Abstract
This 90-day study was aimed at characterizing the differences in biological activity between a crystalline ground reference quartz (DQ12) and a quartz with occluded surfaces (quartz isolate) obtained from a clay deposit formed 110 to 112 million years ago. In different test groups, rats were dosed with the same total mass and quartz level by intratracheal instillation, with a total high dose of 15.2 mg/kg (body weight, bw) or approximately 4.7 mg/rat of each quartz species in a saline suspension. The reference quartz was mixed with titanium dioxide to achieve a positive control mixture, which contained the same quartz content as in the quartz isolate. At 3 days post dosing, both quartz groups showed a significant inflammatory response based on total and differential cell counts from bronchoalveolar lavageate (BAL) analysis. At 28 and 90 days, the quartz isolate values were no longer statistically different from vehicle control group values; however, the positive control group values were approximately 12 and 65 times greater than those of the control group, respectively. After 28 days, histopathological evaluation showed moderate effects in the quartz isolate group compared to the saline control animals. These effects did not progress in severity at 90 days. In contrast, the positive control group exhibited more severe effects than the quartz isolate group and these effects showed a progression to a persistent and self-perpetuating inflammatory state. The toxicological properties of quartz particles can vary significantly dependent on their surface characteristics. Toxicity can range from a high-dose-induced, modest, transient inflammation from quartz with occluded surfaces, to a severe and persistent inflammatory state caused by ground quartz with fractured surfaces.
In its 1997 monograph, the International Agency for Research on Cancer (IARC) assessed the carcinogenic potential of respirable crystalline silica using data from human epidemiological and chronic animal inhalation studies. Their general conclusion, that respirable crystalline silica is a human carcinogen, was supplemented by the restrictive statement “that carcinogenicity in humans was not detected in all industrial circumstances studied. Carcinogenicity may be dependent on inherent characteristics of the crystalline silica or external factors affecting its biological activity or distribution of its polymorphs.” Research conducted by Hemenway (Citation1986, Citation1990) has emphasized the crucial role of specific particle properties, such as surface area, protein binding ability, and solubility for initiation of an inflammatory response in lungs. In these rat studies, different lung responses were obtained from α-cristobalite and various types of α-quartz. An important outcome of these studies was that some quartz types show a low and transient toxicological response after uptake in the lungs, as defined by inflammatory cells in the bronchoalveolar lavage (BAL) fluid. Mechanistic studies conducted by CitationDonaldson and Borm (1998) have emphasized that the toxic impact of quartz is related to surface reactivity and may vary dramatically depending on the origin of the quartz sample. These studies also showed that the mechanism of quartz toxicity includes the generation of free radicals and reactive oxygen species. Additionally, CitationFubini (1998) has found that the biological response to quartz can be reduced by substances affecting the quartz surface. These studies point out the need to better understand the relationship between quartz types and toxicological responses.
The current study was undertaken to investigate the intrinsic lung toxicity of a respirable-size quartz, with naturally occluded surfaces (quartz isolate), that had been isolated from a sodium bentonite clay deposit which formed 110 to 112 million years ago. The isolation techniques used to obtain the quartz isolate enriched the quartz content while avoiding the use of chemicals or mechanical grinding that might artificially affect the occluded surfaces of the quartz particles. Lung effects were characterized using BAL and histopathological parameters at 3, 28, and 90 days after intratracheal instillation of test materials into rats. The effects were compared to those of a reference quartz (DQ12) with a well-characterized biological activity.
This study was preceded by a 28-day intratracheal rat instillation study that investigated the inflammatory and genotoxic effects of similar quartz isolate and reference quartz (DQ12 fine size) samples (CitationCreutzenberg et al., 2003). In the 28-day study, the intrinsic toxicity of the quartz isolate sample (geometric mean: 3.70) was compared to that of the reference quartz (geometric mean: 1.31). The inflammatory endpoints, bronchoalveolar lavage (BAL) and histopathology of lungs, revealed a moderate effect induced by the quartz isolate, whereas the reference quartz showed a strong effect, e.g., 36% PMNs in BAL fluid on day 28 after instillation versus 65%, respectively.
Since it is known that particle diameter can play a significant role in the toxicity of crystalline silica, it could not be determined whether the results from the 28-day study were attributable to particle size differences or to other factors. In the current study, a quartz isolate and a reference quartz with similar particle size distributions, geometric means of 3.58 and 3.01 μ m, respectively, were used. A 3-mo postdosing observation period was also used, which gave a broader base for evaluating the effects on the lungs. This study also included lung retention measurements of the test materials, which provided some information on the toxicokinetics and effects on the morphological properties of the quartz species after 90 days in the lungs.
MATERIALS AND METHODS
Test Materials
Quartz Isolate
A quartz isolate sample was extracted from crude, undried and unprocessed bentonite clay obtained from the 100-to 112-million-year-old, lower Cretaceous age, northeastern Wyoming Newcastle formation. As used here, the term “bentonite” is restricted to a geologic deposit derived from the alteration of silica-rich, glassy volcanic ash that fell into a shallow sea, dissolved rapidly, and re-precipitated primarily as the aluminosilicate mineral montmorillonite. Excess silica resulting from this re-precipitation formed quartz and the amorphous silica hydrate mineral opal. Quartz formed in this manner bears the name “authigenic” to differentiate it from quartz resulting from crystallization from the molten state. Authigenic quartz, in bentonite, exhibits a low degree of internal crystal organization as compared to quartz formed at high temperature, which exhibits a much higher degree of internal crystal organization. The surfaces of quartz particles formed in this manner are also intimately associated with, and occluded by, coatings of the clay and amorphous silica with which they were formed, as well as water and metal ions such as Al3 + and Fe2 +/3 +.
The quartz isolate was gently extracted from an aqueous suspension of crude, unground bentonite clay, without the addition of any chemicals, using a centrifugal method designed to avoid alteration of the quartz particle surfaces. The physicochemical properties of the quartz isolate obtained in this manner are presented in . The quartz isolate particles consist of a sedimentary, chert-like, microcrystalline form of quartz formed at low temperature in the presence of liquid water, intergrown with the amorphous hydrous mineral opal and the clay mineral montmorillonite. shows a scanning electron photomicrograph of a representative sample of quartz isolate. The convoluted nature of the quartz particles and their occluding coating can clearly be seen in this figure. The quartz content of the quartz isolate was 60%. Prior to use, the quartz isolate was stored at 3°C as a 34.8% suspension in deionized water.
TABLE 1 Physicochemical properties of test materials used in 90-day study
Reference Quartz
The quartz sample DQ12 middle size was obtained from Bergbauforschung, Essen, Germany. This material, which was produced by mechanical grinding, was a mid-size fraction of a classified Dörentrup quartz sample, and was used as the reference quartz. It is thought to consist entirely of broken fragments of particles of single crystal α-quartz. α-Quartz is a polymorph of quartz crystallized from an igneous melt. The physicochemical properties of the reference quartz are presented in . shows a scanning electron photomicrograph of a representative sample of the reference quartz material. The clean, flat surfaces and highly angular nature of the particles of this material can clearly be seen here.
Titanium Dioxide
The titanium dioxide sample Bayertitan T (TiO2) was obtained from Bayer AG, Krefeld, Germany. Based upon information obtained from the supplier, the chemical composition is 98.174% TiO2, the annealing weight loss is 0.22%, and the mineralogical composition is 99.5% rutile polymorph. The density of this material is 4.26 g/cm3. Additional physicochemical properties of the titanium dioxide are also presented in . shows a scanning electron photomicrograph of a representative sample of the titanium dioxide.
Animals and General Procedures
Male Wistar rats (strain Crl:WU), approximately 6 wk of age at delivery, were obtained from Charles River Deutschland, Sulzfeld, Germany. The age of the animals at the start of treatment was approximately 11 wk and their weight was approximately 300 g. Rats were dosed with the test materials by intratracheal instillation. Males were preferred to females because literature data indicate a higher sensitivity in the fibrotic response induced by crystalline silica (CitationHonnons & Porcher, 2000).
Study Design and Dosing Scheme
The test materials were administered on a body weight basis (). The quartz isolate and positive control were dosed at 15.2 mg/kg body weight. Aliquots of 3.8 mg/kg body weight, suspended in approximately 0.3 ml saline, were administered intratracheally in 4 consecutive daily applications. To minimize particle agglomeration, the suspensions were shaken moderately prior to instillation but did not undergo an ultrasonic treatment. The study was designed to dose the animals, in the different test groups, with the same total mass and the same quartz level. The quartz content of the quartz isolate was 60% while the quartz content of the reference quartz was 87%. Thus, in order to deliver the same mass dose as well as the same dose of quartz, the reference quartz had to be supplemented with additional particulate material to produce a positive control mixture that contained 60% quartz. Fine titanium dioxide particles were selected because they represent particles that have been used and recognized for decades, in inhalation toxicology, as a negative control particle (CitationFerin et al., 1965; CitationFerin, 1994). In the previously conducted 28-day study (CitationCreutzenberg et al., 2003), separate groups of rats were dosed with reference quartz (quartz DQ12 fine size) or a mixture of 71.25% reference quartz and 28.75% titanium dioxide. In both cases the total amount of reference quartz was identical. The results of the 28-day study clearly showed that, at all time periods, the effects caused by reference quartz alone were essentially identical to those seen in rats dosed with the mixture of reference quartz and titanium dioxide. Based on these results, it was concluded that the presence of titanium dioxide would not alter the toxicological effects of the reference quartz in the present study. Therefore, in the present study, the effects seen in the animals dosed with the positive control mixture are assumed to be attributable to the reference quartz.
TABLE 2 Study design
The quartz isolate was supplied as a 38.8% aqueous suspension in deionized water. Sodium chloride was added to reach a 0.9% isotonic saline concentration. The suspension was further diluted with sterile and pyrogen-free saline to reach the target concentration for dosing. Only saline was added to the positive control mixture to prepare the positive control dosing suspension. Concurrent control animals were treated with the saline vehicle only. Prior to dosing, the rats were slightly anesthetized with CO2.
Sacrifice of Rats
Rats scheduled for bronchoalveolar lavage (BAL) were anesthetized by an intraperitoneal application of sodium pentobarbital (Narcoren at 0.1 ml per 100 g body weight) and exsanguinated by cutting the vena cava caudalis. Rats scheduled for histopathological examination were sacrificed by an overdose of carbon dioxide and subsequent bleeding via cutting the vena cava caudalis.
Endpoints
Bronchoalveolar Lavage (BAL)
On days 3, 28, and 90 posttreatment, a bronchoalveolar lavage (BAL) was performed and the lavage fluid collected for differential cell counts and analysis of biochemical parameters (see and ). With minor alterations, the procedure followed the method described by CitationHenderson et al. (1987). Following preparation, the lungs were lavaged with saline using 5 lavages of 5 ml each. The lavage fluid was collected separately for lavages 1 and 2 (first series, without massage of lungs) and for lavages 3 to 5 (second series, using gentle massage of lungs). After centrifugation of the first series, biochemical indicators relevant for diagnosis of lung damage were determined in the supernatant (lactic dehydrogenase [LDH], β-glucuronidase, total protein). The cell pellet of the first lavage series was resuspended in the residual supernatant, combined with the second lavage series, and the resulting cell pool of five lavages was used for cytology. The leukocyte concentration of the lavageate was determined using a counting chamber and two cytoslides were prepared with a cytocentrifuge (Shandon Co., Frankfurt, Germany) for differential cell count (macrophages, neutrophils, eosinophils, lymphocytes, epithelial cells).
Histopathology
On days 28 and 90 posttreatment, histopathology of all 5 lung lobes, including bronchi and the lung-associated lymph nodes (LALN), was conducted on 5 animals per group. Lungs were inflated under a pressure of approximately 20 cm water, with 10% neutral-buffered formalin, and fixed by immersion. They were embedded in paraffin, sectioned, and stained with hematoxylin and eosin (H&E). A special stain (Masson's trichrome) was applied for diagnosis of fibrotic changes.
Morphology of Test Materials in Lungs
Lungs were harvested from dosed and undosed (reserve) rats and lyophilized 90 days after treatment of the animals. The lungs were then ashed using a low-temperature plasma technique and the inorganic content of the ash was analyzed by scanning electron microscopy to determine general particle morphology, particle size distribution, and particle chemistry.
Statistics
Differences between groups were considered to be statistically significant at the level of p < .05. Data were analyzed using analysis of variance. If the group means differed significantly by the analysis of variance the means of the treated groups were compared with the means of the control groups. Tukey's test was used. Incidences of histopathological microscopic findings were compared using the two-tailed Fisher test at a level of p < .05.
RESULTS
Survival of Animals and Clinical Observations
No deaths occurred during the course of the study. Body weights were in the normal range and treatment-related effects were not observed.
BAL Enzyme and Total Protein Levels
The results of BAL fluid analysis are summarized in and . Plots of lactic dehydrogenase, β-glucuronidase and total protein are presented in through . The saline control group values were at historical levels.
TABLE 3 Means of parameters measured in BAL fluid 3, 28, and 90 days after the last of 4 instillations
TABLE 4 Differential cell count 3, 28, and 90 days after intratracheal instillation (means)
FIG. 5 Quartz isolate recovered from rat lung after 90 days by low-temperature plasma ashing (5000×).
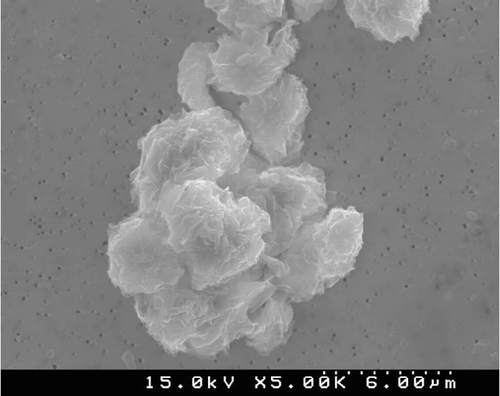
FIG. 6 Lactic dehydrogenase levels in bronchoalveolar lavage fluid measured on days 3, 28, and day 90 after end of treatment.
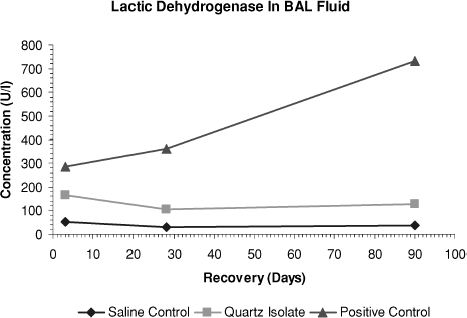
FIG. 7 β-Glucuronidase levels measured in bronchoalveolar lavage fluid on days 3, 28, and day 90 after end of treatment.
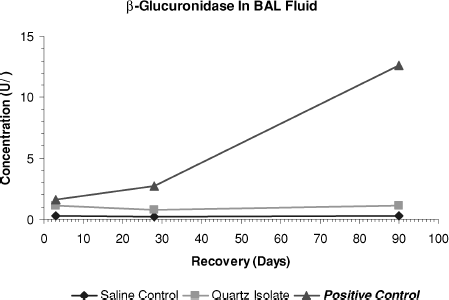
FIG. 8 Total protein levels in bronchoalveolar lavage fluid measured on days 3, 28, and day 90 after end of treatment.
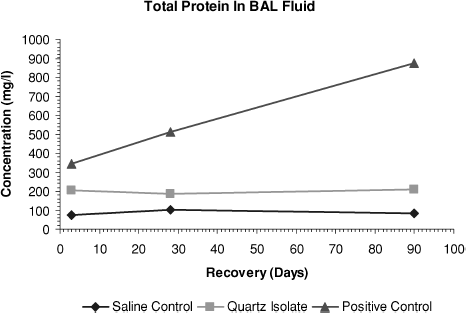
At day 3, the quartz isolate group's β-glucuronidase and total protein values were statistically greater than those of the saline control group. However, during the 90-day posttreatment observation period there was no progression of these effects. In contrast, at all time points in the positive control group, values showed strong, statistically significant differences from the saline control group and there was a significant progression in severity during the study period.
Total and Differential Cell Counts
The results of differential cell counts are presented in . Differential cell counts for the saline control group resulted in polymorphonuclear neutrophil (PMN) levels of 0.0% to 0.3% at 3, 28, and 90 days after the last intratracheal instillation. These values are comparable to historical values.
After 3 days, the PMN value for the quartz isolate group reached 18%, while the value for the positive control group was 39%. The PMN values for the quartz isolate and positive control groups after 28 days were 25% and 45%, respectively. After 90 days they were 32% and 46%, respectively. These data indicate a moderate inflammatory response for the quartz isolate group and a strong response for the positive control group. When considering the large increase in the total cell number by quartz (, ), the number of PMNs for the positive quartz control and quartz isolate (in parenthesis) show even greater differences: 7.78 × 106 (2.25 × 106) on day 3; 18.29 × 106 (2.71 × 106) on day 28; and 99.94 × 106 (2.15 × 106) on day 90. This means, that the elicited PMNs in the quartz exposed rats were manyfold higher than those of quartz isolate exposed rats: 3.5 fold on day 3; 6.8 fold on day 28; and 46 fold on day 90. These data indicate the strongest and persistent inflammatory response in the quartz positive control group, and this response was evidently considerably smaller in the quartz isolate group.
Terminal Body and Lung Wet Weights (BAL Rats)
Absolute and relative wet lung weights of BAL animals were statistically significantly elevated, in both treatment groups, after 3 and 28 days (; ). After 90 days, lung weights in the quartz isolate group were not statistically different from those in the saline control group. By contrast, the positive control group continuously increased lung weights throughout the postexposure period and showed a high degree of statistical difference when compared to the quartz isolate and saline control groups.
TABLE 5 Terminal body and lung wet weights 3, 28, and 90 days after the last of 4 instillations—BAL rats
Histopathology
Histopathologically, the quartz isolate group showed a very slight to slight inflammatory response compared to the saline control group (). At 28 days and 90 days, there was no progression of the effects. The positive control group, after 28 days and 90 days, exhibited a significantly stronger reaction than the quartz isolate group and the effects showed a continued progression in the intensity.
TABLE 6 Histopathological findings on days 28 and 90 posttreatment
Lungs
Very slight interstitial fibrosis of the lungs was observed in the quartz isolate group in all 5 rats after 28 days. At 90 days, the incidence was 4 of 5 rats (). In contrast, all animals of the positive control group, at 28 and 90 days, showed a higher grade of interstitial fibrosis. Alveolar lipoproteinosis, which is a measure of alveolar macrophage degeneration or necrosis, was statistically significantly increased, after 28 and 90 days, in the positive control group. Alveolar lipoproteinosis was not observed in the quartz isolate or saline control groups ().
Lymph Nodes
Accumulation of particle-laden macrophages was most pronounced in the positive control group(moderate: 4/5; severe: 1/5). Particle-laden macrophages were present only to a minimal degree in rats in the quartz isolate group (very slight: 5/5). Associated with this finding was reactive lymphoid hyperplasia, which occurred at slight to moderate degrees in 2/5 (slight: 1/5; moderate: 1/5) animals in the quartz isolate group and 5/5 (slight: 1/5; moderate: 4/5) animals in the positive control group.
DISCUSSION AND CONCLUSION
The focus of this study was to characterize differences in biological activity between different quartz species of similar particle size. A vast literature exists suggesting that (1) geologically old mixtures, (2) aging of freshly cleaved quartz (not for all varieties observed), and (3) experimental surface modification have an attenuating effect on the intrinsic toxicity of quartz-containing particles. For example, contaminants such as coal dust or metals (aluminum, iron) can diminish the toxic potential of quartz in quartz-containing materials (CitationFubini, 1998), and crushed quartz and aged quartz behave differently in terms of toxicological potency (CitationVallyathan et al., 1995; CitationCastranova et al., 1997). The fracturing of quartz generates silicon-based free radicals that are able to produce reactive oxygen species (ROS) (CitationDonaldson & Borm, 1998). In terms of toxicological potency, freshly ground quartz-containing materials are significantly more capable of causing lung injury than corresponding aged materials. The surfaces of freshly ground quartz particles become less active as they age due to the reaction of free radicals on the fractured surfaces with other ions or molecules. Although an aging effect may not be observed for every quartz modification, it is, nevertheless, an important parameter that must be considered in evaluating the toxic potential of quartz-containing materials from different sources.
CitationRoss et al. (1962) exposed rats to a mixture of quartz dust and very low quartz coal dust (ground together at a ratio of 5% and 95%). Although the quartz/coal dust mixture was inhaled 16 h/day at 60 mg/m3 for 1 yr, the high level of inhaled quartz induced only little fibrosis, demonstrating the reduction of toxicity due to the accompanying coal dust. In a complementary rat experiment, CitationLe Bouffant et al. (1982) compared a quartz-containing coal dust to an artificial quartz/coal dust mixture (not ground together) of the same quartz content. Following a 12-mo inhalation, lung collagen analysis revealed that the artificial mixture had induced 3 times more collagen as compared to the quartz occurring naturally in association with the coal.
Other examples include studies showing that the strong hydrogen bonding agent polyvinylpyridine N-oxide (PVPNO), after surface treatment, can powerfully attenuate the hemolytic activity of quartz (CitationNolan et al., 1981). Another study of CitationErnst et al. (2002), investigating the quartz surface-modifying effect of PVPNO, confirmed strong reduction of the inflammogenic effect of quartz DQ12 in terms of BAL endpoints or histopathological effects.
Still other studies have demonstrated that aluminum ions can lower quartz-induced toxicity. CitationBrown et al. (1990) found that aluminum-coated quartz was substantially less inflammogenic to the rat lung than native quartz as evaluated by a differential cell count. Thus, as this quenching effect of aluminum lactate is quartz specific it can be used to modify the surface of quartz-containing particles and to distinguish between a quartz-specific induction of inflammation and a general particle effect.
More recently, CitationFubini et al. (2004) characterized four different quartz samples and could attribute physicochemical properties to the varying lung toxicity potential observed by CitationSeiler et al. (2004) in parallel rat studies. Two samples induced inflammation in vivo, whereas the other two were mostly inert. With respect to physicochemical parameters, the two less inflammogenic quartzes were more resistant to solubility in HF, irreversibly coordinated H2O molecules, and exhibited strong adsorption sites. In addition, they appeared to be covered by aluminum ions known to quench quartz-specific toxicity.
The quartz isolate investigated in this study was derived from a sodium bentonite deposit formed 110 to 112 million years ago. It is composed of quartz particles that were formed by precipitation from aqueous solution, at the time the clay was formed, and has been in contact with the clay since that time. In contrast, the reference quartz in the positive control is relatively recently crushed quartz that has been accepted as a biologically active standard in other toxicological studies (CitationRobock, 1973; CitationClouter et al., 2001). The physicochemical properties of the quartz isolate and the reference quartz sample reveal that these materials are substantially different (). In particular, the crystal domain size of the reference quartz is 2.1 times that of the quartz isolate, indicating that the reference quartz has a much coarser crystal structure than does the quartz isolate; the zeta potential of the reference quartz is 20 to 75% higher than that of the quartz isolate, depending on pH, indicating that the reference quartz has a much more electrically reactive surface than does the quartz isolate; and the mineralogical composition, as determined by XRD analysis, and the chemical analysis show the reference quartz to be nearly pure silicon dioxide while the quartz isolate contains 30% montmorillonite clay as an inseparable coating on the surface of the quartz particles. Additionally, the morphological characteristics of particles of these materials are also quite different, as illustrated in and . These, and other differences, provide some physicochemical basis for the differences observed in the overall toxicological results. Based on the greater specific surface area of the quartz isolate compared to the reference quartz (), one would expect that the toxicity of quartz isolate should be significantly greater than for quartz if surface reactivity were the same for both. The opposite was found, i.e. quartz isolate was significantly less toxic than quartz, indicating that surface reactivity of quartz isolate is considerably less reactive than that of the reference quartz.
Samples of the quartz isolate and positive control from low-temperature plasma-ashed lungs of treated animals were evaluated at the end of the study. No significant difference in particle size distribution, morphology, or chemistry between the recovered and original materials was found. and show the appearance of the quartz isolate before and after 90-day retention in rat lungs. The only significant change noted was a decrease in the reference quartz/TiO2 ratio in the recovered positive control, possibly indicating an overproportional clearance of the reference quartz. This effect can be explained by the high cytotoxicity of the reference quartz allowing the permeation of free reference quartz particles through the alveoli walls into the interstitium/lymph nodes. This translocation of quartz particles retained in lungs to lung-associated lymph nodes has been described by CitationPorter et al. (2004). The result of the histopathological examination of the lung associated lymph nodes, presented in , provides evidence that there was significant movement of the reference quartz particles, from the lungs, into the lymph nodes. In addition, there was a marked difference in the degree of accumulation of particle-laden macrophages, between the quartz isolate and the positive control groups. The effect was significantly more progressive in the positive control group, from the 28-day to the 90-day sacrifice. This indicates a greater degree of tissue damage in the positive control group relative to the quartz isolate group. A similar pattern of particle accumulation was also seen in the previously conducted 28-day study (CitationCreutzenberg et al., 2003).
CitationGeh et al. (2006) found that respirable bentonite particles, with up to 6% quartz, did not induce micronuclei (MN) in human lung fibroblasts, whereas the positive control material (quartz DQ12) did. In the 28-day study preceding this 90-day study, micronuclei (MN) in alveolar macrophages were evaluated as an endpoint to determine genotoxic potential. This work revealed a significant increase in MN in the alveolar macrophages from animals treated with the 28-day study reference quartz only. In contrast, no abnormal MN increase was noted in alveolar macrophages from animals treated with the quartz isolate from the 28-day study, the quartz isolate from the 90-day study or the positive control from the 90-day study.
The results of this study consistently reveal that the lung inflammatory response for the quartz isolate was significantly lower than that of the positive control. The biochemical, differential cell count, and histopathology data, summarized in , show a persistent and progressive inflammatory response in the positive control animals. In contrast, the data show a moderate response for the quartz isolate group with an initial inflammatory effect that, for all but the PMN values, remained constant or declined after the first few days.
In conclusion, this study showed that:
The vehicle control did not induce lung inflammation.
The positive quartz control produced significant and progressive lung inflammation.
The quartz isolate induced a significantly weaker inflammatory response than the positive control, and this response was not progressive.
TABLE 7 Summary of the toxicological effects of the quartz isolate and positive control
This study was supported by the Sorptive Minerals Institute, Washington, DC.
REFERENCES
- C. A. Bower, and J. O. Goertzen. (1959). Surface area of soils and clays by an equilibrium ethylene glycol method. Soil Sci. 87:289–292.
- G. M. Brown, K. Donaldson, and D. Brown. (1990). Bronchoalveolar leukocyte response in experimental silicosis: Modulation by a soluble aluminium compound. Toxicol. Appl. Pharmacol. 101:95–105.
- S. Brunauer, P. H. Emmett, and E. Teller. (1938). Adsorption of gases in multimolecular layers. J. Am. Chem. Soc. 60:309–319.
- D. L. Carter, M. D. Heilman, and C. L. Gonzalez. (1965). Ethylene glycol monoethyl ether for determining surface area of silicate minerals. Soil Sci. 100:356–360.
- V. Castranova, V. Vallyathan, D. M. Ramsey, J. L. McLaurin, D. Pack, S. Leonhard, M. W. Barger, J. Y. C. Ma, N. S. Dalal, and A. Teass. (1997). Augmentation of pulmonary reactions to quartz inhalation by trace amounts of iron-containing particles. Environ. Health Perspect. 105 (Suppl. 5):1319–1324.
- A. Clouter, D. Brown, D. Höhr, P. Borm, and K. Donaldson. (2001). Inflammatory effects of respirable quartz collected in workplaces versus standard DQ12 quartz: Particle surface correlates. Toxicol. Sci. 63:90–98.
- O. Creutzenberg, G. Oberdörster, S. G. Ampian, W. F. Moll, R. Hamilton, and H. Muhle. (2003). Inflammatory effects of quartz samples after intratracheal instillation in rats. Toxicologist 72:214. (abstr.).
- K. Donaldson, and P. J. A. Borm. (1998). The quartz hazard: A variable entity. Am. Occup. Hyg. 42:287–294.
- K. E. Driscoll, J. Carter, B. W. Howard, D. G. Hassenbein, W. Pepelko, R. B. Baggs, and G. Oberdörster. (1997). Pulmonary inflammatory, chemokine, and mutagenic responses in rats after subchronic inhalation of carbon black. Carcinogenesis 18:423–430.
- A. C. P. Elder, R. Gelein, J. N. Finkelstein, C. Cox, and G. Oberdörster. (2000). Endotoxin priming affects the lung response to ultrafine particles and ozone in young and old rats. Inhal. Toxicol. 12 (Suppl 1):85–98.
- H. Ernst, S. Rittinghausen, W. Bartsch, O. Creutzenberg, C. Dasenbrock, B.-D. Görlitz, M. Hecht, U. Kairies, H. Muhle, M. Müller, U. Heinrich, and F. Pott. (2002). Pulmonary inflammation in rats after intratracheal instillation of quartz, amorphous SiO2, carbon black, and coal dust and the influence of poly-2-vinylpyridine-N-oxide (PVNO). Exp. Toxicol. Pathol. 54:109–126.
- J. Ferin, G. Urbankiva, and A. Vickova. (1965). Pulmonary clearance and the function of macrophages. Arch. Environ. Health 10:790–795.
- J. Ferin. (1994). Pulmonary retention and clearance of particles. Toxicol. Lett. 72:121–125.
- B. Fubini. (1998). Surface chemistry and quartz hazard. Ann. Occup. Hyg. 42:521–530.
- B. Fubini, I. Fenoglio, R. Ceschino, M. Ghiazza, G. Martra, M. Tomatis, P. Borm, R. Schins, and J. Bruch. (2004). Relationship between the state of the surface of four commercial quartz flours and their biological activity in vitro and in vivo. Int. J. Environ. Health 207:89–104.
- S. Geh, T. Shi, B. Shokouhi, R. P. F. Schins, L. Armbruster, A. W. Rettenmeier, and E. Dopp. (2006). Genotoxic potential of respirable bentonite particles with different quartz contents and chemical modifications in human lung fibroblasts. Inhal. Toxicol 18:405–412.
- D. R. Hemenway, M. P. Absher, L. Trombley, and P. M. Vacek. (1990). Comparative clearance of quartz and cristobalite from the lung. Am. Ind. Hyg. Assoc. J. 51:363–369.
- D. R. Hemenway, M. P. Absher, M. Landesman, L. Tromble, and R. J. Emerson. Differential lung response following silicon dioxide polymorph aerosol exposureSilica, silicosis, and cancerD. F. Goldsmith, D. M. Winn, and C. M. Shy. Praeger, New York, (1986) 105–116.
- R. F. Henderson, J. L. Mauderly, J. A. Pickerell, R. F. Hahn, H. Muhle, and A. H. Rebar. (1987). Comparative study of bronchoalveolar lavage fluid: Effect of species, age and method of lavage. Exp. Lung Res. 13:329–342.
- S. Honnons, and J. M. Porcher. (2000). In vivo experimental model for silicosis. J. Environ. Pathol. Toxicol. Oncol. 19:391–400.
- IARC. (1997). Silica. Silica, some silicates, coal dust and para-aramid fibrils. IARC Monogr. Eval. Carcinogen. Risks Hum 68:210–211.
- L. Le Bouffant, H. Daniel, J. C. Martin, and S. Bruyere. (1982). Effect of impurities and associated minerals on quartz toxicity. Ann. Occup. Hyg. 26:625–634.
- W. Miles, W. F. Moll, R. D. Hamilton, and R. K. Brown. (2008). Physicochemical and mineralogical characterization of test materials used in 28-day and 90-day intratracheal instillation toxicology studies in rats. Inhal. Toxicol. 20:981–993.
- R. P. Nolan, A. M. Langer, J. S. Harington, G. Oster, and U. Selikoff. (1981). Quartz hemolysis as related to its surface functionalities. Environ. Res. 26:503–520.
- G. Oberdörster, J. Ferin, and B. E. Lehnert. (1994). Correlation between particle size, in vivo particle persistence, and lung injury. Environm. Health Perspect. 102 (Suppl. 5):173–179.
- D. W. Porter, A. F. Hubbs, R. Mercer, V. A. Robinson, D. Ramsey, J. McLaurin, A. Khan, L. Batelli, K. Brumbaugh, A. Teass, and V. Castranova. (2004). progression of lung inflammation and damage in rats after cessation of silica inhalation. Toxicol. Sci. 79:370–380.
- K. Robock. (1973). Standard quartz DQ12 < 5 μ m for experimental pneumoconiosis research projects in the Federal Republic of Germany. Ann. Occup. Hyg. 16:63–66.
- H. F. Ross, E. J. King, M. Yognathan, and G. Nagelschmidt. (1962). Inhalation experiments with coal dust containing 5%, 10%, 20% and 40% quartz: Tissue reactions in the lungs of rats. Ann. Occup. Hyg. 5:149–161.
- F. Seiler, B. Rehn, S. Rehn, and J. Bruch. (2004). Different toxic, fibrogenic and mutagenic effects of four commercial quartz flours in the rat lung. Int. J. Environ. Health 207:115–124.
- U.S. Environmental Protection Agency, and Recommendations and documentation of biological risk. U.S. EPA, WashingtonDC, (1988) PB88/127984, February..
- V. Vallyathan, V. Castranova, D. Pack, S. Leonhard, L. Shumaker, A. F. Hubbs, D. A. Shoemaker, D. M. Ramsey, J. R. Pretty, and J. L. McLaurin. (1995). Freshly fractured quartz inhalation leads to enhanced lung injury and inflammation. Potential role of free radicals. Am. J. Respir. Crit. Care Med. 152:1003–1009.
- V. Vu, J. C. Barrett, J. Roycroft, L. Schuman, and D. Dankovic. (1996). Workshop report: Chronic inhalation toxicity and carcinogenicity testing of respirable fibrous particles. Regul. Toxicol. Pharmacol. 24:202–212.
- B. E. Warren, and B. L. Averbach. (1953). The effect of cold-work on X-ray patterns. J. Appl. Phys. 21:595–599.