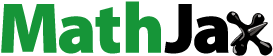
Abstract
Aims
To validate the hypothesis that hepatic vein ligation (HVL) alone may produce similar results to liver venous deprivation (LVD or HVL + portal vein ligation [PVL]).
Methods
Rats were assigned to five groups, namely, the control group; the R group in which the right median hepatic vein (RMHV) was ligated; the M group in which the middle median hepatic vein (MMHV) was ligated; the RM group in which both the RMHV and MMHV were ligated (R + MMHVL, extended ligation of the hepatic veins); and the LVD group in which both the right median portal vein and the RMHV were ligated. The liver hypertrophy effect and liver enzymes were determined. Methylene blue staining and retrograde pressurized perfusion assays were performed to investigate the hemodynamic changes.
Results
The RM and LVD groups exhibited similar significant hypertrophy in the future liver remnants when compared to that of the control group, and almost no additional hypertrophy effect was observed in the R and M groups. There was a remarkable elevation in serum transaminase levels in both groups. The methylene blue staining experiment indicated that pressure-dependent collaterals formed between the contiguous drainage areas, and the R + MMHVL procedure blocked the outflow of the right median lobe.
Conclusion
Extended ligation of the hepatic vein (R + MMHVL) resulted in a similar hypertrophy effect and hepatic damage to those of LVD (HVL + PVL) treatment in a rat model, and intrahepatic venovenous collaterals play key roles.
1. Background
Sufficient future liver remnants (FLR) is a necessary condition for curative hepatectomy.Citation1 There are a variety of methods that surgeons can choose to improve the FLR when the FLR is inadequate.Citation2–4 At present, the clinically used procedures to induce hypertrophy of the FLR volume include portal vein embolization or ligation (PVE or PVL), associating liver partition and PVL for staged hepatectomy (ALPPS), and liver venous deprivation (LVD) and their variants.Citation3,Citation5–7 However, the risks and benefits of some of these methods are controversial, and their advantages and limitations need to be further studied.
Among them, LVD was introduced by Boris Guiu’s team and has attracted much attention in recent years.Citation6,Citation7 LVD is an effective and safe method that simultaneously combines both portal and hepatic vein embolization (PVE + HVE) during the first-stage operation. Right PVE is completed by using a lipiodol-glue mixture through transhepatic access under ultrasound guidance, and right HVE is achieved by placing a vascular plug at the distal part of the hepatic vein (HV) after ultrasound puncture.Citation6,Citation7 Both animal experiments and retrospective studies have compared LVD to PVE and suggest that LVD shows a higher efficacy in inducing hypertrophy of the FLR while exhibiting fewer postoperative complications in the following hepatectomy compared to those of PVE.Citation8–Citation15
Many mechanisms have been shown to be involved in liver hypertrophy stimulated by LVD, and one widely recognized mechanism is the redistribution of portal blood flow to the non-injured liver.Citation4,Citation5 Specifically, the PVE procedure interrupts portal blood flow and directly increases portal vein pressure, consequently increasing portal blood flow into the contralateral liver lobe. In that case, obstruction of the hepatic outflow can also lead to regurgitation into the portal vein through the sinusoids, which can indirectly increase the portal pressure and promote the perfusion of portal blood to the non-embolized lobe in theory.Citation4,Citation5
Therefore, we propose the hypothesis that HVE alone may produce similar results to LVD (HVE + PVE). HVE is a much simpler technique than LVD. In this study, the rat model of LVD is developed by ligating the RMPV and RMHV at same time (RMPVL + RMHVL), and this method has been described in our previous study.Citation8 Then, we compared the hepatic hypertrophy effect and liver damage between HVL and LVD (HVL + PVL) treatment.
2. Materials and methods
2.1. Animals
All procedures and housing of the animals were performed in accordance with Chinese animal regulations and guidelines. This study was approved by the Ethics Committee of the Animal Experiment Center of Wuhan University. Male Sprague–Dawley (SD) rats weighing ∼350 g from Hunan SJA (Changsha, China) were housed under specific pathogen-free conditions with controlled temperature (23 °C) and a light/dark (12 h:12 h) cycle. Animals were provided with standard rat food and water. The surgeries were conducted after a 1-week adjustment period.
2.2. Experimental design
The left median lobe (LML) was designated as the FLR in the experimental groups. Specifically, a total of 60 rats were randomly assigned to five groups (n = 12 in each group, and ): the control group; the R group in which the right middle hepatic vein (RMHV) was ligated; the M group in which the middle median hepatic vein (MMHV) was ligated; the RM group in which both the right and middle median hepatic veins (R + MMHV) were ligated; the LVD group in which both the right hepatic vein (RMHV) and the right median portal vein (RMPV) were ligated. Because the portal vein branch supplying the caudate lobe (CL) is too small to dissect, the CLs of all the rats were removed. In addition, because the left lateral lobe (LLL) and right lateral lobe (RLL) account for a large proportion of the whole liver, the portal vein supplying the LLL and RLL was ligated.
Figure 1. Schematic of the different rat models. (A) Portal (left) and hepatic (right) vein anatomy of the RML and LML are displayed, the dotted line indicated the boundaries of drainage areas of different HVs. (B) The second hepatic hilum of rat liver. The posterior vena cava, RMHV and common trunk were presented. (C) The MMHV were obstructed with suturing ligation, the needle entry and exit points were shown. (D) Schematics of the R, M, RM and LVD groups are described, respectively.
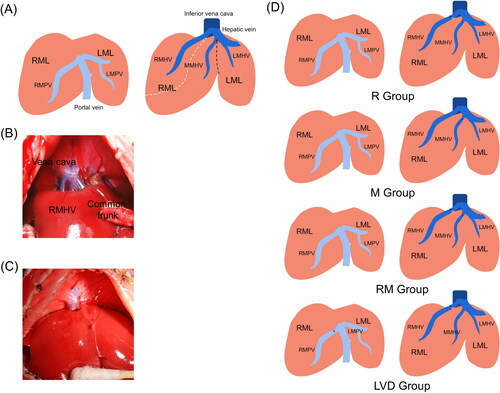
Table 1. Experimental designation.
2.3. Anesthesia and surgical procedures
Animals were anesthetized by inhalation of a mixture of 3% isoflurane (RWD, Shenzhen, China) mixed with pure oxygen at a flow rate of 0.5 L/min. The hair was shaved, and the abdominal skin was disinfected. Then, a 5.0-cm midline incision was made. The falciform ligament was released to fully expose the HVs (). After the operation, each animal was injected with 6 mL of a 5% glucose and sodium chloride solution containing 0.96 mg of gentamicin. Animals were placed on a heating pad to keep warm before recovery.
PVL: The RPV was ligated or sutured with 5-0 nylon thread. Damage to the accompanying bile duct and artery was avoided during this procedure.
HVL: The RMHV is apparent and easily dissected and ligated. In rats, the MMHV confluences into the left median hepatic vein (LMHV) and is embedded in the liver parenchyma. Dissection of the MMHV would injure the vena cava or the MMHV and lead to uncontrollable bleeding, so we chose to suture with 5–0 nylon thread, and choosing the right angle and depth was key to successful suturing ().
2.4. Sample collection
At postoperative days (PODs) 2 and 7, the animals (n = 6 at each time point) were sacrificed, blood samples were collected into anticoagulative tubes (BD Biosciences, America), and serum was isolated by centrifugation and stored at −20 °C. The livers were explanted with no accessory vessels or ligaments. Each individual liver lobe was weighed and recorded. Because each liver lobe compared to body weight in normal healthy SD rats is constant, our previous data showed that the LML/body weight is approximately 0.00376, so the LML growth ratio was calculated using the following formula:
2.5. Liver injury assessment
Serum assessments related to liver function, including aspartate aminotransferase (AST), alanine aminotransferase (ALT), total bilirubin (T-Bil), albumin (ALB), and the prothrombin time (PT), were examined using an automatic procedure in a serum multiple biochemical analyzer (Siemens, Germany). Whole blood was analyzed with an automatic blood cell analyzer (Japan, Nihon Kohden).
2.6. Pathological examination
The harvested liver lobes (LML and RML) were fixed in 4% buffered formalin for 48 h. After paraffin embedding, these tissues were cut into slices (4-mm thick) to prepare slides. Standard hematoxylin-eosin (H-E) staining was conducted to observe the ultrastructural changes. Picric acid-Sirius red staining was performed to visualize liver mesangial collagen fibers. Additionally, Ki-67 (Proteintech, Wuhan, China) immunostaining was performed to evaluate hepatocyte regeneration. All sections were digitalized with a Slide Scanner (Hamamatsu Electronic, Japan). The number of Ki-67-positive hepatocytes was determined by counting 1000 hepatocytes in five randomly selected visual fields (20×).
2.7. Methylene blue staining assay
The methylene blue staining assay was performed to indicate the collaterals between the conterminous drainage area of the HVs.Citation9 Specifically, the different models were completed as described before, but the CL, RL, LLL and their supplying vessels were reserved. Then, 100 µL of a solution of methylene blue was injected into the liver, and the needle eye was pressed with cotton or closed with a surgical cautery pencil. The puncture site was chosen at the middle of the drainage basin of the HV. After 30 s, the dyed range was observed and recorded.
2.8. Retrograde pressurized perfusion assay
Posterior vena cava puncture and HV catheterization were conducted first (Figure S1), and the conduit was placed in the RMHV. Then, the RMHV and MMHV were ligated. The methylene blue solution was injected into the RMHV, and the dyed range was observed with increasing injection pressure.
2.9. Statistical analysis
All quantitative data are presented as the mean values plus standard deviations (means ± SDs). GraphPad Prism 6.0 software (San Diego, CA) was used for graphs and statistical analyses. Multiple t test or Student’s t test was applied to compare the differences among multiple groups or between two groups, respectively. The level of statistical significance was set at p < .05.
3. Results
3.1. Experimental designation
The vascular system and shape of the RML and LML in rats structurally mimic those of the right and left hemiliver in humans, respectively, so they were selected as the observed objects, and the LML was designated as the FLR in the experimental groups (). As described in , the RMHV, MMHV, and LMHV have independent and clear drainage areas (DAs), which are termed the drainage area of right median hepatic vein (DAR), drainage area of middle median hepatic vein (DAM), and drainage area of left median hepatic vein (DAL), respectively. Different groups received different interventions, which are presented in and . We proposed a simple and safe method to ligate the MMHV ().
3.2. Macroscopic findings
As shown in , immediately after ligation of the RMHV or MMHV in the R, M and RM groups, an apparent demarcation line was observed between the obstruction zone and normal liver, and the area of dark red discoloration indicated the drainage territory of the HV. In the LVD group, all the RMLs first turned from a bright red color to a dull red after ligation of the RMPV, and then the DAM turned dark red after ligation of the RMHV.
Figure 2. Liver remodeling after the different surgeries. (A) Changes in the appearance of the live ML after surgery on postoperative days (PODs) 0, 2 and 7. (B) Changes in the body weight at each time point. (C) Weight of the remnant liver/body weight evolution. (D) The LML growth ratio of different groups were shown. (E) The LML/RML shows the morphological changes in the ML after surgery (*, RM vs. Control; #, LVD vs. Control. * or #, P < 0.05; *** or ###, P < 0.001).
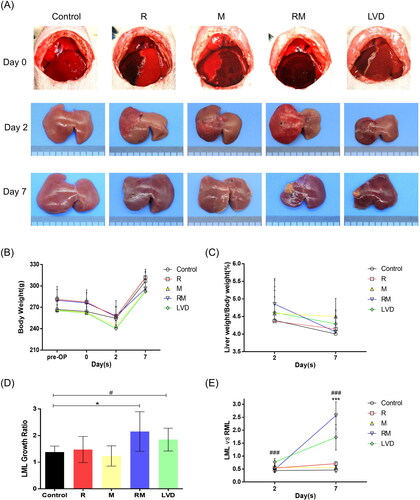
At POD 2, the color of the congestive area was reduced, and the borderlines between the normal and obstructive areas were distinguishable and remained at the original positions in all the experimental groups (). At POD 7, the obstructive zones in the R and M groups almost completely recovered to normal, and the dividing or marked lines had disappeared. The edges of all the liver lobes were blunt, which indicated regeneration of the liver. In contrast, the DARs in the RM and LVD groups were shrunken, hard, pale and yellow, indicating complete necrosis, and the DAMs were atrophied to some extent. Notably, the FLRs (LML) in the RM and LVD groups were hypertrophied ().
All the rats survived the surgical procedures; they suffered from rapid body weight loss during the first 2 days after surgery and then gradually recovered (), which led the liver/body weight ratios reaching a peak at POD 2 and then falling (). The proliferation extent of the FLRs (LML) was quantitatively analyzed by the growth ratio. As seen in , the LMLs were hypertrophic in all the groups (LML growth ratio >1), but the LML/RML ratio in the control, R and M groups remained stable or exhibited small changes, implying that the proliferation of the LML and RML was synchronous, which might be spurred by the removal of the CL and PVL of the other liver lobes. In contrast, the LMLs in the RM and LVD groups were significantly proliferated when compared to those of the control group (2.54 ± 0.74, 1.85 ± 0.43 vs. 1.38 ± 0.23, p < .05). Moreover, the LML/RML ratio significantly increased to 2.57 ± 0.53 and 2.73 ± 0.49, respectively (p < .001). It was suggested that the proliferation of the LML in the RM and LVD groups was provoked by procedures other than the removal of the CL and PVL of the other liver lobes. There was no significant difference between the RM and LVD groups at different time points.
3.3. Microscopic findings
Both the LMLs and RMLs in each group were subjected to tissue microarray analyses (). Pictures of LMLs are shown in . Enlarged and crowded hepatocytes were observed in both groups but not in the control group. The liver regenerative responses were measured by staining for Ki-67, a classical marker of cell proliferation. The expression of Ki-67 in the RM and LVD groups was significantly higher than that in the control, R, and M groups at POD 2 (73.57 ± 8.88, 74.23 ± 7.67 vs. 15.40 ± 2.83, 37.05 ± 11.16, 42.54 ± 8.52, p < .001), and there was no difference between the RM and LVD groups (p > .05).
Figure 3. Histologic and immunohistochemical assessment. (A) The LMLs were subjected to a tissue chip analysis. Slides were stained with H-E (upper) and Ki-67 (lower). Quantification of the number of Ki-67-positive cells in the LML after surgery in all of the different groups (DAM were subjected to the histology analysis. The white, red and black arrows indicate the viable hepatocyte islands, necrotic area and fibrous tissues, respectively) (*** or ###, P < 0.001). (B) The RMLs (congestive liver tissues) of the different groups exhibited different changes; thus, the representative images of H&E-staining were presented, respectively. Collagen deposition was detected by Sirius red staining. In the RM and LVD group at POD7, the DAR were necrotic.
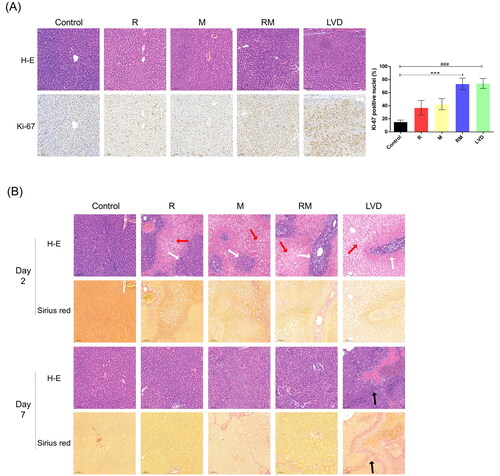
The RML could be divided into two parts according to the drainage area of the HVs: DAR and DAM (). The DARs in the RM and LVD groups were totally necrotic at POD 7, so the DAMs in the RM and LVD groups were analyzed (). Large areas of confluent pericentral necrosis and scattered viable portal hepatocyte islands were observed in the R, M, RM and LVD groups on POD 2 (). Surprisingly, the initial obstructive area was almost recovered, and only a small amount of scar tissue and fibrosis were found in the R and M groups on POD 7, similar to the DAM in the RM group. In contrast, the DAM was replaced with fibrosis tissue in the LVD group (). These results suggested that the congestive lobes in the LVD group suffered from more complete and severe necrosis as well as scar repair.
3.4. Laboratory tests
The serum ALT, AST, T-Bil, ALB, and PT levels and platelet counts were examined to reflect the impairment of liver function. The levels of both ALT and AST were elevated in the control, R, M, RM and LVD groups on POD 2 (162.50 ± 107.99 vs. 149.33 ± 93.19, 236.50 ± 67.44, 254.50 ± 223.76, and 217.00 ± 74.33 U/L, p > 0.05; 567.00 ± 198.81 vs. 395.17 ± 120.13, 709.33 ± 415.28, 614.33 ± 475.52, and 650.83 ± 250.07 U/L, p > .05), and they returned to normal values on POD 7 (). T-Bil levels moderately increased after surgery (2.03 ± 1.26 vs. 3.98 ± 5.51, 3.32 ± 2.75, 3.63 ± 3.03, and 3.55 ± 1.86 µmol/L, p > .05) and declined on POD 7 (). The RM and LVD groups showed more prolonged PT than the control, R, and M groups on POD 2 and returned to the baseline level on POD 7 (16.63 ± 0.52 s vs. 17.75 ± 0.51, 18.11 ± 0.66, 19.56 ± 1.69, 19.13 ± 1.35 s, p < .05, ). ALB levels exhibited the opposite trend and declined to 29.67 ± 1.59 g/L and 27.50 ± 3.09 g/L in the RM (31.26 ± 0.95 g/L, p > 0.05) and LVD (31.26 ± 0.95 g/L, p < 0.05) groups, respectively (). The ALB levels in the RM and LVD groups did not return to normal even on POD 7. No significant difference was observed between the RM and LVD groups at different time points. The preoperative PLT count is an independent risk factor for posthepatectomy liver failure. As shown in , the PLT count in the RM and LVD groups decreased greatly compared to that in the control, R and M groups on POD 2 (162.50 ± 107.99 vs. 149.33 ± 93.19, 236.50 ± 67.44, 254.50 ± 223.76, and 217.00 ± 74.33 U/L, p > .05; 567.00 ± 198.81 vs. 395.17 ± 120.13, 709.33 ± 415.28, 614.33 ± 475.52, and 650.83 ± 250.07 U/L, p > .05), and the counts returned to normal levels on POD 7.
3.5. Methylene blue staining
The range of methylene blue dye could mark the flow direction of blood outflow (). In the control group, blue dye was injected into the DAR and DAM. It was observed that the blue dye immediately flowed in the direction of the HV and hardly crossed the corresponding drainage area. After ligation of the RMHV or MMHV, methylene blue flowed from the obstructive areas to the contiguous normal area in the R, M, and LVD groups. This phenomenon suggests that a bidirectional opening exists bilaterally between the two adjacent DAs. Interestingly, after ligation of both the RMHV and MMHV in the RM group, the injected blue dye flowed from the DAM to the DAL but hardly flowed from the DAR to the DAM.
Figure 5. Methylene blue staining and retrograde pressurized perfusion assay. (A) The RMHV, MMHV or both the RMHV and MMHV were obstructed, then injected the methylene blue solution into the center of different DRs. The dyeing area indicated the direction of blood flow. (B) After the occlusion of both the RMHV and MMHV, the methylene blue was retrogradely perfused into the RMHV. These photos presented the liver appearance before (left) and after (right) occlusion of hepatic pedicle, respectively. The hepatic-portal venous reflux was observed and the LLL turned blue (white arrow). After the hepatic pedicle was occluded with vascular forceps and continue to push the methylene blue, part of DAM were dyed with methylene blue (green arrow).
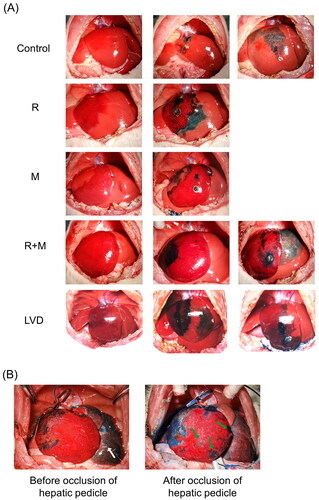
The above phenomenon indicated that the bilateral opening might be pressure controlled, so a retrograde pressurized perfusion assay was performed (). With increasing injection pressure, the DAR was first dyed with methylene blue, and then hepatic-portal venous reflux was observed. Next, the undisturbed liver lobes, including the LLL, LML and RL, turned blue, while the DAM maintained a dull red appearance. To further increase the pressure of the RMHV, the hepatic pedicle was occluded with vascular forceps. Continuing to push the methylene blue, the total RML was apparently swollen and enlarged, and part of the DAM was dyed with methylene blue.
4. Discussion
LVD is a novel technique to increase the FLR, and many clinical studies have proven that it is more effective than PVE.Citation10–15 However, the mechanism of liver hyperplasia caused by LVD has not been clearly elucidated.Citation4,Citation5 At present, the popular view on LVD is that PVE can occlude the inflow of the portal vein. In addition, HVE restricts the inflow of the hepatic artery by blocking blood outflow and mitigating the arterial buffer effect led by PVE; as a result, more blood from the portal vein and hepatic artery is redistributed to the contralateral liver.Citation2–5 In fact, the pressure of the portal vein is significantly lower than that of the hepatic artery (6 ± 2 vs. 110 ± 20 mmHg in humans, 7.8 ± 1.1 vs. 124 ± 18 mmHg in ratsCitation16,Citation17) According to the above view, HVE could also strongly limit the inflow of portal blood if HVE could restrict the inflow of the hepatic artery. Thus, could HVE alone achieve the same effect as LVD?
In fact, sacrifice of the major HV is sometimes inevitable in hepatic segmentectomy, hepatic trauma or adult-to-adult living donor liver transplantation, although the liver function per unit volume in the hepatic veno-occlusive area is approximately 40% of that in the unaffected areas at an early stage, and serious atrophy or necrosis does not frequently occur in the preserved segment.Citation18–21 Furthermore, both our and previous rat experiments have also found that the RML could almost be completely restored to a normal state after ligation of the RMHV alone on POD 7, and there was no obvious hyperplasia in other liver lobes.Citation8,Citation22 In summary, the liver showed good tolerance for the regional obstruction of the HV. While the proliferation of the FLR was always accompanied by lesions of other lobes, obviously, the occlusion of only one HV alone cannot induce hypertrophy of the contralateral liver.
However, whether the ligation of two HV branches at the same time could result in damage to the congested area and stimulate the proliferation of the non-congested area remains unknown. As shown in and , occlusion of RMHV or MMHV alone did not cause atrophy of the occlusive area, which was consistent with the previous experimental results. In contrast, the extended HVL (R + MHVL) resulted in necrosis of part of the RML and proliferation of the FLR (LML), which can achieve the same hypertrophy effect as LVD. In addition, extended HVL and LVD exert more serious damage on liver function than that induced by other procedures ( and ). However, there was no significant difference between the RM and LVD groups ( and ).
Next, a methylene blue staining experiment was carried out to investigate the mechanism behind the above phenomenon. As shown in , the blue dye could flow from congestive areas to non-congestive areas when only one HV was ligated, which indicated that intrahepatic venovenous shunts existed in the two adjacent DAs. The existence of intrahepatic venovenous bilateral vessels in humans and animals has been recognized for a long time.Citation23 This assay indicated the following two characteristics of these collateral pathways: 1. two-way opening, where blood always flows from the congested area to the normal area without a specific direction; and 2. real-time opening (). The opening of the collateral can be detected within a few seconds after ligation of the HV.
Figure 6. Schematic illustration of intrahepatic venovenous collaterals. There exist several intrahepatic venovenous collaterals between the adjacent hepatic venous drainage areas. This assay indicated the two characteristics of these collateral pathways: 1. Two way opening; 2. Timely opening; 3. Pressure-dependent.
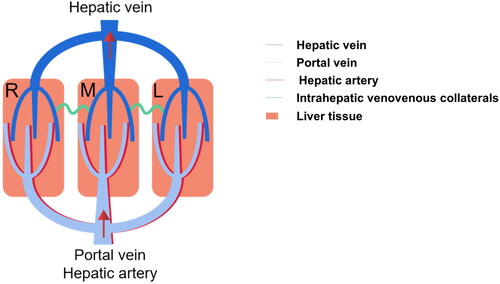
However, it should be emphasized that methylene blue could not flow between two adjacent congestive areas when both the RMHV and MMHV were ligated. Obviously, the intrahepatic venovenous pathway between the DAM and DAR in the RM group was closed, which aggravated blood stasis and eventually led to necrosis of the DAR. A hypothesis for this phenomenon was proposed: the opening of venovenous collaterals is pressure dependent. Only once the pressure difference between the two adjacent areas of HVs exceeds a threshold will the traffic branches open. Then, a compression experiment was conducted to prove the above hypothesis. As expected, as the pressure of the RMHV increases, methylene blue in the DAR begins to flow to the DAM (). Apparently, although the pressures of the RMHV and MMHV were elevated when they were ligated, the pressure gradient between the two congestion areas was too low to open the venovenous bilateral.
This experiment preliminarily indicated that the extended HVL (R + MMHVL) may obtain a similar effect to LVD (RMHVL + RMPVL) in a rat model and has great significance in developing new techniques to increase the FLR. According to previous reports, LVD was performed by ultrasound-guided liver puncture to complete PVE and HVE.Citation6 In fact, this operation is technically difficult and requires experienced doctors to perform. Moreover, there is a great risk of bleeding because after LVD, part of the liver is congested and swollen. Bleeding at the liver puncture point is almost inevitable if the puncture point is in the congested area. This situation was fully observed when methylene blue was injected into the congestion area. However, the implementation of HV occlusion can be completed by radiation intervention or intubation through the internal jugular vein or femoral vein and then placing the vascular plug at the HV. Extended HVE is simpler than the LVD technique, which may make it easy to popularize, and it avoids the risk of liver bleeding.
In this experiment, the intrahepatic venovenous shunt was observed in almost all the rats. However, only 36.3% of patients (94/259) had varying degrees of intrahepatic venovenous shunts when the wedged hepatic venous pressure in patients with cirrhotic portal hypertension was examined.Citation24 In fact, other research has found that intrahepatic venovenous shunts occurred less frequently in cirrhotic patients, which may explain the difference between cirrhosis patients and healthy rats.Citation25 However, it was also implied that cirrhosis and fibrosis in the liver may destroy the normal tissue structure as well as the venovenous collaterals.
There are some limitations to the present study. First, the anatomical structure in animals may be different from that in humans, and the conclusions of this study may not be fully applicable to the human body. Second, the opening of venovenous collaterals in the early stage was pressure excited, while a stable intrahepatic venovenous shunt was confirmed in the LVD pig model, so the development of intrahepatic venovenous shunts in the long term should be further studied.Citation26 Finally, the intrahepatic venovenous shunt is an important outflow channel for the non-isolated liver lobe, and it is conditionally open. Different treatments targeting the liver inflow/outflow pathway can have different effects on the liver, as shown in . What are the effects of these different procedures on the HV pressure and the formation of intrahepatic venovenous shunts? The role of intrahepatic venovenous shunts should be further investigated.
Table 2. Different treatments targeting the liver inflow/outflow pathway can have different effects on the liver.
5. Conclusions
In conclusion, the rat experiment proved that extended HVL(R + MMHVL) can achieve the same results as LVD (HVL + PVL). In addition, the extended HVL procedure eliminated the pressure difference between two adjacent DAs of the HV and prevented the formation of intrahepatic venovenous shunts, which more completely blocked the blood outflow of the congested area, resulting in necrosis of the liver lobe and proliferation of the contralateral liver. There are three characteristics of the intrahepatic venovenous shunts: (1) two-way opening; (2) timely opening; and (3) pressure dependent. Finally, the extended HVL operation is simpler than LVD, with less risk of bleeding and greater clinical significance, but more clinical trials are needed.
Author contributions
Study concept and design (XH, YZ, GZ), acquisition of data (PM, LX, WW), analysis and interpretation of data (YX, GZ), drafting of the manuscript (YS), critical revisions of the manuscript for important intellectual content (XH, KY), and administrative, technical, or material support, study supervision (KY, WW).
Abbreviations | ||
ALB | = | albumin |
ALT | = | alanine aminotransferase |
ALPPS | = | associating liver partition and portal vein ligation for staged hepatectomy |
AST | = | aspartate aminotransferase |
CL | = | caudate lobe |
DA | = | drainage area |
DAL | = | drainage area of left median hepatic vein |
DAM | = | drainage area of middle median hepatic vein |
DAR | = | drainage area of right median hepatic vein |
FLE | = | future liver excised |
FLR | = | future liver remnant |
GS | = | glucose and sodium chloride |
H-E | = | hematoxylin-eosin |
HV | = | hepatic vein |
HVE | = | hepatic vein embolization |
HVL | = | hepatic vein ligation |
LLL | = | left lateral lobe |
LMHV | = | left median hepatic vein |
LML | = | left median lobe |
LMPV | = | right median portal vein |
LVD | = | liver venous deprivation |
MMHV | = | middle median hepatic vein |
MMHVL | = | middle median hepatic vein ligation |
POD | = | postoperative day |
PT | = | Prothrombin time |
PLT | = | platelet |
PV | = | portal vein |
PVE | = | portal vein embolization |
PVL | = | portal vein ligation |
RIL | = | right inferior lobe |
RMHV | = | right median hepatic vein |
RMHVL | = | right median hepatic vein ligation |
RML | = | right medial lobe |
RMPV | = | right median portal vein |
RMPVL | = | right median portal vein ligation |
RSL | = | right superior lobe |
T-Bil | = | total bilirubin |
Supplemental Material
Download PDF (251.2 KB)Data sharing statement
Data and materials are available upon reasonable request to the corresponding author.
Disclosure statement
The authors have no conflicts of interest related to this article to declare.
Additional information
Funding
References
- Rahbari NN, Garden OJ, Padbury R, et al. Posthepatectomy liver failure: a definition and grading by the International Study Group of Liver Surgery (ISGLS). Surgery. 2011;149(5):1–11. doi:10.1016/j.surg.2010.10.001.
- Memeo R, Conticchio M, Deshayes E, et al. Optimization of the future remnant liver: review of the current strategies in Europe. Hepatobiliary Surg Nutr. 2021;10(3):350–363. doi:10.21037/hbsn-20-394.
- Fernandez H, Nadalin S, Testa G. Optimizing future remnant liver prior to major hepatectomies: increasing volume while decreasing morbidity and mortality. Hepatobiliary Surg Nutr. 2020;9(2):215–218. doi:10.21037/hbsn.2019.10.24.
- Le Roy B, Dupré A, Gallon A, Chabrot P, Gagnière J, Buc E. Liver hypertrophy: underlying mechanisms and promoting procedures before major hepatectomy. J Visc Surg. 2018;155(5):393–401. doi:10.1016/j.jviscsurg.2018.03.005.
- Kim D, Cornman-Homonoff J, Madoff DC. Preparing for liver surgery with “Alphabet Soup”: PVE, ALPPS, TAE-PVE, LVD and RL. Hepatobiliary Surg Nutr. 2020;9(2):136–151. doi:10.21037/hbsn.2019.09.10.
- Guiu B, Chevallier P, Denys A, et al. Simultaneous trans-hepatic portal and hepatic vein embolization before major hepatectomy: the liver venous deprivation technique. Eur Radiol. 2016;26(12):4259–4267. doi:10.1007/s00330-016-4291-9.
- Guiu B, Quenet F, Escal L, et al. Extended liver venous deprivation before major hepatectomy induces marked and very rapid increase in future liver remnant function. Eur Radiol. 2017;27(8):3343–3352. doi:10.1007/s00330-017-4744-9.
- Zhang Y, He X, Ma P, et al. Establishment of a rat model of liver venous deprivation: simultaneous portal and hepatic vein ligation. J Clin Transl Hepatol. 2023;11(2):393–404. Epub 2022 Jul 15. PMID: 36643043; PMCID: PMC9817047. doi:10.14218/JCTH.2022.00032.
- Shou-Wang C, Shi-Zhong Y, Wen-Ping L, et al. Sustained methylene blue staining to guide anatomic hepatectomy for hepatocellular carcinoma: initial experience and technical details. Surgery. 2015;158(1):121–127. doi:10.1016/j.surg.2015.01.018.
- Sparrelid E, Hasselgren K, Røsok BI, et al. How should liver hypertrophy be stimulated? A comparison of upfront associating liver partition and portal vein ligation for staged hepatectomy (ALPPS) and portal vein embolization (PVE) with rescue possibility. Hepatobiliary Surg Nutr. 2021;10(1):1–8. doi:10.21037/hbsn.2019.10.36.
- Heil J, Korenblik R, Heid F, et al. Preoperative portal vein or portal and hepatic vein embolization: DRAGON collaborative group analysis. Br J Surg. 2021;108(7):834–842. doi:10.1093/bjs/znaa149.
- Kobayashi K, Yamaguchi T, Denys A, et al. Liver venous deprivation compared to portal vein embolization to induce hypertrophy of the future liver remnant before major hepatectomy: A single center experience. Surgery. 2020;167(6):917–923. doi:10.1016/j.surg.2019.12.006.
- Le Roy B, Gallon A, Cauchy F, et al. Combined biembolization induces higher hypertrophy than portal vein embolization before major liver resection. HPB (Oxford). 2020;22(2):298–305. doi:10.1016/j.hpb.2019.08.005.
- Laurent C, Fernandez B, Marichez A, et al. Radiological Simultaneous Portohepatic Vein Embolization (RASPE) before major hepatectomy: a better way to optimize liver hypertrophy compared to portal vein embolization. Ann Surg. 2020;272(2):199–205. doi:10.1097/SLA.0000000000003905.
- Schadde E. Combined portal vein and hepatic vein embolization—finally the platinum procedure of regenerative liver surgery? Hepatobiliary Surg Nutr. 2020;9(1):92–94. doi:10.21037/hbsn.2019.09.20.
- Eipel C, Abshagen K, Vollmar B. Regulation of hepatic blood flow: the hepatic arterial buffer response revisited. World J Gastroenterol. 2010;16(48):6046–6057. doi:10.3748/wjg.v16.i48.6046.
- Tripathi DM, Erice E, Lafoz E, et al. Metformin reduces hepatic resistance and portal pressure in cirrhotic rats. Am J Physiol Gastrointest Liver Physiol. 2015;309(5):G301–9. doi:10.1152/ajpgi.00010.2015.
- Scatton O, Plasse M, Dondero F, Vilgrain V, Sauvanet A, Belghiti J. Impact of localized congestion related to venous deprivation after hepatectomy. Surgery. 2008;143(4):483–489. doi:10.1016/j.surg.2007.11.002.
- Nitta H, Kitano Y, Miyata T, et al. Validation of functional assessment for liver resection considering venous occlusive area after extended hepatectomy. J Gastrointest Surg. 2020;24(7):1510–1519. doi:10.1007/s11605-019-04234-9.
- Lo CM. The middle hepatic vein controversy: art and science. Liver Transpl. 2018;24(7):870–871. doi:10.1002/lt.25207.
- Beppu M, Fukuzaki T, Mitani K, Fujimoto K, Taniguchi S. Hepatic subsegmentectomy with segmental hepatic vein sacrifice. Arch Surg. 1990;125(9):1170–1175. doi:10.1001/archsurg.1990.01410210096015.
- Huang H, Deng M, Jin H, Liu A, Dirsch O, Dahmen U. Hepatic arterial perfusion is essential for the spontaneous recovery from focal hepatic venous outflow obstruction in rats. Am J Transplant. 2011;11(11):2342–2352. doi:10.1111/j.1600-6143.2011.03682.x.
- Sakaguchi T, Suzuki S, Hiraide T, et al. Detection of intrahepatic veno-venous shunts by three-dimensional venography using multidetector-row computed tomography during angiography. Surg Today. 2014;44(4):662–667. doi:10.1007/s00595-013-0710-6.
- Ma J, Gong X, Luo J, et al. Impact of intrahepatic venovenous shunt on hepatic venous pressure gradient measurement. J Vasc Interv Radiol. 2020;31(12):2081–2088. doi:10.1016/j.jvir.2020.08.027.
- Sakaguchi T, Suzuki S, Inaba K, et al. Analysis of intrahepatic venovenous shunt by hepatic venography. Surgery. 2010;147(6):805–810. doi:10.1016/j.surg.2009.11.009.
- Schadde E, Guiu B, Deal R, et al. Simultaneous hepatic and portal vein ligation induces rapid liver hypertrophy: a study in pigs. Surgery. 2019;165(3):525–533. doi:10.1016/j.surg.2018.09.001.