Abstract
Liposomal amphotericin B (AmBisome®) is a lipid-based nanotherapeutic that is used successfully worldwide to treat a broad range of life-threatening invasive fungal infections. In subtropical regions, AmBisome is emerging as the treatment of choice for human parasitic protozoan pathogens such as those from the genus Leishmania. The key to the remarkable efficacy of AmBisome is attributed to its liposome based formulation to deliver a potent drug at high dosage with significantly reduced toxicity in patients with immunocompromised systems. In spite of the rising frequency of AmBisome usage globally, the mechanisms underlying its ability to target to the sites of infection remain largely unknown. This review provides an overview of the current mechanistic understanding of AmBisome, discusses potential challenges and opportunities for the development of clinically effective, refractory resistant antifungal agents.
Introduction
Debilitating to life-threatening systemic fungal infections have been a scourge for immunocompromised patients in the intensive care unit wards for years, and have only been worsening recently as an increasing number of patients who are prescribed immunosuppressive drugs become susceptible to these opportunistic invasive fungal infections. Unfortunately, the mortality rate associated with invasive fungal diseases remains remarkably high in spite of the increased usage of and diversity of antifungal agents. Out of the five classes of antifungals that make up the normal treatment regimen, amphotericin B, a polyene antifungal, is uniquely equipped to kill a broad spectrum of invasive fungal species (and even including parasitic species, e.g. visceral leishmaniasis), while retaining a remarkably low incidence of reported microbial resistance despite decades of worldwide usage. Amphotericin B based therapies are an instrumental part of the arsenal to treat devastating mold infections of the central nervous system including the highly publicized 2012 outbreak of fungal meningitis caused by contaminated methylprednisone (McCarthy et al. Citation2014). Despite its effectiveness against common systemic fungal infections such as invasive aspergillosis, Candida infections, mucormycosis, and cryptococcal meningitis, a conventional formulation of amphotericin B deoxycholate micelle suspension is severely limited by its therapeutic dose-dependent nephrotoxicity and infusion-related reactions.
To improve upon these dosage dependent toxicities, a liposomal formulation was developed in the 1980s and successfully demonstrated in preclinical models to achieve a reduction in toxicities by at least 30-fold while retaining the pharmacological effectivity of the active ingredient (Adler-Moore and Proffitt Citation1993). This liposomal based formulation marketed under the tradename AmBisome involves embedding the amphotericin B active ingredient into the bilayer of small (∼80 nm median size) unilamellar liposomes. The first commercially available AmBisome was approved in the 1990s and is now available globally in over 58 countries for use as a safe and effective treatment against severe systemic fungal infections. The liposomal formulation is as effective as the conventional formulation and, more importantly, tolerated at significantly higher dosages. This year marks the 20th anniversary of AmBisome since it was first approved by the FDA in 1997. Amphotericin B and its liposomal formulation, AmBisome, have demonstrated very high cure rates (up to 96%) in Bihar, India in patients infected with visceral leishmaniasis, which is caused by a protozoa agent transmitted by parasites in infected sand flies (Moore and Lockwood Citation2010). Relative to fungal treatment, in spite of its high clinical efficacy, relatively little is known at the molecular level of the mechanism by which AmBisome traverses through the fungal cell wall to reach the fungal membrane and the precise way by which amphotericin B is able to leave the bilayer to exert its toxic effects. Moreover, even less is understood of the mechanisms underlying the profile of resistance to amphotericin B despite more than 40 years of clinical use. This review provides an update on what is currently known regarding targeting of AmBisome to the fungal membrane, with brief comments on potential clinical relevance. The aim is to summarize key aspects underpinning mechanism of action for AmBisome that in turn support efforts to improve use of AmBisome or in the future design of antifungal agents with high antibiotic refractory-resistance activity.
The overall pharmacokinetic profile of AmBisome is increasingly well understood based on years of extensive characterization in preclinical model systems (Adler-Moore et al. Citation2017). A number of murine studies have demonstrated that AmBisome is partly concentrated in the spleen and liver as well as kidneys that makes up the majority of the reticuloendothelial system (RES) as well as the kidneys and lung (Oku et al. Citation2000). Both the particle size and liposomal composition are critical factors that influence a distinct set of pharmacokinetic properties of AmBisome. For example, by featuring a small particle size, liposomes are able to evade the uptake and opsonization by macrophages residing within the RES. For AmBisome, this RES avoidance profile is somewhat altered by the overall anionic charge on the particle. This escape is the key reason liposomes can have longer residence mean times in tissues which, presumably, result in a potentially larger therapeutic effect. Studies in rabbits, for instance, have found that AmBisome can penetrate the blood brain barrier and concentrate in the brain that is due in part to the less rapid clearance of the liposomes from the bloodstream (Groll et al. Citation2000). Likewise, much of the pharmacokinetic data in patients have shown similar localization patterns of AmBisome concentrations in the liver, spleen, kidneys, lungs and brain that are consistent with that found in preclinical data (Stone et al. Citation2016).
Despite having good basic knowledge on the distribution pattern of AmBisome in vivo, there is a lack of understanding pertaining to the distribution of AmBisome within the sub-compartments of a specific tissue due to technical limitations inherent to measuring concentrations from whole tissue homogenates. More importantly, there is some evidence of treatment limitation at a given dose from the use of current amphotericin B formulations to reach the site of fungal infections located in deep tissue regions where amphotericin B concentrations are either undetectable or below the minimum inhibitory concentration (MIC) (Paterson et al. Citation2003). Relatively little is known regarding the mechanisms underlying the targeting of AmBisome to the fungal cell wall, how it transits to the fungal membrane from the cell wall or how amphotericin B is released from the liposome to exert its fungicidal effect. Therefore, acquiring a better understanding of the mechanisms underlying liposome delivery to the site of infection will further support efforts to improve upon the design of liposome carriers that can improve the therapeutic index by improving the delivery of antifungal agents with strong antimicrobial refractory-resistance at infection sites.
Mechanism of action
The effectiveness of amphotericin B as a fungicidal agent is widely thought to be attributed to its ability to bind to ergosterol and a singular ability to self-assemble into ion-permeating channels in the fungal cell membrane. A central driver of the pharmacokinetic properties specific to AmBisome is the makeup of its liposome composition. Its liposome bilayer is composed of a mixture of disteareoyl phoshatidylglycerol (DSPG), hydrogenated soy phoshatidylcholine (HSPC), and cholesterol (Adler-Moore and Proffitt Citation1993). Amphotericin B has an amphoteric architecture comprised of a rigid, hydrophobic polyene macrolide backbone attached to the carboxylate and mycosamine appendages. The addition of cholesterol to the liposomal formulation facilitates retention of amphotericin B within the lipid bilayer by stabilizing it via hydrophobic interaction between the sterol core and the polyene backbone of amphotericin B, thus improving the drug’s toxicity profile.
For decades since its discovery, amphotericin B has been known to exhibit a relatively high affinity to bind to ergosterol, a key factor that drives amphotericin B towards the fungal membrane. According to the prevailing theory, the mechanism underpinning amphotericin B broad-spectrum antifungal activity is caused by the drug’s ability to self-assemble in the lipid bilayer to form discrete ion-permeable channels in the fungal membrane leading to the leakage of cytoplasmic contents and thereby causing cell death. This classic ‘barrel-stave’ channel model has been the dominate hypothesis over the last five decades since its discovery and suggests that the path to improved therapeutic index involves the formation of fungal-selective ion channels. Despite the extensive evidence characterizing the channel activity by amphotericin-embedded liposomes in vitro, it is not entirely clear that ion channel activity and permeabilization is necessarily the essential mechanism driving fungicidal action (Khutorsky Citation1992, Murata et al. Citation2009). In recent years an alternative model has emerged emphasizing that the strong binding affinity between amphotericin B and the sterol ergosterol is itself sufficient as the primary driver of fungicidal activity (Gray et al. Citation2012). Based on mutation studies in yeast, selectively deleting functional groups of amphotericin B identified a mutant that abolishes channel forming activity while retaining the capacity to bind to ergosterol. Sterol binding, not channel formation, may indeed play a role in fungicidal activity (Palacios et al. Citation2007, Palacios et al. Citation2011). Collectively, these findings advance the idea that channel formation and cytotoxic activities may be segregated and are not necessarily inextricably linked as suggested by the classic model.
Given that ergosterol serves a multitude of critical functions such as vacuole fusion, membrane regulation, cell division and cell growth, it is not so farfetched to speculate that perturbing the function of a vital fungal sterol could lead to pleiotropic physiological effects with devastating consequences – i.e. cell death (Alcazar-Fuoli and Mellado Citation2013). In stark contrast to the classic channel model, investigators provided evidence from solid-state NMR and transmission electron microscopy that amphotericin B can form extra-membrananous aggregates that sequester ergosterol by extracting it from the fungal membrane (Anderson et al. Citation2014). This new sterol sponge model proposes that ergosterol extraction is sufficient to destabilize homeostasis and disturb the fungal membrane, leading to cell death. Alternatively, earlier studies have reported that amphotericin B induces oxidative stress can damage fungal cells and result in cell death (Mesa-Arango et al. Citation2012). Taken together, such evidence broadens our understanding that channel formation may not be the only means by which to kill fungi, and enhances the onus to develop an improved understanding of mechanism of action.
Targeting to cell wall
Undoubtedly, the efficacy of the drug is inextricably related to its ability to reach the site of fungal infection. In in vivo pre-clinical model systems, researchers have discovered that the drug accumulates at sites at or near fungal infections. The distribution of AmBisome in tissues has been studied utilizing liposomes, with or without amphotericin B, that carry aqueous fluorescent dyes entrapped in the insides of liposomes. In mouse model systems infected with either Aspergillus fumigatus or Candida albicans, sulphorhodamine-labelled AmBisome readily localizes to the lungs and kidney sites of fungal outbreak (Adler-Moore and Proffitt Citation1993, Takemoto et al. Citation2006). The ability to localize to these infection sites is independent of the presence of amphotericin B since sulphorhodamine containing liposomes without drug also accumulate at these same tissue sites of infection. illustrates the various labelling schemes for labelled liposomal amphotericin B preparations.
Based on numerous mechanistic studies, it is thought that AmBisome binds to the fungal cell wall where amphotericin B is released from the liposome, traverses through the cell wall and binds to ergosterol in the fungal cell membrane. Armed with an arsenal of fluorescent dyes and dye conjugated lipid moieties, investigators have consistently found that in a number of in vitro model systems, fluorescently labelled dye-encapsulated AmBisome preferentially accumulate at the surfaces of the fungal cell wall of C. albicans, A. fumigatus (Adler-Moore and Proffitt Citation1993, Adler-Moore Citation1994), Saccharomyces cerevisiae (Shimizu et al. Citation2010), and pulmonary Aspergillus (Lestner et al. Citation2010). It is not entirely clear which mechanism governs the attraction of liposomes to the fungal cell wall. But one clue may lie in the composition of the cell wall. Chavan et al. (Citation2012) reports that fluorescently labelled liposomes (as visualized by confocal microscopy) showed enhanced fluorescence along the surfaces of A. fumigatus following incubation with caspofungin, which promotes expression of β-glucans at the cell wall. In contrast, cells pretreated with a soluble β-glucan competitor, laminarin, showed a decrease in fluorescence at the fungal cell surface as a result of interference with binding of liposomes to the cell wall. β-glucans are covalently-linked polysaccharides that serve as major structural components of the fungal cell wall and account for approximately 30–80% of mass of the wall (Free Citation2013) depending on the strain. Interestingly, pretreatment with laminarin was more effective at prolonging the survival of A. fumigatus than an absence of laminarin, suggesting β-glucans may be an important factor that attracts liposomes to the fungal cell wall. Another possibility that has not yet been completely explored is the hypothesis that the liposomes binding to fungal surfaces might be mediated by surface charge attractions.
At the ultrastructural level, Adler-Moore demonstrated by freeze-fracture electron microscopy that gold particles bound to lipids incorporated into liposomes, with or without drug, localized to the surfaces of the fungal cell wall (Adler-Moore, Citation1994, Adler-Moore and Proffitt Citation1998). Only fungal cultures treated with AmBisome exhibit gold-conjugated lipids penetrating the cell wall and dispersed throughout the cytoplasm of A. fumigatus cells, presumably after disruption of the cell membrane. In contrast, fungal cells that are treated with liposomes absent of the drug show intact liposomes accumulate largely at the cell wall surface. More recent evidence from to-be published research by Gow (personal correspondence) demonstrates by high resolution electron-microscopy that gold-labelled AmBisome is capable of passing through the cell wall of C. albicans as intact liposomes, confirming the earlier observations by Adler-Moore. On the contrary, liposomes without the drug are retained by the outer cell wall layer. Cell wall mutant cell lines expressing varying cell wall thickness are susceptible to AmBisome following 24 h incubation (personal communications by Neil Gow). This work also studies the impact of ergosterol related mutants on MIC of AmBisome relative to wild type. A particularly intriguing finding is the discovery that cells expressing ergosterol mutants are found to be less susceptible to AmBisome with a 16-fold increase in minimal inhibitory concentration (MIC 16 µg/ml) in contrast to wild-type. Various alternate mechanisms by which liposomal amphotericin B exerts its fungicidal activity is depicted in .
Figure 2. Alternate modes of liposome fungal interaction with the fungal membrane involves (A) amphotericin B leaving the liposome that is perturbed by fungal well and binds to ergosterol in the fungal membrane, or (B.1) the liposome traverses through the fungal cell wall to (B.2) to fuse with the fungal cell membrane and facilitate transference of amphotericin B.
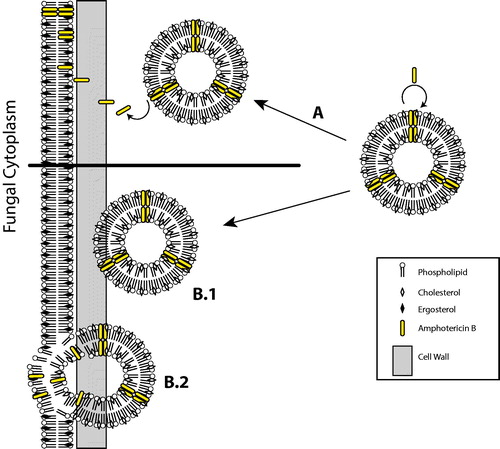
An advanced understanding underlying the mechanism by which amphotericin B is released from AmBisome upon contact with the fungal membrane remains lacking. Progress was made by Shimizu et al. in a report of promising results that provides additional data on the drug transfer mechanism and the temperature-dependence and sterol-specificity. AmBisome effectively inhibits S. cerevisiae colony formation at 35 °C but not at 4 °C up to three hours of incubation (Shimizu et al. Citation2010). This finding corroborates basic understanding of the gel to liquid crystal transition of the bilayer and observations by confocal microscopy that the dispersal of fluorescently-labelled AmBisome is detected only in cells at 35 °C following 24 h incubation. The importance of temperature on the drug transfer mechanism is further confirmed by evaluating the effects on sterol affinity to amphotericin B. The addition of liposomes engineered with ergosterol and amphotericin B to yeast cultures results in substantially less efficacious killing than compared to use of AmBisome at 35 °C, indicating that the stronger avidity of ergosterol to amphotericin B effects its ability to kill, most likely by greatly reducing the thermodynamic driver of transfer. Nevertheless, it is likely that ergosterol plays a role in further facilitating the temperature-dependent mechanism of transferring the drug to the fungal cell membrane. The fluidity of the liposome membrane was also evaluated using amphotericin B liposomes composed of highly fluid phospholipids, egg yolk phosphatidylcholine (EPC) and egg yolk phosphatidylglycerol (EPG), in place of HSPC and DSPG, respectively. Liposomes composed of EPC and EPG exhibit increased membrane fluidity when compared to AmBisome. Investigators found that EPC-, EPG-liposomes containing drug are potent at killing yeasts incubated in either 35 °C or 4 °C, demonstrating that membrane fluidity is a key factor effecting the transfer of amphotericin B to the membrane. By confocal microscopy, fluorescently-labelled lipids from EPC-, EPG-liposomes are observed dispersed throughout the yeast cytoplasm at both temperatures following 3 h incubation, quicker than that observed for AmBisome. Taken together, these results help support the model that the mechanistic basis of the ampohotericin B transfer from the liposome to the fungal membrane is dependent on membrane fluid dynamics and binding affinities of specific sterols.
Until recently, amphotericin B remains for years the gold-standard drug of choice and powerful last line of defense in the treatment of life-threatening systemic fungal infections because of its uncanny ability to outwit antibiotic resistance in spite of widespread therapeutic application. Only on rare instances have fungal isolates resistant to amphotericin B been reported. It is thought that the reduction in ergosterol content confers resistance to these cells, which is supported by data correlating reduction of drug susceptibility to a reduction in ergosterol content as observed in resistant isolates (Balkis et al. Citation2002). Loss of ergosterol obviously comes at a cost to the organism. Overall there is concern about fungal resistance as exemplified by the global outbreak of multi-drug resistant fungal species that recently has been reported. The sudden emergence of a new Candida strain, Candida auris, first detected in 2009 from an ear canal infection in Japan, is quickly becoming a growing threat across three continents (South America, Africa and Asia) (Lockhart et al. Citation2017). C. auris is a highly virulent strain of a yeast pathogen that can cause life-threatening invasive fungal infections. Out of the 54 isolates collected from patients, 35% show intrinsic resistance to amphotericin B and 93% are resistant to fluconazole, a first-line treatment agent against Candidiasis (Lockhart et al. Citation2017). A particularly grave concern is the discovery of C. auris isolates that are resistant to both fluoconazale and amphotericin B with the potential for a devastating outbreak. The capacity for C. auris to form biofilms on surfaces predicts its ability to resist a wide spectrum of antifungal agents and enhanced effectiveness to cause outbreaks (Sherry et al. Citation2017). This mechanical form of resistance may be more amenable to resistance to amphotericin B. Studies with liposomal amphotericin B are seeking to address both the ability to target biofilms and emerging resistant strains.
Conclusions
Collectively, what we can adduce from the targeting behaviour of AmBisome is that the drug’s potency depends on its ability to reach the fungal membrane at sufficiently high levels to be effective. Although the actual mode of action requires further investigation, it stands now that clearly fungicidal action depends on the membrane content of ergosterol. With the increasing incidence of multi-drug resistant pathogenic fungal strains, progressing our mechanistic understanding of antifungal agents such as amphotericin B will be an important strategy to developing improved use of existing agents and a battery of potent agents able to combat these virulent strains in the future.
Acknowledgements
The author thanks Professor Neil A. R. Gow from the College of Life Sciences and Medicine, University of Aberdeen, for the research of gold-labelled liposomes in C. albicans mutants and Tarquinus H. Bunch for providing the illustrations.
Disclosure statement
The author reports no conflict of interest. The author alone is responsible for the content and writing of the article.
References
- Adler-Moore, J., 1994. AmBisome targeting to fungal infections. Bone Marrow Transplantation, 14 (Suppl5), S3–S7.
- Adler-Moore, J., Proffitt, R.T., 1993. Development, characterizations, efficacy and mode of action of AmBisome®, a unilamellar liposomal formulation of amphotericin B. Journal of Liposome Research, 3 (3), 429–450.
- Adler-Moore, J., Proffitt, R.T., 1998. AmBisome®: long circulating liposomal formulation of amphotericin B. In: Long Circulating Liposomes: Old Drugs, Therapeutics, Berlin, Heidelberg: Springer-Verlag and Landes Bioscience, Chapter 13, 185–206.
- Adler-Moore, J., et al., 2017. Tissue pharmacokinetics and pharmacodynamics of AmBisome® (L-AmBis) in uninfected and infected animals and their effects on dosing regimens. Journal of Liposome Research, [Epub ahead of print]. doi: 10.1080/08982104.2017.1327543
- Alcazar-Fuoli, L., Mellado, E., 2013. Ergosterol biosynthesis in Aspergillus fumigatus: its relevance as an antifungal target and role in antifungal drug resistance. Frontiers in Microbiology, 3 (439), 1–6.
- Anderson, T.M., et al., 2014. Amphotericin forms an extramembranous and fungicidal sterol sponge. Nature Chemical Biology, 10 (5), 400–406.
- Balkis, M.M., et al., 2002. Mechanisms of fungal resistance: an overview. Drugs, 62 (7), 1025–1040.
- Chavan, N.L., et al., 2012. Interactions of liposome carriers with infectious fungal hyphae reveals the role of β-glucans. Molecular Pharmaceutics, 9, 2489–2496.
- Free, S.J., 2013. Fungal cell wall organization and biosynthesis. Advances in Genetics, 81, 33–82.
- Gow, N.A.R., personal communication.
- Gray, K.C., et al., 2012. Amphotericin primarily kills yeast by simply binding ergosterol. Proceedings of the National Academy of Sciences of the United States of America, 109 (7), 2234–2239.
- Groll, A.H., et al., 2000. Comparative efficacy and distribution of lipid formulations of amphotericin B in experimental candida albicans infection of the central nervous system. The Journal of Infectious Diseases, 182, 274–282.
- Khutorsky, V.E., 1992. Structures of amphotericin B-cholesterol complex. Biochimica et Biophysica Acta, 1108, 123–127.
- Lestner, J.M., et al., 2010. Pharmacokinetics and pharmacodynamics of amphotericin B deoxycholate, liposomal amphotericin B, and amphtoericin B lipid complex in an in vitro model of invasive pulmonary aspergillosis. Antimicro Agents and Chemother, 54, 3432–3441.
- Lockhart, S.R., et al., 2017. Simultaneous emergence of multidrug-resistant Candida auris on 3 continents confirmed by whole-genome sequencing and epidemiological analyses. Clinical Infectious Diseases, 64, 135–140.
- McCarthy, M., et al., 2014. Mold infections of the central nervous system. The New England Journal of Medicine, 371 (2), 150–160.
- Mesa-Arango, A.C., Scorzoni, L., Zaragoza, O., 2012. It only takes one to do many jobs: Amphotericin B as antifungal and immunomodulatory drug. Frontiers in Microbiology, 3 (286), 286.
- Moore, E.M., Lockwood, D.N., 2010. Treatment of visceral leishmaniasis. Journal of Global Infectious Diseases, 2 (2), 151–158.
- Murata, M., et al., 2009. Ion channel complex of antibiotics as viewed by NMR. Pure and Applied Chemistry, 81, 1123–1129.
- Oku, N., et al., 2000. Evaluation of drug targeting strategies and liposomal trafficking. Current Pharmaceutical Design, 6, 1669–1691.
- Palacios, D.S., Anderson, T.M., Burke, M.D., 2007. A post-PKS oxidation of the Amphotericin B skeleton predicted to be critical for channel formulation is not required for potent antifungal activity. Journal of the American Chemical Society, 129 (45), 13804–13805.
- Palacios, D.S., et al., 2011. Synthesis-enabled functional group deletions reveal key underpinnings of amphotericin B ion channel and antifungal activities. Proceedings of the National Academy of Sciences of the United States of America, 108 (17), 6733–6738.
- Paterson, P.J., et al., 2003. Treatment failure in invasive Aspergillosis: susceptibility of deep tissue isolates following treatment with amphotericin B. The Journal of Antimicrobial Chemotherapy, 52, 873–876.
- Sherry, L., et al., 2017. Biofilm-forming capability of highly virulent, multidrug-resistant Candida auris. Emerging Infectious Diseases, 23 (2), 328–331.
- Shimizu, K., et al., 2010. Temperature-dependent transfer of amphotericin B from liposomal membrane of AmBisome to fungal cell membrane. Journal of Controlled Release: Official Journal of the Controlled Release Society, 141, 208–215.
- Stone, N.R.H., et al., 2016. Liposomal amphotericin B (AmBisome®): a review of the pharmacokinetics, pharmacodynamics, clinical experience and future directions. Drugs, 76 (4), 485–500.
- Takemoto, K., et al., 2006. Comparative study on the efficacy of AmBisome and Fungizone in a mouse model of pulmonary aspergillosis. Journal of Antimicrobial Chemotherapy, 57, 724–731.