Abstract
Background: In this study, we aimed to explore whether the SRY-box transcription factor 9 (SOX9) can play protective roles against the occurrence and development of osteonecrosis of the femoral head (ONFH) by regulating the proliferation, apoptosis, and osteogenic differentiation of human bone marrow stromal cells (hBMSCs) via the Wnt/β-catenin pathway.
Methods: We used 1600 mg of glucocorticoid (GC) to induce hBMSCs to establish an ONFH cell model and performed various experiments. Reverse transcription-quantitative polymerase chain reaction and western blotting assays were used to determine the expression levels of SOX9 and osteoblast markers, such as the RUNX family transcription factor 2 (RUNX2), alkaline phosphatase (ALP), osterix, Wnt3a, and β-catenin. An ALP detection kit was used to measure the ALP activity. Flow cytometry and 3-(4,5-dimethylthiazol-2-yl)-2,5-diphenyltetrazolium bromide assays were performed to determine the cell viability.
Results: GC treatment decreased the expression levels of RUNX2, ALP, and osterix, suppressed ALP activity, and inhibited SOX9 expression. SOX9 overexpression promoted GC-induced cell proliferation and decreased cell apoptosis. Additionally, hBMSCs were transfected with SOX9-small interfering RNA in GC treatment, and SOX9 knockdown was found to suppress the osteogenic differentiation of cells and decrease their viability.
Conclusion: Our results revealed that SOX9 is related to the Wnt/β-catenin pathway in ONFH. Moreover, SOX9 participated in ONFH development by activating the Wnt/β-catenin pathway.
Introduction
Arthritis usually occurs in human joints and surrounding tissues, and is a degenerative inflammatory disease caused by inflammation, infection, degeneration, trauma, or other factors. Clinical manifestations include joint swelling, pain, redness, dysfunction, and deformities [Citation1]. Severe cases can lead to joint disability, affecting the quality of life of the patients. Arthritis can be divided into several types, including rheumatoid arthritis, osteoarthritis, gouty arthritis, ankylosing spondylitis, and reactive arthritis [Citation2,Citation3]. In recent years, an increasing number of people have been diagnosed with osteoarthritis [Citation4,Citation5]. Osteonecrosis of the femoral head (ONFH) is a special type of osteoarthritis and the most serious type of joint degeneration osteonecrosis disease [Citation6,Citation7].
ONFH is a progressive chronic disease that causes disabilities, in which bone cells gradually die and structural changes occur in the bone structure, often resulting in femoral head collapse and hip joint destruction, and affected patients require surgery to remove the sequestrum and total hip arthroplasty (THA) in severe cases [Citation8–10]. Previous trauma and excessive use of corticosteroids are the most common contributing factors to ONFH [Citation11]. Excessive use of steroids disrupts lipid metabolism, damages the vascular endothelium, accumulates fat in the medulla, disrupts osteoblast differentiation, and hinders bone repair and remodeling. THA is often performed to treat the pain and soreness in the middle and late stages of the disease. Advancement of the pharmaceutical industry can aid in the development of a novel approach for the early diagnosis and treatment of steroid-induced necrosis of the femoral head.
At present, glucocorticoid (GC) has been widely used to treat rheumatic, autoimmune, and inflammation dependent diseases [Citation12]. However, the use of GC is one of the most common reasons for ONFH [Citation13]. Studies have confirmed that most patients receiving high-dose GC treatment have clinical symptoms of osteonecrosis within 2 years [Citation14]. In addition, glucocorticoid induced ONFH usually involves young people, and most glucocorticoid induced ONFH patients need surgery, which seriously affects the quality of life of patients [Citation15]. GC can inhibit bone formation by affecting bone cells [Citation12]. Previous research has shown that the crucial determining factor for ONFH is GC-induced cell apoptosis [Citation16,Citation17]. GCs can cause ischemia in various ways, such as the induction of endothelial dysfunction and inhibition of angiogenesis, leading to apoptosis, necrosis, and destruction of the bone structure [Citation18,Citation19]. Therefore, inhibition of apoptosis and enhancement of angiogenesis can prevent the progression of GC-induced ONFH.
SRY-box transcription factor 9 (SOX9) is a transcription factor that regulates the transactivation domain [Citation20]. Several studies have shown that SOX9 is involved in the development of various diseases. Javier et al. reported that SOX9 can act as a therapeutic target for colorectal carcinoma [Citation21]. SOX9 also plays a crucial role in cartilage and bone metabolism [Citation22]. It is expressed in chondrocytes, and it participates in cell differentiation and cartilage formation [Citation23,Citation24]. Song et al. demonstrated that SOX9 is involved in ONFH development [Citation25]. However, the role and mechanism of SOX9 in ONFH remain to be explored.
In this study, we aimed to investigate the role and mechanism by which SOX9 affects the occurrence of ONFH.
Materials and methods
Cell culture and treatment
Human bone marrow stromal cells (hBMSCs) were acquired from the American Type Culture Collection (Manassas, VA, USA). Cells were cultured in 90% α-modified minimal essential medium (Gibco, Grand Island, NY, USA) supplemented with 10% fetal bovine serum (Gibco) at 37 °C in a 5% CO2 humidified incubator. We then established a cell model using 1 mL (1600 mg/mL) glucocorticoid (GC) to treat hBMSCs for 48 h.
Cell transfection
To up-regulate SOX9 expression in hBMSCs, Sox-9CRISPR activating plasmid (SOX9-plasmid, sc-400143-ACT) was obtained from Santa Cruz Biotechnology, and the Control CRISPR Activation Plasmid (control-plasmid, sc-437275) was used as the negative control. To knockdown the SOX9 expression in hBMSCs, SOX9-siRNA (sc-36533) was obtained from Santa Cruz Biotechnology, and the control-siRNA (sc-36869) was used as the negative control. hBMSCs were transfected with control-plasmid, SOX9-plasmid, control-siRNA, or SOX9-siRNA using the Lipofectamine™3000 (Invitrogen, Carlsbad, CA, USA) for 48 h, following the manufacturer’s instructions. RT-qPCR was used to detect the transfection efficiency.
Reverse-transcription-quantitative polymerase chain reaction (RT-qPCR)
After transfection for 24 h, TRIzol reagent (TaKara, Shiga, Japan) was used to isolate the RNA from cells following the manufacturer’s instructions. Next, a quantitative amount of RNA was used to synthesize cDNA using a reverse transcription kit, followed by qPCR with the SYBR Green PCR kit (Vazyme, Nanjing, Jiangsu) using an ABI 7500 Real-Time PCR instrument (ThermoFisher, Waltham, MA, USA). The RT-qPCR reaction procedure was as follows: denaturation at 95 °C for 3 min, denaturation at 95 °C for 15 s, annealing at 60 °C for 30 s, and extension at 72 °C for 40 cycles. Target gene expression was calculated using the 2-ΔΔCt formula.
3-(4,5-dimethylthiazol-2-yl)-2,5-diphenyltetrazolium bromide (MTT) assay
hBMSCs were transfected with the corresponding plasmids or small interfering RNAs (siRNAs) for 24 h and seeded into a 96-well plate. Then, 20 µL MTT (5 mg/mL; Sigma, St. Louis, MO, USA) was added to the wells, and the cells were cultured for an additional 4 h. Finally, the supernatant was removed and 200 µL of dimethyl sulfoxide was added. The absorbance was measured at 570 nm using a microplate reader.
Flow cytometry (FCM) assay
An annexin V-fluorescein isothiocyanate (FITC)/propidium iodide (PI) staining kit was used to detect hBMSC apoptosis (BD Bioscience, San Jose, CA, USA). Briefly, hBMSCs were transfected with different plasmids or siRNAs, and the transfected cells were collected via centrifugation at 1000 g at 4 °C for 5 min. Then, we suspended the cells in 500 µL of the FITC-binding buffer. Next, we added annexin V-FITC and PI solution into the cells based on the standard operating procedure. The cells were gently mixed and incubated for 20 min to protect them from the light. A flow cytometer (BD Technologies, Petaluma, CA, USA) was used to determine the apoptosis rate, and the data were analyzed by Kaluza Analysis (version 2.1.1.20653; Beckman Coulter, Inc.).
Western blotting assay
In order to collect proteins from the transfected cells, we first removed the cell culture medium, washed the cells with PBS, then split the cells with the radioimmunoprecipitation assay (Solarbio, Beijing), and finally the cells were centrifugated at 14000 g for 5 minutes to take the supernatant. Proteins were resolved using 12% sodium dodecyl sulfate-polyacrylamide gel electrophoresis and transferred onto polyvinylidene fluoride membranes. The membranes were blocked with 5% nonfat milk for 2 h and incubated with primary antibodies, including anti-SOX9 (1:1000; Abcam, Cambridge, MA, USA), anti-Wnt3a (1:1000; Abcam), and anti-β-catenin (1:1500; Abcam) antibodies, at 4 °C overnight. They were washed thrice with PBST and incubated with secondary antibodies. Enhanced chemiluminescence was used to detect the signals (Applygen Technologies, Inc., Beijing, China).
Alkaline phosphatase (ALP) activity
An ALP kit was used to determine the ALP activity. The specific operation steps were as follows: cells at a cell density of 1 × 104 cells/mL were seeded into a 96-well plate. After 14 d, the medium was discarded, lysate was added to the 96-well plate, and incubates at 37 °C for 30 min in the dark. Then, 100 μL of the stop reaction solution was added, and absorbance was measured at 405 nm.
Statistical analyses
All experimental results were analyzed using SPSS 11 statistical software (IBM, Armonk, NY, USA). The differences between diverse groups were determined using the Student’s t-test or one-way analysis of variance. Data are represented as the mean ± standard deviation. Statistical significance was set at p < 0.05.
Results
SOX9 expression is downregulated in the GC-induced ONFH cell model
First, hBMSCs were treated with 1600 mg of GCs for 48 h to establish an ONFH cell model in vitro. We determines the expression levels of several osteoblast makers including Runx2, ALP, and Osterix. Results indiated that compared to untreated hBMSCs, the mRNA expression levels of RUNX2, ALP, and osterix were decreased in GC-treated cells (). Morover, ALP activity was found to be reduced in GC-treated cells using an ALP activity detection kit (). These findings imply the successful establishment of ONFH cell model. In addition, SOX9 was weakly expressed at the transcriptional and translational levels in the GC-induced ONFH cell model ().
Figure 1. SRY-box transcription factor 9 (SOX9) expression levels are decreased in glucocorticoid (GC)-induced human bone marrow stromal cells (hBMSCs). (A–C) RUNX family transcription factor 2 (RUNX2), alkaline phosphatase (ALP), and osterix expression levels were determined in untreated and GC-induced hBMSCs via reverse transcription-quantitative polymerase chain reaction (RT-qPCR) analysis. (D) ALP detection kit was used to measure the ALP activity. (E and F) RT-qPCR and western blotting analysis of SOX9 expression. **p < 0.01 vs. control group.
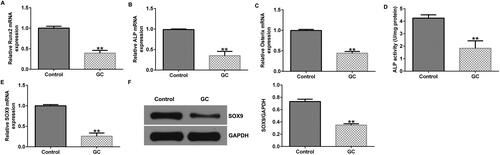
SOX9 overexpression promotes the osteogenic differentiation of GC-treated hBMSCs
We then explored the role of SOX9 on osteogenic differentiation of GC-treated hBMSCs. hBMSCs were induced by GC and transfected with SOX9-plasmid for 48 h. Compared to the control-plasmid group, SOX9 expression was upregulated in the SOX9-plasmid group (). Furthermore, SOX9 levels were decreased in GC-treated hBMSCs than in untreated hBMSCs (). Expression levels of osteoblast makers, such as RUNX2, ALP, and osterix, were significantly decreased in the GC-treated group (). Additionally, ALP activity was reduced in the GC-treated group (). These changes were inhibited by the transfection of the SOX9-plasmid into GC-treated hBMSCs.
Figure 2. SOX9-plasmid improves the osteogenic differentiation of hBMSCs. (A and B) hBMSCs were transfected with the control-plasmid and SOX9-plasmid in the presence of 1600 mg GCs for 48 h, followed by RT-qPCR and western blotting analysis to measure the SOX9 expression levels. (C and D) hBMSCs were divided into four groups: control, GC, GC + control-plasmid, and GC + SOX9-plasmid groups. SOX9 expression levels were determined via RT-qPCR and western blotting. (E–G) RT-qPCR analysis of RUNX2, ALP, and osterix expression levels. (H) ALP activity was examined using a specific kit. **p < 0.01 vs. control-plasmid; ##p < 0.01 vs. control; &&p < 0.01 vs. GC + control-plasmid.
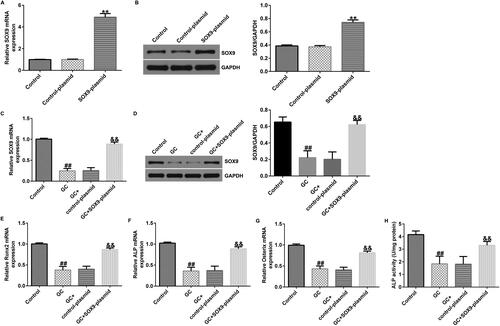
SOX9 overexpression promotes GC-treated hBMSC proliferation and inhibits their apoptosis
After treating with 1600 mg GCs and transfecting with the control-plasmid or SOX9-plasmid, we determined the cell viability of hBMSCs. MTT and FCM assays revealed that, compared to the control group, GC treatment suppressed cell proliferation and promoted apoptosis. However, SOX9-plasmid treatment simultaneously enhanced the cell viability and reduced apoptosis (). Therefore, SOX9-plasmid improved the viability of GC treated hBMSCs.
Figure 3. Upregulation of SOX9 expression promotes the viability of hBMSCs. (A) 3-(4,5-dimethylthiazol-2-yl)-2,5-diphenyltetrazolium bromide (MTT) assay was performed to examine cell proliferation in different groups: control, GC, GC + control-plasmid, and GC + SOX9-plasmid groups. (B and C) Flow cytometry (FCM) assay was performed to examine cell apoptosis. **p < 0.01 vs. control; ##p < 0.01 vs. GC + control-plasmid.
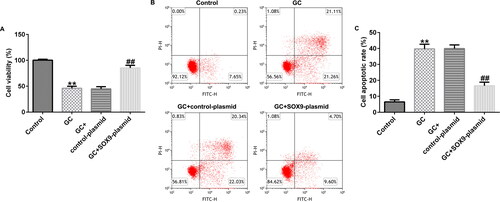
SOX9 knockdown suppresses the osteogenic differentiation of GC-treated hBMSCs
Based on the experimental conclusions drawn from and , we knocked down SOX9 and transfected GC-treated and untreated hBMSCs with SOX9-siRNA. SOX9 expression levels were lower in the SOX9-siRNA group than in the control siRNA group (). GC treatment inhibited SOX9 expression (). GC decreased RUNX2, ALP, and osterix expression levels (). ALP activity was also reduced in the GC-treated group (). In GC and SOX9-siRNA-treated hBMSCs, SOX9-siRNA further decreased SOX9 expression levels (), suppressed the expression of osteoblast-related markers (), and inhibited ALP activity (). Overall, SOX9 knockdown inhibited the osteogenic differentiation of GC-treated hBMSCs.
Figure 4. SOX9-small interfering RNA (siRNA) suppresses the osteogenic differentiation of hBMSCs. (A and B) hBMSCs were transfected with the control-plasmid and SOX9-plasmid in the presence of 1600 mg GCs for 48 h, followed by RT-qPCR and western blotting analysis to determine the transfection efficiency of SOX9-siRNA and expression levels of SOX9. (C and D) SOX9 expression levels were determined via RT-qPCR and western blotting assay in four groups: control, GC, GC + control-siRNA, and GC + SOX9-siRNA groups. (E–G) RT-qPCR analysis of RUNX2, ALP, and osterix expression levels. (H) ALP activity was examined using a specific kit. **p < 0.01 vs. control-siRNA; ##p < 0.01 vs. control; &, &&p < 0.05, 0.01 vs. GC + control-siRNA.
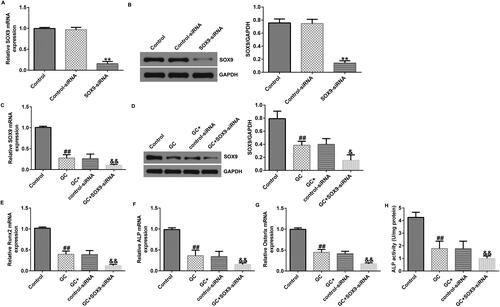
Downregulation of SOX9 expression decreases the viability of GC-treated hBMSCs
Next, we examined the cell proliferation and apoptosis of hBMSCs using MTT and FCM assays. The results demonstrated that compared to the untreated group, GC treatment suppressed the cell proliferation and promoted apoptosis. However, SOX9-siRNA treatment accelerated cell proliferation and apoptosis (). Therefore, SOX9-siRNA inhibited the viability of GC-treated hBMSCs.
Figure 5. SOX9 knockdown inhibits hBMSC proliferation and promotes their apoptosis. (A) MTT assay was used to determine the cell viability in different groups: control, GC, GC + control-siRNA, and GC + SOX9-siRNA groups. (B and C) FCM assay was used to examine the cell apoptosis. **p < 0.01 vs. control; ##p < 0.01 vs. GC + control-siRNA.
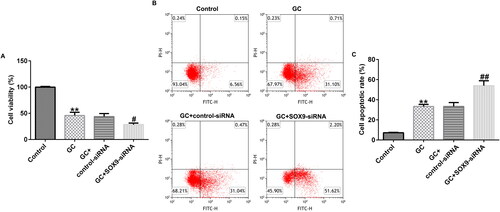
Wnt/β-catenin pathway is involved in the effect of SOX9 on ONFH
Finally, we explored the signaling mechanism of SOX9 in the ONFH cell model. hBMSCs were transfected with the SOX9-plasmid under GC treatment conditions. Results indicated that conmpared with the control group, Wnt3a and β-catenin expression levels were decreased in the GC group, but SOX9-plasmid increased their expression at the mRNA and protein levels (). Notably, SOX9-siRNA transfected into GC-treated hBMSCs exerted the opposite effect. Compared to the GC + control-siRNA group, SOX9-siRNA decreased the expression levels of Wnt3a and β-catenin (). Taken together, SOX9 is associated with the Wnt/β-catenin signaling pathway in ONFH.
Figure 6. Role of SOX9 is associated with the Wnt/beta-catenin pathway in the osteonecrosis of the femoral head (ONFH) in vitro model. (A and D) Western blotting analysis of Wnt3a and β-catenin expression levels. (B and E) RT-qPCR analysis of Wnt3a expression levels. (C and F) RT-qPCR analysis of β-catenin expression levels. **p < 0.01 vs. control; ##p < 0.01 vs. GC + control-plasmid; &&p < 0.01 vs. GC + control-siRNA.
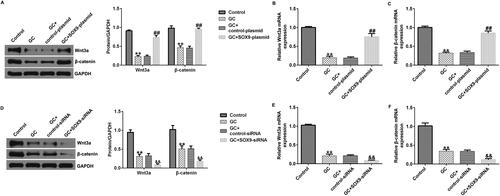
Discussion
When patients with arthritis undergo GC therapy, long-term or excessive steroid use can cause serious side effects, potentially leading to ONFH [Citation26]. ONFH occurs due to insufficient blood supply to the femoral head, leading to apoptosis and necrosis. According to a survey, steroid-induced osteonecrosis accounts for approximately 24% of all cases. THA is currently the only treatment option, and most patients undergo several surgeries during their lifetime. It is not easy to diagnose in the prophase, and there is no obvious change in the histology of the femoral head; however, over time, bone metabolism of the femoral head may be disordered [Citation27]. So we need to look for new targets to cure ONFH.
Hao et al. repoted that microRNAs (miRNAs) and their downstream target genes are involved in ONFH occurrence and development [Citation28]. Wang et al. revealed that the recombinant bone morphogenetic protein 2 (rBMP-2)/Fc treatment was effective in bone formation and beneficial for ONFH treatment [Citation29]. Chen et al. indicated that urine stem cell-derived extracellular vesicles could suppress GC-induced ONFH effects, and tissue inhibitor of metalloproteinase-1 played a crucial role in this process [Citation30]. A report by Okazaki et al. revealed that lipopolysaccharide treatment increased the occurrence of steroid-related ONFH via toll-like receptor 4 [Citation31]. Based on these results, we explored the effects of SOX9 in an ONFH cell model in vitro in this study.
Huang et al. showed that some factors, such as fibroblast growth factor 2, insulin-like growth factor 1, and SOX9, may influence ONFH pathogenesis [Citation32]. SOX9 is involved in early embryonic growth. It is also involved in sex determination and chondrogenesis [Citation25]. Mutations in the upstream sequence of SOX9 gene lead to abnormal chondrogenesis. Li et al. reported that miR-432-5p can act on SOX9 and that SOX9 is involved in gastric cancer development [Citation33]. Tian et al. revealed that SOX9 promotes ovarian cancer cell growth [Citation34]. A previous study showed that SOX9 gene polymorphisms and expression levels were associated with ONFH risk and development, and its expression was downregulated in ONFH [Citation25]. Consistently, in our study, GC treatment suppressed SOX9 expression in an in vitro ONFH cell model. SOX9 overexpression significantly reversed GC-induced hBMSC osteogenic differentiation reduction, while SOX9 knockdown inhibited osteogenic differentiation in GC-induced hBMSCs. Furthermore, SOX9 overexpression improved the viability of GC-induced hBMSCs, and its knockdown suppressed the proliferation of GC-induced hBMSCs.
SOX9 plays important roles in chondrocyte regeneration, joint formation, and fracture repair, mainly via the Wnt signaling pathway [Citation35–37]. BMP and Wnt/β-catenin pathways are required for osteoblast differentiation and synergistic control of bone formation, and Wnt signaling stimulates the differentiation of MSCs into osteogenic progenitors [Citation38]. Wnt/β-catenin signaling pathway plays a crucial role in osteoblast differentiation. In our study, we found that SOX9 overexpression promoted Wnt3a and β-catenin expression in GC-induced hBMSCs, but SOX9 knockdown had the opposite effect. Therefore, SOX9 plays an important role in ONFH via the Wnt/β-catenin signaling pathway.
Taken together, this study shows that SOX9 promotes osteogenic differentiation and the proliferation of hBMSCs through activating Wnt/β-catenin pathway, thereby inhibiting the progression of ONFH. These findings encourage SOX9 as a clinical biomarker and potential therapeutic target of ONFH, and provide more theoretical basis for the development of treatment plans for ONFH. However, there were also some limitations of the current study. Firstly, this investigation focus on the role of SOX9 in ONFH in vitro, but not in vivo, and there are great differences between in vitro experiences and human diseases. Besides, although we have proved that Wnt/β-catenin pathway participates in the role of SOX9 in ONFH cell model, the interaction between SOX9 and Wnt/β-catenin pathway still needs further exploration (e.g. E.g. the use of Wnt/β-catenin signaling pathway inhibitors). We will further investigate these in our next research.
Conclusion
Overall, this study revealed that SOX9 inhibits ONFH progression by promoting the osteogenic differentiation and proliferation of hBMSCs via the activation of the Wnt/β-catenin pathway. SOX9 may be a potential therapeutic target of ONFH.
Authors’ contributions
Xiangsheng Meng contributed to data collection, statistical analysis, data interpretation and manuscript preparation. Haiquan Zhu contributed to data collection and data interpretation. All authors have read and approved the final manuscript.
Disclosure statement
The authors declare that they have no competing interests.
Data availability statement
The datasets used and/or analyzed during the current study are available from the corresponding author on reasonable request.
References
- Abramoff B, Caldera FE. Osteoarthritis: pathology, diagnosis, and treatment options. Med Clin North Am. 2020;104(2):1–8. doi:10.1016/j.mcna.2019.10.007.
- Shepherd J, Cooper K, Harris P, Picot J, Rose M. The clinical effectiveness and cost-effectiveness of abatacept, adalimumab, etanercept and tocilizumab for treating juvenile idiopathic arthritis: a systematic review and economic evaluation. Health Technol Assess. 2016;20(34):1–222. doi:10.3310/hta20340.
- Peat G, Thomas MJ. Osteoarthritis year in review 2020: epidemiology & therapy. Osteoarthritis Cartilage. 2021;29(2):180–189. doi:10.1016/j.joca.2020.10.007.
- Katz JN, Arant KR, Loeser RF. Diagnosis and treatment of hip and knee osteoarthritis: a review. JAMA. 2021;325(6):568–578. doi:10.1001/jama.2020.22171.
- George G, Lane JM. Osteonecrosis of the femoral head. J Am Acad Orthop Surg Glob Res Rev. 2022;6:e21.00176.
- Cohen-Rosenblum A, Cui Q. Osteonecrosis of the femoral head. Orthop Clin North Am. 2019;50(2):139–149. doi:10.1016/j.ocl.2018.10.001.
- Zhao D, Zhang F, Wang B, et al. Guidelines for clinical diagnosis and treatment of osteonecrosis of the femoral head in adults (2019 version). J Orthop Translat. 2020;21:100–110. doi:10.1016/j.jot.2019.12.004.
- Petek D, Hannouche D, Suva D. Osteonecrosis of the femoral head: pathophysiology and current concepts of treatment. EFORT Open Rev. 2019;4(3):85–97. doi:10.1302/2058-5241.4.180036.
- Hoggard TM, Chen DQ, Quinlan ND, Bell JE, Werner BC, Cui Q. Outcomes following total hip arthroplasty for osteonecrosis of the femoral head in patients on hemodialysis. J Bone Joint Surg Am. 2022;104(Suppl 2):90–94. doi:10.2106/JBJS.20.00352.
- Zhao D, Xie H, Xu Y, et al. Management of osteonecrosis of the femoral head with pedicled iliac bone flap transfer: a multicenter study of 2190 patients. Microsurgery. 2017;37(8):896–901. doi:10.1002/micr.30195.
- Zhang YL, Yin JH, Ding H, Zhang W, Zhang CQ, Gao YS. Vitamin K2 prevents glucocorticoid-induced osteonecrosis of the femoral head in rats. Int J Biol Sci. 2016;12(4):347–358. doi:10.7150/ijbs.13269.
- Chang C, Greenspan A, Gershwin M. The pathogenesis, diagnosis and clinical manifestations of steroid-induced osteonecrosis. J Autoimmun. 2020;110:102460.
- Xie Y, Hu JZ, Shi ZY. MiR-181d promotes steroidinduced osteonecrosis of the femoral head by targeting SMAD3 to inhibit osteogenic differentiation of hBMSCs. Eur Rev Med Pharmacol Sci. 2018;22:4053–4062.
- Zhao DW, Yu M, Hu K, et al. L. Prevalence of nontraumatic osteonecrosis of the femoral head and its associated risk factors in the Chinese population: results from a nationally representative survey. Chin Med J. 2015;128(21):2843–2850. doi:10.4103/0366-6999.168017.
- Gao Y, Zhu H, Wang Q, Feng Y, Zhang C. Inhibition of PERK signaling prevents against glucocorticoid-induced endotheliocyte apoptosis and osteonecrosis of the femoral head. Int J Biol Sci. 2020;16(4):543–552. doi:10.7150/ijbs.35256.
- Nie Z, Chen S, Peng H. Glucocorticoid induces osteonecrosis of the femoral head in rats through GSK3β-mediated osteoblast apoptosis. Biochem Biophys Res Commun. 2019;511(3):693–699. doi:10.1016/j.bbrc.2019.02.118.
- Fan ZQ, Bai SC, Xu Q, et al. Oxidative stress induced osteocyte apoptosis in steroid-induced femoral head necrosis. Orthop Surg. 2021;13(7):2145–2152. doi:10.1111/os.13127.
- Güler-Yüksel M, Hoes JN, Bultink IEM, Lems WF. Glucocorticoids, inflammation and bone. Calcif Tissue Int. 2018;102(5):592–606. doi:10.1007/s00223-017-0335-7.
- González-Martín JJ, Novella-Navarro M, Calvo-Aranda E, et al. Endothelial dysfunction and subclinical atheromatosis in patients with systemic sclerosis. Clin Exp Rheumatol. 2020;38:48–52.
- Song H, Park KH. Regulation and function of SOX9 during cartilage development and regeneration. Semin Cancer Biol. 2020;67:12–23. doi:10.1016/j.semcancer.2020.04.008.
- Javier BM, Yaeger R, Wang L, et al. Recurrent, truncating SOX9 mutations are associated with SOX9 overexpression, KRAS mutation, and TP53 wild type status in colorectal carcinoma. Oncotarget. 2016;7(32):50875–50882. doi:10.18632/oncotarget.9682.
- Akiyama H. Transcriptional regulation in chondrogenesis by SOX9. Clinical Calcium. 2011;21:845–851.
- Tamamura Y, Katsube K, Mera H, Itokazu M, Wakitani S. Irx3 and Bmp2 regulate mouse mesenchymal cell chondrogenic differentiation in both a Sox9-dependent and-independent manner. J Cell Physiol. 2017;232(12):3317–3336. doi:10.1002/jcp.25776.
- Cucchiarini M, Orth P, Madry H. Direct rAAV SOX9 administration for durable articular cartilage repair with delayed terminal differentiation and hypertrophy in vivo. J Mol Med (Berl). 2013;91(5):625–636.
- Song Y, Du ZW, Ren M, et al. Significant associations of SOX9 gene polymorphism and gene expression with the risk of osteonecrosis of the femoral head in a Han Population in Northern China. Biomed Res Int. 2016;2016:1–11. doi:10.1155/2016/5695317.
- Wang XS, Zhuang QY, Weng XS, Lin J, Qian WW. Etiological and clinical analysis of osteonecrosis of the femoral head in Chinese patients. Chin Med J (Engl). 2013;126:290–295.
- Sun H, Zhang W, Yang N, et al. Activation of cannabinoid receptor 2 alleviates glucocorticoidinduced osteonecrosis of femoral head with osteogenesis and maintenance of blood supply. Cell Death Dis. 2021;12(11):1035. doi:10.1038/s41419-021-04313-3.
- Hao YQ, Lu C, Zhang BG, Xu ZC, Guo H, Zhang GK. Identifying the potential differentially expressed miRNAs and mRNAs in osteonecrosis of the femoral head based on integrated analysis. CIA. 2021;16:187–202. doi:10.2147/CIA.S289479.
- Wang CH, Zang HM, Zhou DS. Bone morphogenetic protein-2 exhibits therapeutic benefts for osteonecrosis of the femoral head through induction of cartilage and bone cells. Exp Ther Med. 2018;15:4298–4308.
- Chen CY, Du W, Rao SS, et al. Extracellular vesicles from human urine-derived stem cells inhibit glucocorticoid-induced osteonecrosis of the femoral head by transporting and releasing pro-angiogenic DMBT1 and anti-apoptotic TIMP1. Acta Biomater. 2020;111:208–220.
- Okazaki S, Nagoya S, Matsumoto H, et al. TLR4 stimulation and corticosteroid interactively induce osteonecrosis of the femoral head in rat. J Orthop Res. 2016;34(2):342–345. doi:10.1002/jor.23008.
- Huang G, Zhao G, Xia J, et al. FGF2 and FAM201A affect the development of osteonecrosis of the femoral head after femoral neck fracture. Gene. 2018;652:39–47. doi:10.1016/j.gene.2018.01.090.
- Li H, Zhou X, Yu Z, Tian Y. Circ_0075825 promotes gastric cancer progression via adsorbing miR-432-5p to modulate SOX9. Clinics (Sao Paulo). 2022;77:100018. doi:10.1016/j.clinsp.2022.100018.
- Tian H, Hou L, Xiong Y, Cheng Q. Dexmedetomidine upregulates microRNA-185 to suppress ovarian cancer growth via inhibiting the SOX9/Wnt/β-catenin signaling pathway. Cell Cycle. 2021;20(8):765–780. doi:10.1080/15384101.2021.1897270.
- Topol L, Chen W, Song H, Day TF, Yang Y. Sox9 inhibits Wnt signaling by promoting β-catenin phosphorylation in the nucleus. J Biol Chem. 2009;284(5):3323–3333. doi:10.1074/jbc.M808048200.
- Feng L, Yang ZM, Li YC, et al. Linc-ROR promotes mesenchymal stem cells chondrogenesis and cartilage formation via regulating SOX9 expression. Osteoarthr Cartil. 2021;29(4):568–578. doi:10.1016/j.joca.2020.12.020.
- He X, Bougioukli S, Ortega B, Arevalo E, Lieberman JR, McMahon AP. Sox9 positive periosteal cells in fracture repair of the adult mammalian long bone. Bone. 2017;103:12–19. doi:10.1016/j.bone.2017.06.008.
- Chen XJ, Shen YS, He MC, et al. Polydatin promotes the osteogenic differentiation of human bone mesenchymal stem cells by activating the BMP2-Wnt/β-catenin signaling pathway. Biomed Pharmacother. 2019;112:108746. doi:10.1016/j.biopha.2019.108746.