Abstract
In grassland areas where herbage production has no economic value, the cut grass is often left on the sward surface where its decomposition is influenced by weather conditions. Although the influence of temperature and humidity on decomposition has been investigated under controlled lab conditions, experimentation has generally been under ideal moisture conditions that have not tested the combinations of climatic limitations that might occur in the field. The decomposition of mown turfgrass clippings deposited at different times of vegetation period was studied in situ using nylon bags during the first 8 weeks after deposition to investigate the effect of weather conditions (the air temperature, relative humidity, precipitation) on decomposition. Decomposition is the highest in the case of high air humidity and temperature of 10°C. Limiting factors for decomposition at temperatures above 10°C is the air humidity and below 10°C the air temperature. The general tendency was that the rate of decomposition increased with increasing air temperature up to 10°C, but with further increases of air temperature the decomposition rate slowed down. Relative air humidity had a variable impact (at the beginning of the decomposition process (weeks 1–2) the influence was negative, during weeks 3–8 of the decomposition process the effect was positive), and hence had no generalized relationship with decomposition over the studied decomposition period (weeks 1–8). The most significant influence of weather conditions on the decomposition rate was recorded directly after cutting. If the cutting was done during hot weather conditions, the material was drying fast and therefore decomposed slowly. Our results indicate that for fast decomposition of clippings it is important to maintain the freshness of material. Lower decomposition rates occurred during conditions of hot and dry weather, and also cooler (temperature near to 0°C) weather, and can be compensated as soon as favourable weather arrives.
Introduction
In amenity grassland where there is a requirement to mow grassland for maintenance, the mown grass clippings often have no economic value and are left on the sward surface, where their decomposition is influenced by weather conditions. In temperate zones the weather conditions change during the year and could have a direct influence on the rate of decomposition of organic matter. During periods of unfavourable conditions for decomposition, residues of grass clippings left on the sward surface could tend to accumulate, partly because their deposition exceeds decomposition rate. To avoid these problems, and to ensure good turfgrass management in the absence of agronomic productivity, it is important to know the rates of decomposition of grass residues left on the sward surface during different growing stages.
Temperature and moisture conditions are the main factors influencing the activity of decomposers involved in the decomposition process (Paul and Clark Citation1996, Dalias et al. Citation2001a, Citation2001b, Pietikäinen et al. Citation2005, Uvarov et al. Citation2006). During the process of decomposition of organic matter an increase in soil temperature and moisture generally results in greater rates of microbial activity and thus increased rates of reduction of plant residue (Stott et al. Citation1986, Donnelly et al. Citation1990). Microbial activity is generally predicted to increase rapidly up to a temperature of about 30°C. An optimal temperature for microbial activity is reached between 35 and 45°C, and the optimal moisture content for organic matter decay is 50–60% (McKinley and Vestal Citation1985, Chen et al. Citation2000). It is found that at higher temperatures the temperature influence to the decomposition rate is reduced i.e. increasing temperature by the same number of degrees at lower temperatures accelerated decomposition more than the same increase at higher temperatures (Kirschbaum Citation1995, Dalias et al. Citation2001a).
The influence of temperature and humidity on decomposition has been investigated mostly under controlled laboratory conditions and in field experiments where plant residues are incorporated into soil (Kirschbaum Citation1995, Henriksen and Breland Citation1999, Dalias et al. Citation2001a). Laboratory experiments that focus on temperature responses have been generally conducted under ideal moisture conditions, so that the various combinations of climatic limitations to decomposition that might occur in the field have not necessarily been tested. Field tests carried out in soil have shown there are strong interactive effects of temperature and moisture on litter respiration during litter decomposition (Flanagan and Veum Citation1974, Clark and Gilmour Citation1983, Doel et al. Citation1990, O'Connell Citation1990).
Plant residues spread on the soil surface will normally be exposed to more variable temperature and moisture conditions than will residues buried in the soil, and these variable conditions may greatly slow down the decomposition of organic residues on the soil surface. For example, Curtin et al. (Citation1998) found that CO2 evolution was 36–62% less from soil being exposed to drying/wetting cycles compared with soil having adequate constant moisture content. In natural conditions decomposition of organic matter on the surface of soil is influenced by air temperature, air relative humidity and precipitation, in addition to the influences of soil temperature and moisture content, which as a rule are different from the respective parameters for air (Quemada and Cabrera 1995). Close contact with soil will usually increase the microbial decomposition of organic matter (Douglas et al. Citation1980, Cogle et al. Citation1989, Havstad et al. Citation2010) and this is mainly due to higher moisture content in residues (Parr and Papendick Citation1978).
Even though the influence of temperature on decomposition has been the focus of many investigations, the decomposition of mown plant residues at different periods in the growing season has received little attention. There is also little information about the effect of weather conditions on decomposition of fresh organic material. The moisture content in fresh grass material is high at the starting point of decomposition and it is probably very favourable material for the microbes so that they can start the decomposition process immediately. Therefore, we can assume that at the beginning of decomposition the rate of decomposition depends only on temperature, because the moisture needed for the decomposition process is adequate. The influence of temperature can occur in two ways: temperature activates the decomposers, and at the same time it also dries the material causing moisture to become a limiting factor as temperatures increase. The objective of the research was to investigate the influence of air temperature, relative air humidity and precipitation on the dynamics of decomposition. We hypothesized that turfgrass clippings mowed at different times during the growing season will decompose at different rates, and that these rates would be mainly influenced by different weather conditions during these periods.
Materials and methods
Background of experimental site
The field experiment was carried out at the Experimental Station Eerika of the Estonian University of Life Sciences (58°23′32′′ N latitude, 26°41′31′′ E longitude). The soil of the experimental field was a Stagnic Luvisol according to WRB classification (FAO, ISSS, ISRIC Citation1998). Soil analyses by establishing the trial showed that the humus horizon contained 16.0 mg organic carbon g−1 and 1.63 mg N g−1. The sward had been established in June 2003 with a turfgrass mixture of Festuca rubra rubra (198 kg ha−1 germinating seed) and Poa pratensis (52 kg ha−1), the mixture providing a 50:50 ratio of germinating seeds by seed number of Festuca rubra rubra and Poa pratensis. The sward was unfertilized during the period between the sward establishments in 2003 and in May 2004 when in those plots the research was initiated to demonstrate the influence of returned clippings to the yield and growth of sward plants.
The experimental design was a randomized complete block with four replicates of each of four fertilization treatments, with a plot size 1×7 m for each treatment plot. The fertilizer treatments were as follows: N0P0K0 (N0) as control, N80P11K48 (N80), N160P22K96 (N160) and N400P56K240 (N400) kg ha−1. The N as (NH4)NO3 and K as KCl fertilizer was applied by hand to the plots (a plot size 7 m2) in 2 to 4 splits depending on the ratio during vegetation period. The P fertilizer as Ca(H2PO4)2 was applied to the plots in 1 split at the beginning of May. The turfgrass sward was mown with a rotary lawn mower (Partner 5553 CMDEW) with a bag attachment at 5 cm height when the height of grass was approximately 7–8 cm high. The frequencies of mowing depended on the rates of grass growth. The clippings from each plot were returned to that plot and then mulched with a lawn mower.
The research about decomposition process of clippings was carried out in years 2006 and 2007. We analysed the decomposition process of clippings in four different fertilization treatments (N0, N80, N160, N400) having four replications in each treatment. The research on clippings decomposition was simultaneously implemented together with research on clippings influence on sward and therefore the methodology of the trial was the same in both cases.
Arrangement of experiment
There were four experimental periods, each of 8–10 weeks duration (referred to as Period I, II, III and IV) ().
Table I. Time of experiment, the duration of decomposition periods and sampling weeks.
At the beginning of each period (15 May, 13 Sept, 26 Oct in 2006 and 16 May in 2007), directly after cutting, a sample of 100 g of fresh herbage was collected. From each sample, a subsample of 20 g of fresh herbage (the length of the plant species was 2–3 cm) was put in to 20×20 cm nylon bags with a 1.5 mm mesh size. Each of the bags with the fresh clippings were placed and fixed with clamps into the thatch layer of the plot from which it had been harvested. The number of bags used, per treatment, was between 12 and 20 in different periods and it depended on how many times the bags were planned to be removed from the plots. For example in period I the bags were removed four different times during 8 weeks that means every fertilizing variant received altogether 16 bags (4 bags for every replication). Weekly mowing took place even when studying the decomposition of clippings. The nylon bags didn't disturb the mowing because they were fixed tightly to the soil surface. The mowing height was 5 cm and the mower did not touch the nylon bags. Bags were removed according to a certain timetable (). Weeks were counted since the day when the bags were placed on the experimental plot. The removal of bags was taking place on the 7th day of the week. Simultaneously four bags were removed from each fertilization treatment (1 bag per replicate of a treatment).
Measurements analysis
The remainder part of the sample at the beginning of experiment was used to determine (i) dry matter, (ii) total nitrogen, (iii) total carbon, (iv) cellulose and (v) lignin content.
At retrieval, the content of each bag was carefully examined and visible soil particles were removed continued by determination of cellulose and lignin content of the remaining plant residue.
The dry matter content was determined by drying the sample in a forced-draught oven for 6 hours at 105°C. Total nitrogen and carbon content were analysed by dry combustion method in a varioMAX CNS elemental analyser (ELEMENTAR, Germany). Van Soest's method was used to measure the cellulose and lignin content on a dry matter basis (Van Soest Citation1963).
Equations for calculations
Dry matter
where:
M0 is the initial plant material dry matter mass in the bag;
Mt is plant material dry matter mass in bag in time t, when bags were removed from field.
Weather
The climate of Estonia is almost maritime in the west and slightly continental in the east. The winter period (average air temperature permanently below 0°C) lasts on average 115 days with an average mean temperature of the coldest months of −5.5°C. The average duration of the vegetation period (air temperature permanently above 5°C) is 175–190 days. The average period without night frosts is four months, during which time the average midsummer (July) temperature is 16–17°C. Mean annual precipitation is 550–700 mm; the average precipitation in the wettest months (April to the end of October) is 350–500 mm (Keppart and Loodla Citation2006). Throughout the experiment period we monitored the meteorological conditions at the experimental site using Metos Model MCR300 weather stations (Pessl Instruments GmbH, Weiz, Austria). The sensors were positioned two metres above the ground.
In the current research we gathered the information about average day air temperature (°C), relative air humidity (%) and precipitation (mm). By results the weather parameters are presented for periods I–IV and decomposition weeks (weeks 1–2, weeks 3–8, weeks 1–8) ().
Table II. The dry matter (DM) content, nitrogen (N), carbon (C), cellulose and lignin initial concentration and C:N ratio in clippings at the beginning of Periods I–IV.
Table III. Weather parameters for test periods.
Statistical analysis
Statistical analyses were carried out using software Statistica version 7.0 (StatSoft Inc.). Analysis of variance (ANOVA) for a randomized complete design was used to test the influence of N fertilization on decomposition. In the figures presented the value of standard error (SE) was found by using the two-way unweighted means analysis, where the factors were: (i) dates of sampling and (ii) rates of nitrogen fertilization.
The linear and multiple regression analysis with backward stepwise were performed to evaluate the relationships between the weight loss and different weather parameters (average air temperature, air relative humidity and precipitation of each period).
Results
Chemical composition of turfgrass clippings
The DM content and chemical compositions of turfgrass clippings during the different periods are presented in .
Weather
Summarized weather parameters for the whole decomposition process and different periods of the decomposition process are presented in .
Decomposition of turfgrass clippings
The decomposition process of clippings from four different fertilization treatments was similar in all analysed experimental periods. The fertilization rate did not affect the decomposition process significantly (F(3,343)=0.024; p =0.99).
Decomposition of turfgrass clippings during different periods showed the same pattern of decomposition but the decomposition rates were different (p <0.05). The fastest decomposition during the 8-week period was recorded for material cut in late summer on 13 September (). The slowest rate was observed during the decomposition which started in October, which is middle of the autumn in Estonia. The difference in decomposition rates was most apparent during the first two weeks of each decomposition period. During the following weeks the variations between decomposition periods were smaller. In all compared periods the decomposition rates were highest directly after deposition of clippings and slowed down during the following weeks. When approximately 40% of the initial material still remained in the bag the decomposition process slowed down considerably. The material from harvested plots of different fertilization treatments decomposed at similar rates ().
Figure 1. Turfgrass clippings weight loss, as percentage (%) of the initial dry matter (DM) weight, during decomposition in spring–summer 2006 (Period I), in autumn (Period II), autumn–winter (Period III) and in spring–summer 2007 (Period IV). Bars indicate confidence limits at p<0.05.
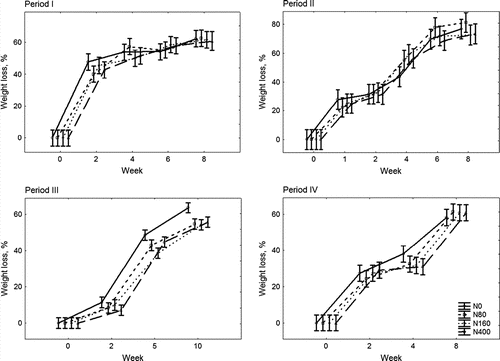
The cellulose and lignin decomposition was studied for the herbage during Period IV only. The cellulose in the turfgrass clippings started to decompose 4 weeks after cutting, when on average 33% of the initial mass had already decomposed (). The initial content of cellulose (179.4 mg g−1) had decreased by the end of the investigated period to 88.8 mg g−1. The initial content of lignin in the decomposing material was 12.4 mg g−1. During 8 weeks (the period of decomposition in this trial) the lignin was not decomposing and its concentration was increasing during successive weeks. By week 8 the content of lignin had increased up to 154.0 mg g−1.
Influence of weather conditions on decomposition of clippings
Air temperature was the only measured weather parameter that showed a significant influence on the decomposition of turfgrass clippings during an 8-week period (R2=0.97; ). The general tendency was that increasing the air temperature to 10°C resulted in an increase in the rate of decomposition, but further increases in air temperature resulted in a slowing down of the decomposition rate (). There were no significant relationships between relative air humidity or precipitation and weight loss over an 8-week period (p>0.05; ).
Figure 3. Regressions of mean temperature and weight losses at different times of the decomposition process. The points at different temperatures indicate weight losses of the decaying material from different fertilization treatments. A: Week 1–8 y = −0.32x2+6.33x + 43.08 R2=0.46*** (polynom). B: Week 1–2 y = −0.32x2+6.30x + 9.15 R2=0.83* (polynom). C: Week 3–8 y = −1.58x + 53.09 R2=0.48* (linear). * Significant at p<0.05. *** Significant at p<0.001.
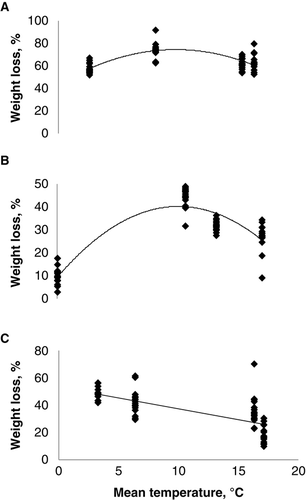
Table IV. Regressions of mean relative humidity and precipitation with weight losses at different times of the decomposition process.
In the spring periods of the two years (Period I and Period IV) at the start of the decomposition process the weather conditions were similar for both 8-week periods. In 2006, the average air temperature over 8 weeks was 15.4°C and relative air humidity was 76%. In 2007, those parameters were 16.5°C and 82%, respectively. In similar weather conditions the weight losses over 8 weeks were also similar, and in those years by 8 weeks after the cut the proportions of material that had decomposed were 61.9% and 60.3%, respectively. In the late summer period (Period II) the average air temperature over 8 weeks was lower (8.2°C) and relative air humidity compared with the spring periods was higher (96%), and in these weather conditions the rate of decomposition was the fastest. In period III the average air temperature during 8 weeks was 2.2°C, relative air humidity was the highest (98%) and under these conditions 57% of material had decomposed.
By 1–2 weeks of the decomposition process the relationship between air temperature and weight loss was similar to the relationship between air temperature and weight loss of the whole investigated period, and the optimal air temperature for decomposition of clippings was 10°C on average. The mean air temperatures of Period I weeks 1–2 were 10.4°C and of Period IV weeks 1–2 16.3°C. The weight loss during weeks 1–2 in the cooler temperatures of spring–summer 2006 was one-third greater than in the warm temperatures of spring–summer 2007, 43.9% and 27.1% of initial dry matter, respectively.
The rate of decomposition was also decreased under conditions of low temperatures. The slowest was the decomposition during late autumn (Period III) when the average air temperature of weeks 1–2 was 0.1°C. In Period II the average temperature of weeks 1–2 was 8.2°C and weight loss 32%, which was similar to Period IV.
The relationship between weight loss and relative air humidity was negative during weeks 1–2 (). The highest relative air humidity (91–97%) in this period was characteristic of the late summer and autumn periods (Periods II and III) and the lowest was the air humidity (74–80%) during spring periods. The significant relationship between precipitation and weight loss during weeks 1–2 was not observed.
After the second week of each period the decomposition rate was faster during the autumn periods (Period II and III). The average air temperature of weeks 3–8 of Period III was higher than during first 2 weeks, thereby resulting in a remarkable increase in decomposition rate. The slowest was the decomposition in weeks 3–8 of Period I, when the average air temperature was 17°C. Increasing the relative air humidity and precipitation had a positive influence on weight loss ().
Decomposition models
Climate parameters (temperature, humidity and precipitation) were tested to predict plant residues decomposition with a multiple regression model (). By weather parameters weight loss models were fitting better if every 8-week period was divided by two different time periods: 1–2 and 3–8 weeks. The multiple regression backward stepwise analysis revealed that air temperature will have a strong positive influence on decomposition process. The model that contained the average air temperature, relative air humidity and precipitation described well the speed of decomposition in weeks 1–2 and 1–8. In weeks 3–8 the weight loss model was the best if the average air temperature and precipitation were included in the model.
Table V. Results of multiple regression analysis with backward stepwise of the test between weight losses of different weeks and mean temperature, mean relative humidity and precipitation.
Discussion
The influence of air temperature and humidity on decomposition of fresh organic material
The rate of decomposition of turfgrass clippings depends mainly on the weather conditions during the decomposition period. The initial content of nitrogen and different C:N ratio in plant material did not affect the decomposition process through the whole trial period. Quemada and Cabrera (Citation1995) and Wagner and Wolf (Citation1999) have concluded that an increase in nitrogen content does not influence the process of decomposition if the C:N ratio of decomposable material is initially less than 20. In our trial the initial C:N ratio was less than 20 in all fertilization treatments, which was also the presumable cause for a similar decomposition process for the material derived from different fertilization plots. The influence of N fertilization rate on the clippings decomposition has been dealt in our previous paper (Kauer et al. Citation2007).
Air temperature and relative air humidity were important factors influencing the decomposition of turfgrass clippings on the sward surface. Previous investigations have revealed that by increasing temperatures up to 35°C the rate of organic matter decomposition will increase, and after that it stabilizes and then starts to decrease (McKinley and Vestal, Citation1985, Chen et al. Citation2000). Our results indicated that during decomposition of fresh material on the ground surface the optimal air temperature for decomposition was lower than previously reported and did not exceed 10°C. There was a reduction in the rate of decomposition at temperatures above and below 10°C. The research of Flanagan and Veum (Citation1974) showed that decomposition of organic matter can be limited by low air temperature as well as low moisture content, and increasing only one of these factors does not compensate fully for the influence of other limiting factors. Flanagan and Veum (Citation1974) also indicated that, in the case of decomposing material which had low moisture content (<50% of dry weight), temperature increases had little effect on decomposition, but at higher moisture content, respiration was more responsive to temperature changes. Similarly, they noted that moisture changes had little effect on litter decomposition at lower temperatures (<5°C), while at higher temperatures (10–15°C), decomposition was more responsive to moisture changes. Too little or too much water inhibited, or even stopped, litter decomposition due to matric limitation or oxygen diffusion limitation, respectively (Flanagan and Veum Citation1974, Clark and Gilmour Citation1983). Our research gave similar results. In our research the dynamics of moisture content in decaying material was not determined, but still we presume that by increasing the temperature above 10°C the low moisture content in decomposable material was becoming the limiting factor for the decomposition process. The fast drying of clippings is indispensable if they remain on the sward surface. To slow the drying process we must implement activities that promote rapid infiltration of the clippings into the canopy where the moisture content is higher. This is contributed by more frequent mowing. In this case the clippings which sift to the canopy are shorter in length and their reaching the soil surface is less hindered.
At temperatures below 10°C the limiting factor was the air temperature. The influence of air temperature on the decomposition process was the most important at the beginning of decomposition process (during first 1–2 weeks) when the rate of decomposition was highest. By increasing air temperature from the optimal (10°C), the weight loss by decomposing was slowed down and this effect was most likely caused by fast drying of material. Our results indicate that for fast decomposing of turfgrass clippings it is important to maintain the initial moisture content in the material because rainwater can not fully compensate for it. In Period IV the average air temperature during the first 2 weeks of decomposition process was 16°C. This was relatively higher than in other investigated decomposition periods. Even though the amount of the precipitation was also the highest, the weight loss stayed at a lower level compared with the other decomposition periods. The impact of moisture content of returned clippings at the beginning of decomposition is also indicated by the relationship between relative humidity and decomposition. At the beginning of the decomposition period (the first 1–2 weeks) when the material was still fresh, the relationship between air humidity and decomposition rate was negative (). But it was only an apparent effect because the highest rates of air humidity were determined in late autumn when the air temperature was very low and therefore the weight loss by decomposition was very small.
The second reason why the relationship between air humidity and decomposition rate seems negative is due to differences of the air humidity in spring and autumn. In spring (after winter) the content of the air humidity during the first 2 weeks of studied decomposition process is lower (74.1% Period I and 79.4% Period IV) than in autumn (90.3% for Period II and 97.4% for Period III). In spring the higher air temperature causes the higher air humidity due to the higher rate of evaporation from the soil. In summer and autumn the higher temperature decreases the air humidity. Due to the different temperature effect on the air humidity at the different times of the year the relationship between air humidity and decomposition rate was negative during the first 2 weeks of the decomposition process in our study. In summer and autumn the air humidity is more dependent on the air temperature and precipitation, which is why the decomposition during weeks 3–8 of the decomposition process is described mainly with the air temperature and precipitation. This means that to study the influence of weather conditions on the decomposition we should also take into account seasonal effects at different times of the year because the relationships between temperature and humidity may vary at different time of year.
The negative effect of air humidity on the decomposition process in the first weeks (1–2) and weeks 3–8 was the reason why the influence of air humidity was not significantly important on decomposition process in the whole period. From the beginning of the third week, when decomposable material had already dried, the relationship between weight loss and precipitation became positive, showing that in the case of dry material the moisture content is one of the important factors limiting the decomposition process. Henriksen and Breland (Citation1999) found that plant residues were decomposing intensively even though the average temperature of the whole investigation period stayed under the 0°C and did not rise above 2.4°C. Our research results indicated also that by temperatures around zero degrees (Period III) the decomposition process continued but the rate of decomposition of grass clippings was significantly slower than the decomposition rate at higher temperatures. However, our results do not enable us to determine that the decomposition process continued at 0°C, or even by lower temperatures, because during that decomposition period there were days when the average temperature exceeded 5°C. It is possible that the majority of the decomposition process occurred during short periods when the temperatures were higher than 0°C. Due to variability in temperatures, average temperature cannot be considered a good indicator to characterize specified time periods for evaluating the influence of air temperature on decomposition. This assumption is true for late autumn, winter and early spring periods when average temperatures remain around 0°C but there are wide variations between night and day temperatures.
The influence of air temperature and humidity on easily decomposable compounds and cellulose
Our results indicated that during the first 2 weeks, when it was mainly the more easily decomposable compounds that were decomposing under conditions where the content of moisture was not the limiting factor, the rate of decomposition increased remarkably in response to increasing temperatures from 0 to 10°C. In such conditions the influence of increasing temperatures on the decomposition of easily decomposable compounds was especially noticeable at lower temperatures. In Period III, when the temperature was increasing from 0.1 to 3.2°C, the weight loss also increased remarkably as temperatures increased. Our results revealed that the decomposition of easily decomposable compounds depends on both air temperature and relative humidity. Andersen and Jensen (Citation2001) investigated decomposition of previously dried plant residues at three different temperatures (3, 9 and 15°C) and constant air humidity and found that the decomposition of easily decomposable compounds (i.e. water-soluble compounds) was less dependent on temperature than was the decomposition of more slowly decomposable compounds (i.e. cellulose and lignin).
Several studies have concluded that the decomposition of slowly decomposable structural compounds is sensitive to changes in temperature, and the decomposition of such compounds by lower temperatures is more limited than by higher temperatures (De Neve et al. Citation1996, Nicolardot et al. Citation1994, Bol et al. Citation2003). According to chemical kinetic theory, decomposition of recalcitrant, slowly decomposing substrates has higher activation energy, and thus higher temperature sensitivity (Bosatta and Agren Citation1999). In our experiment we analysed the content of cellulose and lignin in the clippings but during the study period only the decomposition of cellulose took place, which does not confirm the earlier findings, that to decompose the structural compounds the higher temperature is needed. The decomposition of cellulose started when the weight loss of the initial sample was 33%. In decomposition Period I, during the first 2 weeks 43% of the sample had already decomposed due to the fact that cellulose was also starting to decompose (assuming that clippings had the same chemical composition in both spring decomposition periods). The average temperature during the first 2 weeks of Period I was lower (10.4°C) than the average temperature in the first 2 weeks in Period IV (16.6°C). The decomposition of cellulose was more intensive if the decomposing material was more humid, because in Period I, due to lower air temperature, we can suppose that the material was not drying so fast as in Period IV. By continuing the decomposition process when the decaying material was already obtaining the characteristics of the surrounding environment (i.e. similar moisture content) the decomposition process is faster in conditions where the air temperature is lower and humidity higher. Donnelly et al. (Citation1990) investigated the decomposition of cellulose and lignin at different temperature conditions (4, 12, 24°C) and soil moisture contents (20, 40, 60%). According to their results the highest rates of cellulose and lignin were decomposed at the highest of the investigated air temperatures and highest soil moisture content. The same research also revealed that, at lower soil moisture contents, the activity of microbes decomposing the cellulose and lignin was not increasing in response to increasing temperature. They concluded that if moisture is a limiting factor, then microbes did not respond to increasing temperatures and in this case the soil moisture content is a more important factor influencing the decomposition process than temperature. The results of our investigation for decomposition in weeks 5–8 are in accordance with the results of Donnelly et al. (Citation1990). In decomposition Periods I and II until week 4, 53.6 and 53.1% was decomposed from the initial mass. The weight losses were similar, meaning that the material should contain similar amounts of different carbon compounds. The Periods I and II, weeks 5–8, the average air temperatures were 19.5 and 3.9°C and air humidity 73.0 and 97.7%, respectively. The decomposition in that time interval was faster in Period II, which was cooler and more humid than in Period I. Some other studies also support the finding that higher air temperatures do not increase the rate of the decomposition process, mainly due to lower moisture content in decomposing material (Giardina and Ryan Citation2000, Epstein et al. Citation2002, Aerts Citation2006).
Acknowledgements
This study was supported by Estonian Science Foundation grant No. 5751 and Estonian University of Life Sciences (project P6062PKPK06).
References
- Aerts , R. 2006 . The freezer defrosting: global warming and litter decomposition rates in cold biomes . Journal of Ecology , 94 : 713 – 724 .
- Andersen , M. K. and Jensen , E. S. 2001 . Low soil temperature effects on shortterm gross N mineralisation – immobilisation turnover after incorporation of green manure . Soil Biology and Biochemistry , 33 : 511 – 521 .
- Bol , R. , Bolger , T. , Cully , R. and Little , D. 2003 . Recalcitrant soil organic materials mineralize more efficiently at higher temperatures . Journal of Plant Nutrition and Soil Science , 166 : 300 – 307 .
- Bosatta , E. and Agren , G. I. 1999 . Soil organic matter quality interpreted thermodynamically . Soil Biology and Biochemistry , 31 : 1889 – 1891 .
- Chen , H. , Harmon , M. E. , Griffiths , R. P. and Hicks , W. 2000 . Effects of temperature and moisture on carbon respired from decomposing woody roots . Forest Ecology and Management , 138 : 51 – 64 .
- Clark , M. D. and Gilmour , J. Y. 1983 . The effect of temperature on decomposition at optimum and saturated soil water contents . Soil Science Society of America Journal , 47 : 927 – 929 .
- Curtin , D. , Selles , F. , Wang , H. , Campbell , C. A. and Biederbeck , V. O. 1998 . Carbon dioxide emissions and transformation of soil carbon and nitrogen during wheat straw decomposition . Soil Science Society of America Journal , 62 : 1035 – 1041 .
- Cogle , A. L. , Saffigna , P. G. and Strong , W. M. 1989 . Carbon transformation during wheat straw decomposition . Soil Biology and Biochemistry , 21 : 367 – 372 .
- Dalias , P. , Anderson , J. M. , Bottner , P. and Coûteaux , M. M. 2001a . Temperature responses of carbon mineralisation in conifer forest soils from different regional climates incubated under standard laboratory conditions . Global Change Biology , 6 : 181 – 192 .
- Dalias , P. , Anderson , J. M. , Bottner , P. and Coûteaux , M. M. 2001b . Long-term effects of temperature on carbon mineralisation processes . Soil Biology and Biochemistry , 33 : 1049 – 1057 .
- De Neve , S. , Pannier , J. and Hofman , G. 1996 . Temperature effects on C- and N mineralization from vegetable crop residues . Plant and Soil , 181 : 25 – 30 .
- Doel , D. S. , Honeycutt , C. W. and Halteman , W. A. 1990 . Soil water effects on the use of heat units to predict crop residue carbon and nitrogen mineralization . Biology and Fertility of Soils , 10 : 102 – 106 .
- Douglas , C. L. , Allmaras , R. R. , Rasmussen , P. E. , Ramig , R. E. and Roager , N. C. 1980 . Wheat straw composition and placement effects on decomposition in dryland agriculture of the Pacific Northwest . Soil Science Society of America Journal , 44 : 833 – 837 .
- Donnelly , P. K. , Entry , J. A. , Crawford , D. L. and Cromack , K. Jr. 1990 . Cellulose and lignin degradation in forest soils: Response to moisture, temperature and acidity . Microbial Ecology , 20 : 289 – 295 .
- FAO, ISSS, ISRIC , 1998 . World reference base for soil resources . World Soil Resources Report 84 , Rome .
- Epstein , H. , Burke , I. and Lauenroth , W. 2002 . Regional patterns of decomposition and primary production rates in the US Great Plains . Ecology , 83 : 320 – 327 .
- Giardina , C. P. and Ryan , M. G. 2000 . Evidence that decomposition rates of organic carbon in mineral soil do not vary with temperature . Nature , 404 : 858 – 861 .
- Flanagan , P. W. and Veum , A. K. 1974 . “ The influence of temperature and moisture on decomposition rates in tundra ” . In Soil organisms and decomposition in tundra , Edited by: Holding , A. J. , Heal , O. W. , MacLean , S. F. and Flanagan , P. W. 249 – 278 . Stockholm : IBP Tundra Biome Steering Committee .
- Havstad , L. T. , Aamlid , T. S. and Henriksen , T. M. 2010 . Decompostion of straw from herbage seed production: Effects of species, nutrient amendments and straw placement on C and N net mineralization, Acta Agriculturae Scandinavica Section B . Plant and Soil Science , 60 : 57 – 68 .
- Henriksen , T. M. and Breland , T. A. 1999 . Decomposition of crop residues in the field: evaluation of a simulation model developed from microcosm studies . Soil Biology and Biochemistry , 31 : 1423 – 1434 .
- Kauer , K. , Köster , T. , Raave , H. , Selge , A. , & Viiralt , R. 2007 . The influence of cut and returned plant material decomposition on the soil properties in swards with different fertilization rates . In NJF Report: Trends and perspectives in agriculture . Copenhagen, June 26–29, 2007. Copenhagen, 3 ( 2 ), 79 – 80 .
- Keppart , L. , & Loodla , K. 2006 . The potential of Estonian agro-climate and extraordinary weather situations during the last 50 years . — http://www.cosis.net/abstracts/EMS2006/00422/EMS2006-A-00422.pdf
- Kirschbaum , M. U. F. 1995 . The temperature dependence of soil organic matter decomposition, and the effect of global warming on soil organic C storage . Soil Biology and Biochemistry , 27 : 753 – 760 .
- McKinley , V. L. and Vestal , J. B. 1985 . Physical and chemical correlates of microbial activity and biomass in composting municipal sewage sludge . Applied and Environmental Microbiology , 50 : 1395 – 1403 .
- Nicolardot , B. , Fauvet , G. and Cheneby , D. 1994 . Carbon and nitrogen cycling through soil microbial biomass at various temperatures . Soil Biology and Biochemistry , 26 : 253 – 261 .
- O'Connell , A. M. 1990 . Microbial decomposition (respiration) of litter in eucalypt forests of southwestern Australia: An empirical model based on laboratory incubations . Soil Biology and Biochemistry , 22 : 153 – 160 .
- Parr , J. F. , & Papendick , R. I. 1978 . Factors affecting the decomposition of crop residues by microorganisms . In W. R. Oschwald , Crop residue management systems , pp. 101 – 129 . ASA Special Publication 31 . Madison , WI : ASA, CSSA, SSSA .
- Paul , E. A. and Clark , F. E. 1996 . Soil microbiology and biochemistry , New York : Academic Press: .
- Pietikäinen , J. , Pettersson , M. and Baath , E. 2005 . Comparison of temperature effects on soil respiration and bacterial and fungal growth rates . FEMS Microbial Ecology , 52 : 49 – 58 .
- Quemada , M. and Cabrera , M. L. 1995 . Carbon and nitrogen mineralization from leaves and stems of four cover crops . Soil Science Society of America Journal , 59 : 471 – 477 .
- Stott , D. E. , Elliott , L. F. , Papendick , R. I. and Campbell , G. S. 1986 . Low temperature or low water potential effects on the microbial decomposition of wheat residue . Soil Biology and Biochemistry , 18 : 577 – 582 .
- Uvarov , A. V. , Tiunov , A. V. and Scheu , S. 2006 . Long-term effects of seasonal and diurnal temperature fluctions on carbon dioxide efflux from a forest soil . Soil Biology and Biochemistry , 38 : 3387 – 3397 .
- Van Soest , P. J. 1963 . Use of detergents in the analysis of fibrous feeds. II. A rapid method for the determination of fibre and lignin . Journal of the Association of Official Analytical Chemists , 46 : 829 – 835 .
- Wagner , G. H. , & Wolf , D. C. 1999 . Carbon transformations and soil organic matter formation . In D. M. Sylvia , J. J. Fuhrmann , P. G. Hartel and D. A. Zuberer , Principles and applications of soil microbiology , pp. 218 – 258 . Upper Saddle River , NJ : Prentice Hall .