Abstract
Background
Mesenteric ischemia is a frequently encountered disease in surgical clinics, difficult to diagnose, and very mortal if not treated. Our study investigated the effects of astaxanthin, which is known to have potent antioxidant properties and is also known to have anti-inflammatory effects on ischemia-reperfusion (I/R) injury.
Methods
A total of 32 healthy Wistar albino female rats were used in our study. Subjects were randomized and equally divided into 4 groups; control (laparotomy group only), I/R (transient mesenteric ischemia group only), astaxanthin 1 mg/kg and 10 mg/kg doses. The transient ischemia time was 60 minutes and the reperfusion time was 120 minutes. Tissue samples were taken from intracardiac blood and terminal ileum after reperfusion. Superoxide dismutase (SOD), catalase (CAT), malondialdehyde (MDA) from blood samples, interleukin-1 (IL-1), IL-6, tumor necrosis factor-α (TNFα), Caspase-3, P53 tests from terminal ileum were studied. Tissue samples were also taken for histopathological evaluation.
Results
At the end of the study, both doses of astaxanthin were found to significantly reduce MDA level, CAT, and SOD enzymatic activity, whereas higher doses of astaxanthin significantly reduced MDA level, CAT, and SOD enzyme activities. In addition, cytokines such as TNFα, IL-1 and IL-6 were found to be reduced at both doses of astaxanthin, but only significantly inhibited at higher doses. We observed that inhibition of apoptosis reduced caspase-3 activity and P53 and deoxyribonucleic acid (DNA) fragmentation.
Conclusion
Astaxanthin, a potent antioxidant, and anti-inflammatory, significantly reduces ischemia and reperfusion injury, especially when used at a dose of 10 mg/kg. These data need to be confirmed by larger animal series and clinical studies.
Introduction
Ischemia happens due to inadequate blood waft to tissues because of disruption of arterial or venous blood waft to the organ. Due to tissue hypoxia, cellular energy stores are depleted and cell death occurs after the accumulation of toxic metabolites. New blood flow is needed to clear these toxic metabolites and regenerate the tissue. However, the reflow of blood to the ischemic tissue causes more damage than the damage caused by ischemia [Citation1–3]. Free oxygen radicals are known to be responsible for this damage [Citation4, Citation5]. Therefore, in clinical conditions such as intestinal transplantation, intestinal volvulus, necrotizing enterocolitis and ischemic bowel disease, the importance of understanding the mechanisms of I/R injury and protecting against it is significant [Citation6]. Based on this assumption, studies have been conducted to investigate the effects of endogenous and exogenous antioxidant levels on many diseases. The main goal of these studies was to prevent the activation of neutrophils and oxidant molecules against reperfusion injury [Citation7]. Astaxanthin is a xanthophyll carotenoid produced by microorganisms and various seaweeds, the main source of which is seafood. Therefore, it cannot be synthesized in the human body and must be obtained from food [Citation8, Citation9]. Astaxanthin, like other carotenoids, scavenges singlet oxygen and free radicals have potent antioxidant properties, plays anti-inflammatory and immunomodulatory roles, and promotes the ability to inhibit peroxidation of cell membranes against aging cells. Astaxanthin’s antioxidant activity is 10 times higher than zeaxanthin, lutein, canthaxanthin, and beta-carotene, 600 times higher than vitamin E, and 6000 times higher than vitamin C [Citation10–12]. Increasing proof indicates that astaxanthin inhibits the improvement of oxidative stress-associated illnesses and mitochondrial dysfunction [Citation13]. Astaxanthin protects cells through many mechanisms. Among them; functions include disposing of singlet oxygen, dissipating energy as heat, disposing of radicals to prevent or stop chain reactions, and inhibiting membrane lipid peroxidation to protect membrane structures [Citation14]. Therefore, the development of clinical uses of antioxidants to prevent and/or reduce reperfusion injury in cases of intestinal ischemia remains the main subject of research.
In our study, we aimed to demonstrate the biological effects of the antioxidant astaxanthin on biochemical, histological, and pathological evaluations by generating an intestinal I/R model in rats.
Materials and methods
This experimental study was approved by the Animal Experiments Local Ethics Committee of Eskişehir Osmangazi University Faculty of Medicine on 23.06.2020, with registration number 792-1/2020, being considered by the ethics committee guidelines.
In this study, 32 healthy Wistar albino rats weighing approximately 250-300 g and aged 3-4 months were used as subjects. It was stored in a bright room for 12 hours and a dark room for 12 hours at a room temperature of 20-25 °C. Rats were fed standard rat chow. They were allowed to drink freely water.
In the study, 10% povidone iodine solution, Ketamine Hydrochloride (60 mg/kg) (intramuscular (IM)), Xylazine Hydrochloride (10 mg/kg) (IM), Astaxanthin (≥97% (High-Performance Liquid Chromatography (HPLC)) (SML0982) 50 mg/100 ml) (Sigma -Aldrich Chemie GmbH, Taufkirchen, Germany) was dissolved in Dimethyl sulfoxide (DMSO) at a dose of 1 mg/kg and 10 mg/kg, respectively, to the 3rd and 4th groups, and used intraperitoneally while starting reperfusion. Zheng et al. [Citation15] and Ranga Rao et al. [Citation16] measured the antioxidant activities of astaxanthin at varying doses. Considering these studies, we investigated the effects of different doses as 1 mg/kg and 10 mg/kg. In addition, it is known that the half-life of astaxanthin in studies on plasma concentration is 52 ± 40 hours [Citation17].
Formation of experimental groups and operation procedure
Thirty-two rats were divided into 4 groups of 8 animals using simple random sampling method (In the analysis made using similar studies, the common standard deviation value was calculated as 1.157 and the effect size value as 1.28. In order to reach 80% power by power analysis, at least 3 experimental animals must be used in each group, and since this number is not enough for statistical significance, 8 animals are needed in each group to reach 99% power).
General anesthesia was achieved by administering a mixture of Xylazine (10 mg/kg) and Ketamine (60 mg/kg) intramuscularly under aseptic conditions, following overnight fasting (allowing them to drink only water). After the anesthetized rats were taken to the experimental table, the operation was started by following the rules of asepsis.
Group 1 (normal group-control group)
Only laparotomy followed by superior mesenteric artery (SMA) dissection was performed without creating an I/R model.
Group 2 (ischemia/reperfusion + DMSO)
SMA dissection was performed after laparotomy. Then, ischemia was achieved by clamping SMA for 60 minutes, and intraperitoneal DMSO solution (10 ml) was administered while starting reperfusion for 120 minutes after the clamp was released.
Group 3 (ischemia/reperfusion + Astaxanthin 0.3 mg dose)
SMA dissection was performed after laparotomy. When starting reperfusion after ischemia, 0.3 mg (dissolved in 10 ml DMSO) of astaxanthin was administered intraperitoneally at a dose of 1 mg/kg [Citation15].
Group 4 (ischemia/reperfusion + Astaxanthin 3 mg dose)
SMA dissection was performed after laparotomy. When starting reperfusion after ischemia, 3 mg (dissolved in 10 ml DMSO) of astaxanthin was administered intraperitoneally at a dose of 10 mg/kg [Citation15].
After 120 minutes of reperfusion, IM Ketamine (60 mg/kg) and Xylazine (10 mg/kg) anesthesia were re-administered to all subjects. The sutures on the old incision line were removed and 5 ml of intracardiac blood was taken. Afterward, all subjects were sacrificed by applying cervical dislocation. After sacrification, approximately 5 cm of the small intestine segment was removed proximal to the terminal ileum, and immediately after sacrification, 5 cm of ileum was removed from the proximal terminal ileum and the tissue contents were washed with 0.9% saline solution. The removed ileum segment was divided into two parts and one part was placed in a formaldehyde (10%) solution for histological examination. The other part was divided into equal parts and placed in microcentrifuge (Eppendorf) tubes. It was stored at −80 °C until the time of processing.
Blood samples for the study of plasma superoxide dismutase (SOD), catalase (CAT), and malondialdehyde (MDA) from all subjects used in the study; Tissue samples were taken from the terminal ileum for caspase-3, P53, tumor necrosis factor-α (TNFα), interleukin-1 (IL-1), IL-6 study and histopathological evaluation.
Examination of MDA, SOD and CAT from blood plasma by enzyme-linked ımmunosorbent assay (ELISA) method
Intracardiac blood samples have been received from all rats in keeping with the procedure. Collected blood samples have been positioned in EDTA (ethylenediaminetetraacetic acid) tubes. The tube became centrifuged at 4100 G-force(G) for 15 minutes. The last plasma withinside the top section became pipetted right into a sterile tube. Then, it became saved at −80 °C till the day of the experiment. On the day of the experiment, the samples have been thawed at room temperature and the stages of MDA, SOD, and CAT have been measured.
Malondialdehyde (MDA) measurement (nmol/L)
Tissue MDA levels were determined by measuring colored compounds at a wavelength of 532 nm as a result of the reaction using thiobarbituric acid (TBA) at 90-100 °C. Results are given in nmol/l.
Superoxide dismutase (SOD) activity measurement (U/ml)
Measurement of SOD activity in plasma was performed by measuring the intensity of the purple formazan dye produced by reaction with iodonitrotetrazolium at a wavelength of 505 nm. Results are given in U/ml.
Catalase (CAT) activity measurement (U/ml)
The serum sample was converted into hydrogen and oxygen by reacting with hydrogen peroxide. The reaction rate was proportional to the catalase concentration. Catalase activity in plasma was measured by chromogen. The absorbance acquired becomes measured at 405 nm and the effects acquired had been expressed as U/ml.
Measurement of caspase-3, P53, TNFα, IL-1, IL-6 in tissue by polymerase chain reaction (PCR) method
After the applied procedures, messenger Ribonucleic Acid (mRNA) was isolated from the studied material tissue sample from the terminal ileum tissue of all rats for the measurement of Caspase-3, P53, TNFα, IL-1, IL-6.
HyperScriptTM First strand synthesis kit was used for complementary Deoxyribonucleic Acid (cDNA) synthesis after mRNA isolation. After the cDNA master mix was prepared, a two-step reverse transcription reaction was initiated. After obtaining the cDNA, mRNA expressions were obtained for Real-Time qPCR using the RealAmpTM SYBR quantitative PCR (qPCR) Master mix kit.
Quantification of mRNA expressions was normalized relative to the control group using the ACTB transcript as a reference. Fold Change was calculated using the “ΔΔCt Method” for calculating the relative quantification. If the Fold Change value is above 1, it was interpreted that the mRNA expression of the target group increased compared to the mRNA expression of the reference group.
Histopathological examination
Hematoxylin-eosin (H&E)
Intestinal tissue samples were first fixed with a 4% paraformaldehyde solution. Tissues were obtained after fixation and paraffin blocks were obtained after routine procedures. The resulting 5 µm serial sections were stained with H&E. The sections were then viewed with an Olympus BX 51 light microscope. Then, the findings were evaluated by scoring according to the Chiu score ().
Table 1. Chiu histological scoring.
Immunohistochemical staining method
Paraffin sections were taken at 3 µm thickness and treated with protein block after routine staining and washing with PBS (phosphate-buffered saline). After incubation with an anti-caspase-3 antibody, it was incubated with Amplifier Quanto and HRP Polymer Quanto, respectively. After washing, hematoxylin was applied for chromogen and soil staining. After washing with distilled water, the sections were visualized with an Olympus BX 51 light microscope.
TdT-dUTP nick-end-labelling (TUNEL) stain method
Proteinase K enzyme was applied to the sections taken at 3 µm thickness in a 37 °C oven. Peroxidase activity of the tissues was blocked with 3% hydrogen peroxide. Samples were washed with PBS. Treated with equilibration buffer. The TdT enzyme was kept in a working solution in an oven at 37 °C. After washing with the Working Stop/Wash buffer solution, they were treated with digoxigenin peroxidase and washed with PBS. Chromogen staining was performed to identify positive cells. Methylene green is used to paint the floor. After washing with distilled water, sections were viewed with an Olympus BX 51 light microscope.
Periodic acid–Schiff (PAS) stain method
Sections taken at 3 µm thickness were kept in Periodic Acid and Schiff reagent solution, respectively, after being separated. After washing the slides with alcohol and distilled water, they were examined under an Olympus BX 51 light microscope.
Statistical analysis
For statistical analysis, IBM SPSS Statistics 22 was used. Using the Kolmogorov-Smirnov and Shapiro-Wilk tests of normality, it was determined whether the groups were normally distributed. Not all parameters showed a normal distribution. Differences between groups were evaluated using the Kruskal-Wallis test. Dunn’s test was used as the multiple comparison test. P-values less than 0.05 were considered significant. One-way ANOVA test was used in histopathological examinations and the TUKEY test was used for post hoc test.
Results
CAT, SOD and MDA values measured in plasma
CAT enzyme activities were statistically very high compared to all other groups and significantly different compared to the control group (p < 0.001) (). CAT enzyme activities were found to be significantly lower in both drug treatment groups (0.3 mg, 3 mg astaxanthin) compared to the reperfusion group (p = 0.021, p < 0.001).
Figure 1. After intestinal I/R of astaxanthin, (A) CAT, (B) SOD, (C) MDA, (D) Caspase-3, (E) IL-1, (F) IL-6, (G) P53 and (H) Effect on TNFα values (All groups were compared with the I/R group and shown as p < 0.001 ★★★, p < 0.01 ★★, p < 0.05 ★).
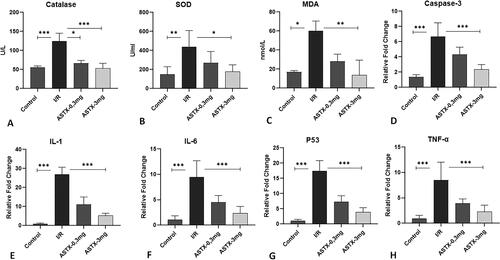
SOD enzyme activities were higher in the reperfusion group than in the control group (p = 0.002) (). SOD enzyme activities in the 3 mg astaxanthin group were significantly lower than in the reperfusion group (p = 0.029). However, there was no adequate response in the 0.3 mg drug group compared to the reperfusion group (p = 1.00).
Comparing the MDA values, the reperfusion group was significantly higher than the control group (p < 0.001) (). MDA values in the astaxanthin-administered group were lower than those in the reperfusion group. However, there was a significant difference in the group receiving high-dose astaxanthin compared to the I/R group (p = 0.009).
Caspase-3, P53, IL-1, IL-6, TNF alpha values measured from tissue by PCR method
When statistically comparing levels of caspase-3, IL-1, IL-6, P53, and TNFα measured from tissues by PCR method, significant differences between groups were observed (p < 0.001). All proinflammatory cytokines and caspase-3 were significantly higher in the I/R group than in the control group (p < 0.001) (). Cytokines and caspase-3 were significantly lower in the 3 mg astaxanthin group compared to the I/R group (p < 0.001). Additionally, cytokine levels in the astaxanthin 3 mg and control groups were similar and not significantly different (p > 0.05). However, cytokine levels and caspase-3 I/R groups were lower in the low-dose astaxanthin group, but the difference was not significant (p > 0.05).
Histopathological findings
Hematoxylin eosin staining
Each group was histopathologically stained with HE staining and then examined in detail by light microscopy (). The presence of healthy mucous villi was observed in the control group. In the I/R group, lamina propria ulceration and villous bleeding areas were observed. Subepithelial congestion and villus tip ulceration were observed in the astaxanthin 0.3 mg administration group. In the astaxanthin 3 mg group, congestion of some villus bases and a decrease in the number of goblet cells compared to normal was observed. Consistent with these results, when we calculated the Chiu score for each group and statistically compared the Chiu scores between groups, we found a significant difference between groups (p < 0.001). A significant increase in Chiu score was observed when comparing the control and I/R groups (p < 0.001). Furthermore, Chiu scores were significantly lower in both the low-dose and high-dose astaxanthin groups than in the I/R group (p < 0.001, p < 0.001).
Caspase-3 immunoreactivity
A statistically significant difference between groups was observed when comparing caspase-3 immunoreactivity in each group (p < 0.001) (). Caspase-3 immunoreactivity was significantly higher when comparing the control and I/R groups (p < 0.001). Comparing the astaxanthin-treated group with the I/R group, caspase-3 immunoreactivity decreased in proportion to the increase in astaxanthin dose, and a statistically significant difference was observed (p < 0.001, p < 0.001).
PAS stain
After PAS staining, the number of goblet cells was examined separately for each group. We observed that the number of goblet cells was at least in the I/R group (). We found that increasing the dose of astaxanthin increased the number of goblet cells.
TUNEL stain
DNA breaks detected in situ by TUNEL staining were statistically evaluated between groups and were significantly different between groups (p < 0.001) (). When comparing the I/R group with the control group, DNA breaks were observed to be significantly higher in the I/R group (p < 0.001). A statistical comparison between the increased dose of astaxanthin and the I/R group showed a significant difference between the groups (p < 0.001, p < 0.001). Although there was a statistical difference between the astaxanthin 3 mg administration group and the control group, numerically similar results were obtained (p < 0.05).
Discussion
Intestinal ischemia is an acute, life-threatening condition resulting from a variety of causes, including trauma, intestinal transplantation, cardiovascular and abdominal aortic surgery, sepsis, and shock [Citation18–20]. Diagnosis and clinical cases are difficult, leading to high morbidity and mortality [Citation21].
Numerous pathophysiological mechanisms play a role, such as energy production through anaerobic metabolism, decrease in intracellular pH due to an increase in lactic acid, decrease in oxygen and ATP amount during I/R injury, and formation of reactive oxygen species [Citation22, Citation23]. In addition, widespread inflammation and free radical formation disrupt the balance of oxidants and antioxidants, and the resulting oxidative stress leads to organ dysfunction [Citation24]. The reason for this is suspected to be neutrophils migrating extracellularly due to blood flow decrease to the tissue during I/R. The enzyme myeloperoxidase (MPO) released by neutrophils causes the enlargement of capillary lumens. In addition, free oxygen radicals are formed during reperfusion by the conversion of xanthine dehydrogenase to xanthine oxidase caused by hypoxia [Citation25].
MDA is outcome of the lipid peroxidation process and projects the state of reactive oxygen species. During I/R injury, neutrophil activation causes migration to inflammatory sites, and neutrophils exacerbate tissue damage by reactive oxygen species and several toxic enzymes such as MPO [Citation22]. SOD is a metalloprotein enzyme that converts superoxide radicals to hydrogen peroxide and is effective in disproportionation reactions [Citation26]. SOD, glutathione peroxidase, CAT enzymes, and vitamins A, C, and E are key components of this mechanism. However, the large amount of free oxygen radicals generated during the reperfusion phase cannot be removed by this system. Therefore, a lot of antioxidants have been evaluated both for treatment and reduction of the damage caused by I/R injury [Citation27, Citation28]. Himanthalia elongata, one of the brown algae species, is also known to have high antioxidant activity and reduce MDA levels and SOD enzyme activities [Citation29].
When establishing an I/R model in experimental animals, sufficient time should be allowed for ischemia to be at a sufficient level and for reperfusion damage to occur. Therefore, as in similar studies, we determined and applied the ischemia time as 60 minutes and the reperfusion time as 120 minutes [Citation20–32]. The common result of many studies showings the effectiveness of experimental and clinical use of free oxygen radical scavengers; antioxidants should be administered before reperfusion begins [Citation33]. In our study, based on this information, we administered astaxanthin intraperitoneally before starting reperfusion.
Catalase, a naturally occurring metalloprotein in the body, reduces the destructive effects of water and oxygen by hydrogen peroxide (H2O2), one of the oxygen free radicals caused by I/R. Zhonghui et al. [Citation34] demonstrated I/R injury in a 2014 study. They found that SOD and CAT enzyme activities increased with I/R injury. In our study, CAT enzyme activities were higher in the I/R group compared with the control group. CAT enzyme activities were lower in both astaxanthin-administered groups than in the I/R group, and there was no difference between them and the control group, indicating that even low doses of astaxanthin reduce CAT enzyme activities. Hasturk et al. [Citation35] and Kandemir et al. [Citation36], it was thought that astaxanthin administered groups significantly reduced the production of SOD and CAT by preventing the aggregation of leukocytes to the ischemic region.
When free radicals attack membrane lipoproteins and polyunsaturated fatty acids, many oxygen-containing compounds, especially aldehydes such as malondialdehyde, are formed. The observed increase in intestinal MDA levels indicates lipid peroxidation, and oxidative damage to intestinal tissue has also been confirmed in animal models [Citation37]. According to Shen et al. [Citation38], a 2009 study used MDA levels to detect ischemic brain injury and found higher MDA levels in the I/R injury group. It was measured to indicate the formation of free oxygen radicals in tissues and was lowest in the control group and highest in the I/R group. was observed to reduce oxygen free radicals.
The increase in SOD enzyme activity during reperfusion after ischemia causes elevation of superoxide radicals and thus exacerbation of tissue damage [Citation36]. It has been shown that SOD enzyme activity increased in rats by creating an I/R model, and antioxidant molecules also decrease this activity [Citation36, Citation39]. In our study, we observed significantly higher SOD enzyme activity when comparing the I/R group with the control group. In addition, when comparing the high-dose astaxanthin group with the I/R group, a significant decrease in SOD enzyme activity was observed, and there was no difference from the control group. However, it was found that lower doses were not adequately responsive.
Many studies to demonstrate the activity of caspase-3, which is known to be the primary initiator of apoptosis, have shown that caspase-3 initiates apoptosis after ischemia, leading to cell death [Citation40]. In our study, the expression intensity of caspase-3 immunoreactivity assessed histologically at the cellular level was most strongly observed in the I/R group compared with the control group. Furthermore, high-dose astaxanthin was found to suppress apoptosis by reducing caspase-3 activity to levels close to normal tissue levels.
Another process in I/R injury is the increase of pro-inflammatory cytokines such as TNFα, IL-1, and IL-6, which cause leukocyte infiltration into damaged tissue [Citation41]. Intestinal I/R injury causes a 4-fold increase in plasma cytokine levels compared to hypoxic injury alone. At the 15th minute of reperfusion, plasma TNFα, IL-1, and IL-6 levels begin to rise and peak at 4 hours [Citation35, Citation42]. In many experimental studies, it has been shown that there is a close relationship between plasma cytokine level and the development of Multiple Organ Failure (MOF) after I/R injury, regardless of the etiological cause of intestinal I/R injury. This close relationship brought up the use of cytokines as early diagnosis markers and it has been shown that TNFα, IL-1, and IL-6 play the leading roles in the development of Systemic Inflammatory Response Syndrome (SIRS) and MOF after acute intestinal ischemia.
Li et al. [Citation43] provided strong evidence that astaxanthin inhibits the production of inflammatory cytokines such as TNFα, IL-1, and IL-6 in serum and tissue in their liver I/R injury study. In our study, it was observed that the anti-inflammatory properties of astaxanthin and the activity of TNFα, IL-1, and IL-6 decreased in a dose-dependent manner.
The P53 gene is a tumor suppressor gene that is activated when DNA damage occurs. The activation of P53 blocks the cell whose DNA is damaged in the G1 phase and gives the cell time for DNA repair. In cells with unrepaired DNA, apoptosis begins when the P53 gene activates the Bax protein [Citation44]. In our study, it was observed that P53 increased considerably during I/R and decreased significantly after high-dose astaxanthin.
Many scoring systems are used to rate intestinal ischemia and reperfusion injury and to classify it in both histological and pathological terms. Among these, the most commonly used grading methods are; Chiu score [Citation45], Park score [Citation46], and Hierholzer et al. [Citation47] is the scoring system. However, Chiu et al. The Chiu score he used was also preferred in our study because it is the most frequently used method, as well as its applicability and a good indication of tissue damage.
When the groups were histopathologically evaluated according to the Chiu score of tissue stained with HE, the control group had healthy mucosal villi and the I/R group had ulceration of the lamina propria and hemorrhagic villi. Subepithelial congestion and ulceration at the apex of the villi were observed in the astaxanthin 0.3 mg group, while partial villi congestion and a decrease in the number of goblet cells were observed in the astaxanthin 3 mg group. Astaxanthin administration reduced I/R damage in intestinal tissue at the same rate, and the number of goblet cells was lowest in the I/R group as assessed by PAS staining. Increasing the dose of astaxanthin increased the number of goblet cells.
The TUNEL method enables the detection of DNA breaks during apoptosis in vitro [Citation48, Citation49]. In our study, high-dose astaxanthin prevents the formation of DNA breaks by reducing apoptosis that occurs during I/R injury.
It has been reported that Astaxanthin, a member of the ketone family of carotenoids, has a powerful mechanism for protecting cell membrane structures [Citation50]. Astaxanthin, like other carotenoids, takes away singlet oxygen and other free radicals by absorbing the energy released by the excitation of singlet oxygen and forming electron-rich polyene chains. In this way, astaxanthin protects cells and tissues from damage. The carotenoid structure remains unchanged and is again used as a radical scavenger [Citation14]. In our study, astaxanthin prevented I/R injury, consistent with the literature [Citation38, Citation43, Citation51–55]. It has been found to have anti-inflammatory effects by reducing oxygen free radicals, inhibiting lipid peroxidation, delaying cell death by preventing apoptosis, and inhibiting pro-inflammatory cytokines. In addition, when we examined it histologically, it has been proven that it increases the number of goblet cells and reduces the ulcerations seen in the mucosa after ischemia. Although it has been shown that it has an effect at both doses, it was observed that the effect of astaxanthin was stronger and more significant when given at a dose of 10 mg/kg.
Consequently, astaxanthin, with its potent antioxidant and anti-inflammatory properties, significantly reduces I/R damage, especially when used at a dose of 10 mg/kg. Astaxanthin may become a potential drug for routine clinical use if I/R studies continue.
Study limitations
The most important limitation of the study is that the drug was studied only in rats with acute mesenteric ischemia. In addition, further investigation in different diseases and with different methods will contribute to a better understanding of the effects of this compound.
Acknowledgments
This experimental study was approved by the Animal Experiments Local Ethics Committee of Eskişehir Osmangazi University Faculty of Medicine on 23.06.2020, with the registration number 792-1/2020, being considered in accordance with the ethics committee guidelines.
Disclosure statement
No potential conflict of interest was reported by the authors.
Additional information
Funding
References
- Zimmerman BJ, Granger DN. Reperfusion injury. Surg Clin North Am. 1992;72(1):1–9. doi:10.1016/s0039-6109(16)45628-8.
- Guerin M, Huntley ME, Olaizola M. Haematococcus astaxanthin: Applications for human health and nutrition. Trends Biotechnol. 2003;21(5):210–216. doi:10.1016/S0167-7799(03)00078-7.
- Tinkler JH, Böhm F, Schalch W, Truscott TG. Dietary carotenoids protect human cells from damage. J Photochem Photobiol B. 1994;26(3):283–285. doi:10.1016/1011-1344(94)07049-0.
- Collard CD, Gelman S. Pathophysiology, clinical manifestations, and prevention of ischemia-reperfusion injury. Anesthesiology. 2001;94(6):1133–1138. doi:10.1097/00000542-200106000-00030.
- Demiryilmaz I, Turan MI, Kisaoglu A, Gulapoglu M, Yilmaz I, et al. Protective effect of nimesulide against hepatic ischemia/reperfusion injury in rats: Effects on oxidant/antioxidants, DNA mutation and COX-1/COX-2 levels. Pharmacol Rep. 2014;66(4):647–652. doi:10.1016/j.pharep.2014.02.015.
- Gonzalez LM, Moeser AJ, Blikslager AT. Animal models of ischemia-reperfusion-induced intestinal injury: Progress and promise for translational research. Am J Physiol Gastrointest Liver Physiol. 2015;308(2):G63–G75. doi:10.1152/ajpgi.00112.2013.
- Riaz AA, Wan MX, Schäfer T, Dawson P, Menger MD, et al. Allopurinol and superoxide dismutase protect against leucocyte-endothelium interactions in a novel model of colonic ischaemia-reperfusion. Br J Surg. 2002;89(12):1572–1580. doi:10.1046/j.1365-2168.2002.02279.x.
- Ambati RR, Phang SM, Ravi S, Aswathanarayana RG. Astaxanthin: sources, extraction, stability, biological activities and its commercial applications-a review. Marine Drugs. 2014;12(1):128–152. doi:10.3390/md12010128.
- Nagao A. Absorption and metabolism of dietary carotenoids. Biofactors. 2011;37(2):83–87. doi:10.1002/biof.151.
- Lee DH, Lee YJ, Kwon KH. Neuroprotective effects of astaxanthin in oxygen-glucose deprivation in sh-sy5y cells and global cerebral ischemia in rat. J Clin Biochem Nutr. 2010;47(2):121–129. doi:10.3164/jcbn.10-29.
- Nakajima Y, Inokuchi Y, Shimazawa M, Otsubo K, Ishibashi T, et al. Astaxanthin, a dietary carotenoid, protects retinal cells against oxidative stress in-vitro and in mice in-vivo. J Pharm Pharmacol. 2008;60(10):1365–1374. doi:10.1211/jpp/60.10.0013.
- Yang Y, Kim B, Lee JY. Astaxanthin structure, metabolism, and health benefits. J Human Nutr Food Sci. 2013;1(1003):1–1003.
- Kuroki T, Ikeda S, Okada T, Maoka T, Kitamura A, et al. Astaxanthin ameliorates heat stress-induced impairment of blastocyst development in vitro: Astaxanthin colocalization with and action on mitochondria. J Assist Reprod Genet. 2013;30(5):623–631. doi:10.1007/s10815-013-9987-z.
- Çolak E. Testiste oluşturulan iskemi/reperfüzyon hasarı üzerine Astaksantin’in koruyucu etkisi. ESOGÜ, Sağlık Bilimleri Enstitüsü (in Turkish) [Master’s Thesis], 2014.
- Zheng D, Li Y, He L, Tang Y, Li X, et al. The protective effect of astaxanthin on fetal alcohol spectrum disorder in mice. Neuropharmacology. 2014;84:13–18. doi:10.1016/j.neuropharm.2014.04.013.
- Ranga Rao A, Raghunath Reddy RL, Baskaran V, Sarada R, Ravishankar GA. Characterization of microalgal carotenoids by mass spectrometry and their bioavailability and antioxidant properties elucidated in rat model. J Agric Food Chem. 2010;58(15):8553–8559. doi:10.1021/jf101187k..
- Coral-Hinostroza GN, Ytrestøyl T, Ruyter B, Bjerkeng B. Plasma appearance of unesterified astaxanthin geometrical E/Z and optical R/S isomers in men given single doses of a mixture of optical 3 and 3’R/S isomers of astaxanthin fatty acyl diesters. Comp Biochem Physiol C Toxicol Pharmacol. 2004;139(1-3):99–110. doi:10.1016/j.cca.2004.09.011.
- Chang JX, Chen S, Ma LP, Jiang LY, Chen JW, et al. Functional and morphological changes of the gut barrier during the restitution process after hemorrhagic shock. World J Gastroenterol. 2005;11(35):5485–5491. doi:10.3748/wjg.v11.i35.5485.
- Petrat F, Swoboda S, de Groot H, Schmitz KJ. Quantification of ischemia-reperfusion injury to the small intestine using a macroscopic score. J Invest Surg. 2010;23(4):208–217. doi:10.3109/08941931003623622.
- Tanyeli A, Ekinci Akdemir FN, Eraslan E, Özbek Sebin S, Gulcin İ. Role of p-Coumaric acid in Alleviating of the Intestinal Ischemia/Reperfusion Injury. Kocaeli Tıp Dergisi. 2020;9(1):166–173. (in Turkish).
- Grootjans J, Lenaerts K, Buurman WA, Dejong CH, Derikx JP. Life and death at the mucosal-luminal interface: New perspectives on human intestinal ischemia-reperfusion. World J Gastroenterol. 2016;22(9):2760–2770. doi:10.3748/wjg.v22.i9.2760.
- Mallick IH, Yang W, Winslet MC, Seifalian AM. Ischemia-reperfusion injury of the intestine and protective strategies against injury. Dig Dis Sci. 2004;49(9):1359–1377. doi:10.1023/b:ddas.0000042232.98927.91.
- Mester A, Magyar Z, Sogor V, Tanczos B, Stark Y, et al. Intestinal ischemia-reperfusion leads to early systemic micro-rheological and multiorgan microcirculatory alterations in the rat. Clin Hemorheol Microcirc. 2018;68(1):35–44. doi:10.3233/CH-170278.
- Carden DL, Granger DN. Pathophysiology of ischaemia-reperfusion injury. J Pathol. 2000;190(3):255–266. doi:10.1002/(SICI)1096-9896(200002)190:3 < 255::AID-PATH526 > 3.0.CO;2-623. Granger DN, Korthuis RJ. Physiologic mechanisms of postischemic tissue injury. Annual Review of Physiology 1995; 57:311-332. doi:10.1146/annurev.ph.57.030195.001523.
- Gülçin I. Antioxidant properties of resveratrol: A structure–activity insight. Innovative Food Sci Emerg Technol. 2010;11(1):210–218. doi:10.1016/j.ifset.2009.07.002.
- Barut I, Tarhan OR, Kapucuoglu N, Sutcu R, Akdeniz Y. Lamotrigine reduces intestinal I/R injury in the rat. Shock. 2007;28(2):202–206. doi:10.1097/SHK.0b013e3180311dee.
- Yildiz Y, Kose H, Cecen S, Ergin K, Demir EM, et al. Protective effects of leflunomide on intestinal ischemia-reperfusion injury: leflunomide against intestinal ischemia-reperfusion. Dig Dis Sci. 2010;55(2):245–252. doi:10.1007/s10620-009-0737-0.
- Aygun H, Olguner C, Koca U, Ergur BU, Sisman AR, et al. The effect of post-reperfusion levosimendan in an experimental intestinal ischemia–reperfusion model. J Anesth Analg Crit Care. 2022;2(1):45. doi:10.1186/s44158-022-00074-3.
- Belda-Antolí M, Padrón-Sanz C, Cejalvo-Lapeña D, Prieto-Moure B, Lloris-Cejalvo JM, et al. Antioxidant potential of Himanthalia elongata for protection against ischemia-reperfusion injury in the small bowel. Surgery. 2017;162(3):577–585. doi:10.1016/j.surg.2017.04.017.
- Liu L, Zhuang R, Xiao L, Chung HK, Luo J, et al. HuR enhances early restitution of the intestinal epithelium by increasing Cdc42 translation. Mol Cell Biol. 2017;37(7):e00574-16. doi:10.1128/MCB.00574-16.
- Özkaya MO. Effects of Levosimendan on Rats Intestinal Ischemia Reperfusion Injury. Dokuz Eylül Üniversitesi Tıp Fakültesi (in Turkish) [Doctoral dissertation], 2010.
- Zhang W, Zhu W, Zhang J, Li N, Li J. Protective effects of glucagon-like peptide 2 on intestinal ischemia-reperfusion rats. Microsurgery. 2008;28(4):285–290. doi:10.1002/micr.20491.
- Liao XP, She YX, Shi CR, Li M. Changes in body fluid markers in intestinal ischemia. J Pediatr Surg. 1995;30(10):1412–1415. doi:10.1016/0022-3468(95)90394-1.
- Zhonghui Z, Xiaowei Z, Fang F. Ganoderma lucidum polysaccharides supplementation attenuates exercise-induced oxidative stress in skeletal muscle of mice. Saudi J Biol Sci. 2014;21(2):119–123. doi:10.1016/j.sjbs.2013.04.004.
- Hasturk A, Atalay B, Calisaneller T, Ozdemir O, Oruckaptan H, et al. Analysis of serum pro-inflammatory cytokine levels after rat spinal cord ischemia/reperfusion injury and correlation with tissue damage. Turkish Neurosurg. 2009;19(4):353–359.
- Kandemir M, Yaşar NF, Özkurt M, Özyurt R, Bektur Aykanat NE, et al. The role of anakinra in the modulation of intestinal cell apoptosis and inflammatory response during ischemia/reperfusion. Turk J Med Sci. 2021;51(4):2177–2184. doi:10.3906/sag-2008-258.
- Zheng X, Mao Y, Cai J, Li Y, Liu W, et al. Hydrogen-rich saline protects against intestinal ischemia/reperfusion injury in rats. Free Radic Res. 2009;43(5):478–484. doi:10.1080/10715760902870603.
- Shen H, Kuo CC, Chou J, Delvolve A, Jackson SN, et al. Astaxanthin reduces ischemic brain injury in adult rats. Faseb J. 2009;23(6):1958–1968. doi:10.1096/fj.08-123281.
- Dimakakos PB, Kotsis T, Kondi-Pafiti A, Katsenis K, Doufas A, et al. Oxygen free radicals in abdominal aortic surgery. An experimental study. J Cardiovasc Surg. 2002;43(1):77–82.
- Sakurai M, Nagata T, Abe K, Horinouchi T, Itoyama Y, et al. Survival and death-promoting events after transient spinal cord ischemia in rabbits: induction of Akt and caspase3 in motor neurons. J Thorac Cardiovasc Surg. 2003;125(2):370–377. doi:10.1067/mtc.2003.112.
- Nezu Y, Nezu Y, Shigihara K, Harada Y, Yogo T, et al. Effects of small intestinal ischemia and reperfusion on expression of tumor necrosis factor-alpha and interleukin-6 messenger RNAs in the jejunum, liver, and lungs of dogs. Am J Vet Res. 2008;69(4):512–518. doi:10.2460/ajvr.69.4.512.
- Karaağaç H, Zeybek N, Peker Y, Yağcı G, Şengül A, et al. Diagnostic value of plasma cytokine levels in acute mesenteric ischemia: an experimental study. GüLhane Tip Dergisi. 2007;49(4):216.
- Li J, Wang F, Xia Y, Dai W, Chen K, et al. Astaxanthin pretreatment attenuates hepatic ischemia reperfusion-induced apoptosis and autophagy via the ROS/MAPK pathway in mice. Mar Drugs. 2015;13(6):3368–3387. doi:10.3390/md13063368.
- Kiess W, Gallaher B. Hormonal control of programmed cell death/apoptosis. Eur J Endocrinol. 1998;138(5):482–491. doi:10.1530/eje.0.1380482.
- Topaloğlu Ü, Güran M, Odabaşı M, Karadağ N. The Effects of Prostaglandın E2 on Ischemia-Reperfusion Damage, Resulting From Mesenter Arterial Ischemia in Small Intestines. Ulusal travma ve acil cerrahi dergisi. 1997;3(4):258–264. (in Turkish).
- Stallion A, Kou TD, Latifi SQ, Miller KA, Dahms BB, et al. Ischemia/reperfusion: A clinically relevant model of intestinal injury yielding systemic inflammation. J Pediatr Surg. 2005;40(3):470–477. doi:10.1016/j.jpedsurg.2004.11.045.
- Hierholzer C, Kalff JC, Audolfsson G, Billiar TR, Tweardy DJ, et al. Molecular and functional contractile sequelae of rat intestinal ischemia/reperfusion injury. Transplantation. 1999;68(9):1244–1254. doi:10.1097/00007890-199911150-00006.
- Gavrieli Y, Sherman Y, Ben-Sasson SA. Identification of programmed cell death in situ via specific labeling of nuclear DNA fragmentation. J Cell Biol. 1992;119(3):493–501. doi:10.1083/jcb.119.3.493.
- Kressel M, Groscurth P. Distinction of apoptotic and necrotic cell death by in situ labelling of fragmented DNA. Cell Tissue Res. 1994;278(3):549–556. doi:10.1007/BF00331373.
- Halliwell B. Antioxidants and human disease: a general introduction. Nutr Rev. 1997;55(1 Pt 2):S44–S52. doi:10.1111/j.1753-4887.1997.tb06100.x.
- Li S, Takahara T, Fujino M, Fukuhara Y, Sugiyama T, et al. Astaxanthin prevents ischemia-reperfusion injury of the steatotic liver in mice. PLoS One. 2017;12(11):e0187810. doi:10.1371/journal.pone.0187810.
- Cakir E, Cakir U, Tayman C, Turkmenoglu TT, Gonel A, et al. Favorable effects of astaxanthin on brain damage due to ischemia- reperfusion injury. Comb Chem High Throughput Screen. 2020;23(3):214–224. doi:10.2174/1386207323666200219121600.
- Qiu X, Fu K, Zhao X, Zhang Y, Yuan Y, et al. Protective effects of astaxanthin against ischemia/reperfusion induced renal injury in mice. J Transl Med. 2015;13(1):28. doi:10.1186/s12967-015-0388-1.
- Zuluaga Tamayo M, Choudat L, Aid-Launais R, Thibaudeau O, Louedec L, et al. Astaxanthin complexes to attenuate muscle damage after in vivo femoral ischemia-reperfusion. Marine Drugs. 2019;17(6):354. doi:10.3390/md17060354.
- Curek GD, Cort A, Yucel G, Demir N, Ozturk S, et al. Effect of astaxanthin on hepatocellular injury following ischemia/reperfusion. Toxicology. 2010;267(1–3):147–153. doi:10.1016/j.tox.2009.11.003.