 and FTH-1 expressions were markedly higher, and ACSL4 and TFR expressions were lower in myocardial tissue in the H-RSV group than in the Sham group (). Subsequently, known as an important component of the antioxidant system, the concentrations of reduced GSH were also markedly low in the CLP group ().Accordingly, higher levels of MDA,4-HNE, and iron were also detected in the CLP group (). Treatment with RSV alleviated the CLP-induced alterations, but the administration of EX527 diminished these effects of H-RSV in the myocardium. Thus, these data demonstrated that RSV reduced ferroptosis in SIC by the upregulation of Sirt1/Nrf2 expression.
Figure 8. Resveratrol prevents sepsis-induced cardiomyopathy from ferroptosis. (A)Relative protein levels of GPX4, ACSL4, TFR and FTH-1, n = 3 per group.(B) The concentrations of GSH in the myocardium tissues, n = 5 per group. (C) The concentrations of iron in the myocardium tissues, n = 5 per group. (D) The concentrations of MDA in the myocardium tissues, n = 5 per group. (E) The representative images of immunofluorescent staining using heart tissues with anti-4-HNE, scar bar = 25 μm, n = 5 per group. Data are expressed as mean ± SD. *p < 0.05 versus Sham. #p < 0.05 versus CLP. &p < 0.05 versus CLP + H-RSV.
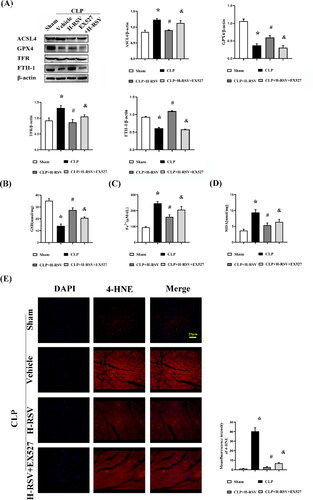
Discussion
In this study, we showed that RSV can alleviate SIC by reducing septic myocardial ferroptosis through the Sirt1/Nrf2 pathway. Specifically, intraperitoneal injection of RSV prior to the CLP model significantly mitigated mitochondrial damage, regulated iron metabolism, and prevented lipid peroxidation, which can ultimately lead to improvement of cardiac dysfunction via ferroptotic suppression (). Moreover, these cardioprotective properties of RSV even at high doses were diminished with the EX527 use. This was suggested by the augmented levels of lipid peroxidation products and ferrous ions, as well as decreased expression of Sirt1/Nrf2 expression. These change supported the fact that RSV promoted cardioprotection from SIC-induced cardiac dysfunction by regulating ferroptosis through upregulation of Sirt1/Nrf2 expression.
Sepsis is a severe organ dysfunction caused by host immune dysfunction in response to infection [Citation1,Citation34], and cardiac dysfunction is a common complication, which often increases patients’ mortality. This reversible cardiac dysfunction is called SIC, and its mechanism remains unclear. Numerous previous studies have reported that SIC involves complex pathological mechanisms ranging from the generation of circulating myocardial depressant substances, mitochondrial dysfunction, and adrenaline-associated pathway downregulation, which may interact to mediate heart dysfunction in sepsis [Citation3,Citation35,Citation36]. As a novel form of cell death, ferritin autophagy- regulated ferroptosis mediates SIC development [Citation5]; this suggests that other signaling modulatory processes may exist, as in the case of increased free iron ions and reactive oxygen radicals that influence SIC.
Ferroptosis, a type of cell death depending on iron and lipid peroxide, is involved in a variety of multi-organ dysfunctions. The heart implicated is one of the main organs affected by ferroptosis [Citation37–39]. However, there is paucity of data regarding the relationship between ferroptosis and extrinsic or intrinsic mechanisms of cardiac defects. A previous study of mice revealed that suppression of ferroptosis by ferrostatin-1 and dexrazoxane is helpful in protecting against SIC injury via ferritinophagy mediation [Citation5]. Furthermore, the maintenance of the natural glutathione antioxidant system provides cardioprotection against many forms of heart disease, including septic cardiomyopathy [Citation40–42]. As shown in our study, the MDA level was stimulated dramatically, while the CLP group had a higher iron concentration. H-RSV and Fer-1 treatment significantly inhibited MDA increase and decrease in Fe2+ concentration. Of note, both are crucial in SIC development and interacts with GSH replenishment, thereby providing a plethora of potential links between ferroptosis and mitochondrial electron transport chain—this may help to elucidate the underlying mechanisms of protection of the myocardium mitochondria exerted by RSV. Oxidative stress is the basis for the pathophysiology of many diseases. ROS, MDA, and 4-HNE are oxidation products. They can impair the biological features of macromolecules such as proteins, lipids, and nucleic acids in cells by altering the conformation [Citation43–45]. In this study, CLP increased the 4-HNE intensity, reduced GPX4 expression, and increased ACSL4 expression. RSV treatment alleviated ferroptosis-associated lipid oxidation and restored protein stability of GPX4 and ACSL4 in SIC more than the Sham procedure did.
RSV, a phytochemicals substance in grapes and red wine, comes to the forefront of researchers as a natural agonist of SIRT1, which is a (NAD+)-dependent histone deacetylase that is involved in several biological processes: cellular metabolism, aging, inflammation, and oxidative stress [Citation46–48]. Sirt1 can activate essential signaling molecules, such as Nrf2. As a stress-inducible transcription factor, Nrf2 is involved in anti-oxidative function, counter inflammatory interaction, and cytoprotection [Citation48–50]. Moreover, important cellular physiological statuses driven by Nrf2-targeting genes include redox homeostasis and, iron metabolism and more [Citation13,Citation51]. For instance, Nrf2 targets are involved in the storage and transport of iron ions by regulating the light chain and heavy chain of ferritin (FTL/FTH1) and ferroportin (SLC40A1), respectively [Citation12,Citation52]. In addition, aldehyde ketone reductase (AKR) and aldehyde dehydrogenase 1 family member A1 (ALDH1A1), which oxidize free aldehyde groups on molecular chains to carboxylic acids, can be substantially induced by Nrf2 [Citation53,Citation54]. As such, our protein expression results suggested that RSV prevents the inactivation of Sirt1 and Nrf2 from CLP, and thus altering the sensitivity of the rat myocardium ferroptosis. Moreover, RSV regulated molecular mechanisms involved in Sirt1/Nrf2 signal transduction, which are beneficial in reducing tissue damage and enhancing the antioxidant defense network. Furthermore, after treatment with RSV, MDA and 4-HNE were curtailed, and the concentration of ferrous ions declined. Regarding the role of increased Sirt1/Nrf2 expression in inhibiting ferroptosis, the reduced lipid peroxidation and iron overload in the RSV-treated septic rats were completely reversed by EX527 via regulation of Nrf2-related antioxidant capacity. Collectively, these data provide support for a multi-factor ferroptosis-associated protective mechanism of RSV.
However, this study had several limitations. First, we concentrated on the anti-ferroptosis effect of RSV, while we did not simultaneously evaluate the function of RSV on other cellular programmed responses, such as, apoptosis, autophagy, and necroptosis. Second, ferroptosis in CLP-related cardiomyopathy was partially verified in the in vivo experiments, but not in the in vitro experiments; therefore, more in-depth molecular biological alterations or stronger chain of evidence through genetic inference and knockouts were not investigated. Third, iron chelators including liproxstatin-1 and other ferroptosis inhibitory drugs were not used to mechanically explore the cardioprotective and therapeutic effects on sepsis-induced myocardial injury. Further studies considering these limitations are warranted.
Conclusions
Our findings confirmed that RSV significantly mitigated the myocardial damage induced by SIC via regulation of Sirt1/Nrf2-mediated ferroptotic alterations. As such, tour findings provide more knowledge on the cardioprotective benefits of RSV via Sirt1/Nrf2 activation in septic myocardiopathy. RSV may be a potential effective treatment for patients with sepsis.
Disclosure statement
The authors declare no conflict of interest.
Additional information
Funding
References
- Singer M, Deutschman CS, Seymour CW, et al. The third international consensus definitions for sepsis and septic shock (Sepsis-3). Jama. 2016;315(8):1. doi:10.1001/jama.2016.0287.
- L'Heureux M, Sternberg M, Brath L, et al. Sepsis-induced cardiomyopathy: a comprehensive review. Curr Cardiol Rep. 2020;22(5):35. doi:10.1007/s11886-020-01277-2.
- Hollenberg SM, Singer M. Pathophysiology of sepsis-induced cardiomyopathy. Nat Rev Cardiol. 2021;18(6):424–15. doi:10.1038/s41569-020-00492-2.
- Li D, Liu X, Pi W, et al. Fisetin attenuates doxorubicin-induced cardiomyopathy in vivo and in vitro by inhibiting ferroptosis through SIRT1/Nrf2 signaling pathway activation. Front Pharmacol. 2021;12:808480. doi:10.3389/fphar.2021.808480.
- Li N, Wang W, Zhou H, et al. Ferritinophagy-mediated ferroptosis is involved in sepsis-induced cardiac injury. Free Radic Biol Med. 2020;160:303–318. doi:10.1016/j.freeradbiomed.2020.08.009.
- Sönmez Aydın F, Hukkamlı B, Budak H. Coaction of hepatic thioredoxin and glutathione systems in iron overload-induced oxidative stress. J Biochem Mol Toxicol. 2021;35(4):e22704. doi:10.1002/jbt.22704.
- Wang X, Chen X, Zhou W, et al. Ferroptosis is essential for diabetic cardiomyopathy and is prevented by sulforaphane via AMPK/NRF2 pathways. Acta Pharm Sin B. 2022;12(2):708–722. doi:10.1016/j.apsb.2021.10.005.
- Dixon SJ, Lemberg KM, Lamprecht MR, et al. Ferroptosis: an iron-dependent form of nonapoptotic cell death. Cell. 2012;149(5):1060–1072. doi:10.1016/j.cell.2012.03.042.
- Hirschhorn T, Stockwell BR. The development of the concept of ferroptosis. Free Radic Biol Med. 2019;133:130–143. doi:10.1016/j.freeradbiomed.2018.09.043.
- Stockwell BR, Jiang X, Gu W. Emerging mechanisms and disease relevance of ferroptosis. Trends Cell Biol. 2020;30(6):478–490. doi:10.1016/j.tcb.2020.02.009.
- Chen X, Li J, Kang R, et al. Ferroptosis: machinery and regulation. Autophagy. 2021;17(9):2054–2081. doi:10.1080/15548627.2020.1810918.
- Harada N, Kanayama M, Maruyama A, et al. Nrf2 regulates ferroportin 1-mediated iron efflux and counteracts lipopolysaccharide-induced ferroportin 1 mRNA suppression in macrophages. Arch Biochem Biophys. 2011;508(1):101–109. doi:10.1016/j.abb.2011.02.001.
- Dodson M, Castro-Portuguez R, Zhang DD. NRF2 plays a critical role in mitigating lipid peroxidation and ferroptosis. Redox Biol. 2019;23:101107. doi:10.1016/j.redox.2019.101107.
- Li S, Zheng L, Zhang J, et al. Inhibition of ferroptosis by up-regulating Nrf2 delayed the progression of diabetic nephropathy. Free Radic Biol Med. 2021;162:435–449. doi:10.1016/j.freeradbiomed.2020.10.323.
- Tamaki N, Cristina Orihuela-Campos R, Inagaki Y, et al. Resveratrol improves oxidative stress and prevents the progression of periodontitis via the activation of the Sirt1/AMPK and the Nrf2/antioxidant defense pathways in a rat periodontitis model. Free Radic Biol Med. 2014;75:222–229. doi:10.1016/j.freeradbiomed.2014.07.034.
- Schiedel M, Robaa D, Rumpf T, et al. The current state of NAD(+)-dependent histone deacetylases (sirtuins) as novel therapeutic targets. Med Res Rev. 2018;38(1):147–200. doi:10.1002/med.21436.
- Mallebrera B, Maietti A, Tedeschi P, et al. Antioxidant capacity of trans-resveratrol dietary supplements alone or combined with the mycotoxin beauvericin. Food Chem Toxicol. 2017;105:315–318. doi:10.1016/j.fct.2017.04.027.
- Zargar S, Alonazi M, Rizwana H, et al. Resveratrol reverses thioacetamide-induced renal assault with respect to oxidative stress, renal function, DNA damage, and cytokine release in Wistar rats. Oxid Med Cell Longev. 2019;2019:1702959. doi:10.1155/2019/1702959.
- Davies S, Contri RV, Guterres SS, et al. Simultaneous nanoencapsulation of lipoic acid and resveratrol with improved antioxidant properties for the skin. Colloids Surf B Biointerfaces. 2020;192:111023. doi:10.1016/j.colsurfb.2020.111023.
- Chen Z, Farag MA, Zhong Z, et al. Multifaceted role of phyto-derived polyphenols in nanodrug delivery systems. Adv Drug Delivery Rev. 2021;176:113870. doi:10.1016/j.addr.2021.113870.
- Mo Y, Duan L, Yang Y, et al. Nanoparticles improved resveratrol brain delivery and its therapeutic efficacy against intracerebral hemorrhage. Nanoscale. 2021;13(6):3827–3840. doi:10.1039/d0nr06249a.
- Han B, Li S, Lv Y, et al. Dietary melatonin attenuates chromium-induced lung injury via activating the Sirt1/Pgc-1α/Nrf2 pathway. Food Funct. 2019;10(9):5555–5565. doi:10.1039/c9fo01152h.
- Braud L, Pini M, Stec DF, et al. Increased Sirt1 secreted from visceral white adipose tissue is associated with improved glucose tolerance in obese Nrf2-deficient mice. Redox Biol. 2021;38:101805. doi:10.1016/j.redox.2020.101805.
- Zhang XS, Lu Y, Li W, et al. Astaxanthin ameliorates oxidative stress and neuronal apoptosis via SIRT1/NRF2/Prx2/ASK1/p38 after traumatic brain injury in mice. Br J Pharmacol. 2021;178(5):1114–1132. doi:10.1111/bph.15346.
- Fang X, Wang H, Han D, et al. Ferroptosis as a target for protection against cardiomyopathy. Proc Natl Acad Sci U S A. 2019;116(7):2672–2680. doi:10.1073/pnas.1821022116.
- Li J, Lu K, Sun F, et al. Panaxydol attenuates ferroptosis against LPS-induced acute lung injury in mice by Keap1-Nrf2/HO-1 pathway. J Transl Med. 2021;19(1):96. doi:10.1186/s12967021-02745-1.
- Smeding L, Leong-Poi H, Hu P, et al. Salutary effect of resveratrol on sepsis-induced myocardial depression. Crit Care Med. 2012;40(6):1896–1907. doi:10.1097/CCM.0b013e31824e1370.
- Shang X, Lin K, Yu R, et al. Resveratrol protects the myocardium in sepsis by activating the phosphatidylinositol 3-kinases (PI3K)/AKT/mammalian target of rapamycin (mTOR) pathway and inhibiting the nuclear factor-kappaB (NF-kappaB) signaling pathway. Med Sci Monit. 2019;25:9290–9298. doi:10.12659/MSM.918369.
- Rittirsch D, Huber-Lang MS, Flierl MA, et al. Immunodesign of experimental sepsis by cecal ligation and puncture. Nat Protoc. 2009;4(1):31–36. doi:10.1038/nprot.2008.214.
- Kishimoto C, Kawamata H, Sakai S, et al. Enhanced production of macrophage inflammatory protein 2 (MIP-2) by in vitro and in vivo infections with encephalomyocarditis virus and modulation of myocarditis with an antibody against MIP-2. J Virol. 2001;75(3):1294–1300. doi:10.1128/JVI.75.3.1294-1300.2001.
- Flameng W, Borgers M, Daenen W, et al. Ultrastructural and cytochemical correlates of myocardial protection by cardiac hypothermia in man. J Thorac Cardiovasc Surg. 1980;79(3):413–424. doi:10.1016/S0022-5223(19)37950-4.
- Forcina GC, Dixon SJ. GPX4 at the crossroads of lipid homeostasis and ferroptosis. Proteomics. 2019;19(18):e1800311. doi:10.1002/pmic.201800311.
- Yuan H, Li X, Zhang X, et al. Identification of ACSL4 as a biomarker and contributor of ferroptosis. Biochem Biophys Res Commun. 2016;478(3):1338–1343. doi:10.1016/j.bbrc.2016.08.124.
- Cecconi M, Evans L, Levy M, et al. Sepsis and septic shock. The Lancet. 2018;392(10141):75–87. doi:10.1016/S0140-6736(18)30696-2.
- Liu YC, Yu MM, Shou ST, et al. Sepsis-induced cardiomyopathy: mechanisms and treatments. Front Immunol. 2017;8:1021. doi:10.3389/fimmu.2017.01021.
- Stanzani G, Duchen MR, Singer M. The role of mitochondria in sepsis-induced cardiomyopathy. Biochim Biophys Acta Mol Basis Dis. 2019;1865(4):759–773. doi:10.1016/j.bbadis.2018.10.011.
- Ravingerová T, Kindernay L, Barteková M, et al. The molecular mechanisms of iron metabolism and its role in cardiac dysfunction and cardioprotection. IJMS. 2020;21(21):7889. doi:10.3390/ijms21217889.
- Liu Y, Tan S, Wu Y, et al. The emerging role of ferroptosis in sepsis. DNA Cell Biol. 2022;41(4):368–380. doi:10.1089/dna.2021.1072.
- Van Coillie S, Van San E, Goetschalckx I, et al. Targeting ferroptosis protects against experimental (multi)organ dysfunction and death. Nat Commun. 2022;13(1):1046. doi:10.1038/s41467-022-28718-6.
- Chen K, Wang S, Sun QW, et al. Klotho Deficiency Causes Heart Aging via Impairing the Nrf2-GR Pathway. Circ Res. 2021;128(4):492–507. doi:10.1161/CIRCRESAHA.120.317348.
- Jang S, Chapa-Dubocq XR, Tyurina YY, et al. Elucidating the contribution of mitochondrial glutathione to ferroptosis in cardiomyocytes. Redox Biol. 2021;45:102021. doi:10.1016/j.redox.2021.102021.
- Wu X, Li Y, Zhang S, et al. Ferroptosis as a novel therapeutic target for cardiovascular disease. Theranostics. 2021;11(7):3052–3059. doi:10.7150/thno.54113.
- Atalay S, Gęgotek A, Domingues P, et al. Protective effects of cannabidiol on the membrane proteins of skin keratinocytes exposed to hydrogen peroxide via participation in the proteostasis network. Redox Biol. 2021;46:1020. doi:10.1016/j.redox.2021.102074.
- Li G, Jin J, Liu S, et al. Inhibition of miR-1224 suppresses hypoxia/reoxygenation-induced oxidative stress and apoptosis in cardiomyocytes through targeting GPX4. Exp Mol Pathol. 2021;121:104645. doi:10.1016/j.yexmp.2021.104645.
- Rehman A, Rehman L, Ullah R, et al. Oxidative status and changes in the adenosine deaminase activity in experimental host infected with tropical liver fluke, Fasciola gigantica. Acta Trop. 2021;213:105753. doi:10.1016/j.actatropica.2020.105753.
- Hwang JW, Yao H, Caito S, et al. Redox regulation of SIRT1 in inflammation and cellular senescence. Free Radic Biol Med. 2013;61:95–110. doi:10.1016/j.freeradbiomed.2013.03.015.
- Chang HC, Guarente L. SIRT1 and other sirtuins in metabolism. Trends Endocrinol Metab. 2014;25(3):138–145. doi:10.1016/j.tem.2013.12.001.
- Singh V, Ubaid S. Role of silent information regulator 1 (SIRT1) in regulating oxidative stress and inflammation. Inflammation. 2020;43(5):1589–1598. doi:10.1007/s10753-020-01242-9.
- Zhang B, Zhai M, Li B, et al. Honokiol ameliorates myocardial ischemia/reperfusion injury in type 1 diabetic rats by reducing oxidative stress and apoptosis through activating the SIRT1-Nrf2 signaling pathway. Oxid Med Cell Longev. 2018;2018:3159801. doi:10.1155/2018/3159801.
- Arioz BI, Tastan B, Tarakcioglu E, et al. Melatonin attenuates LPS-induced acute depressive-like behaviors and microglial NLRP3 inflammasome activation through the SIRT1/Nrf2 pathway. Front Immunol. 2019;10:1511. doi:10.3389/fimmu.2019.01511.
- Tonelli C, Chio IIC, Tuveson DA. Transcriptional regulation by Nrf2. Antioxid Redox Signal. 2018;29(17):1727–1745. doi:10.1089/ars.2017.7342.
- Agyeman AS, Chaerkady R, Shaw PG, et al. Transcriptomic and proteomic profiling of KEAP1 disrupted and sulforaphane-treated human breast epithelial cells reveals common expression profiles. Breast Cancer Res Treat. 2012;132(1):175–187. doi:10.1007/s10549-011-1536-9.
- Jung KA, Choi BH, Nam CW, et al. Identification of aldo-keto reductases as NRF2-target marker genes in human cells. Toxicol Lett. 2013;218(1):39–49. doi:10.1016/j.toxlet.2012.12.026.
- Hayes JD, Dinkova-Kostova AT. The Nrf2 regulatory network provides an interface between redox and intermediary metabolism. Trends Biochem Sci. 2014;39(4):199–218. doi:10.1016/j.tibs.2014.02.002.