Abstract
The objective of this study was to determine how different afforestation strategies and slope positions affect soil organic carbon and total nitrogen. In a unique steepland formerly used as farmland in the Yangjuangou watershed of the Loess Plateau, three adjacent afforestation transects were selected as the sampling site: two single-species transects (Hippophae rhammoides and Artemisia capillaris), which each represented the single-species afforestation mode, and one mixed-species transect, which represented the multi-species afforestation mode. The results indicated that: (1) The soil organic carbon concentration in the multi-species afforestation mode was 25.8% and 27.7% higher than that in the other two single-species afforestation modes, while a minute difference in total nitrogen concentration was detected among the three afforestation transects. (2) Within the mixed-species and Hippophae rhammoides transect, both the soil organic carbon and total nitrogen concentrations showed an increasing trend from the upper slope position to the foot slope position. However, the soil organic carbon in the Artemisia capillaris transect was higher in the foot slope and upper slope but lower in the middle slope, demonstrating a “V” shape, and the total nitrogen increased from the foot slope position to the upper slope position. (3) The carbon : nitrogen ratios were no more than 10:1, which indicated the presence of easily decomposable soil organic matter. This study demonstrated the multi-species afforestation mode has better carbon accumulation potential compared with the single-species afforestation mode, which has important and informative implications for future vegetation restoration practices in the Loess Plateau and similar semi-arid environments.
Abbreviations
HR | = |
Hippophae rhammoides |
AC | = |
Artemisia capillaris |
MX | = |
mixed-species |
SOC | = |
soil organic carbon |
TN | = |
total nitrogen |
C:N ratio | = |
carbon-nitrogen ratio |
SSA | = |
single-species afforestation |
MSA | = |
multi-species afforestation |
GGP | = |
Grain for Green Project |
NFCP | = |
Natural Forest Conservation Program |
Introduction
In the last 50 years, a large portion of the forests and grassland has been depleted in China, resulting in a significant decline in soil quality (Liu and Diamond Citation2005). At present, land degradation has affected 23% of the total national land area (Bai and Dent Citation2009). Furthermore, because of centuries of improper land use, extreme natural disasters in China occurred more frequently in the late 20th century, such as the severe river floods of 1998 and annual sandstorms, water erosion, and mud flows. Since 2000, the Chinese central government has attempted to solve these environmental problems by aggressively launching two ambitious large-scale ecological restoration programs (Cao Citation2011). One was the Grain for Green Project (GGP), which retired and converted steeping farmland into ecological and commercial forests or grass so that deteriorated environments could be efficiently rehabilitated. The other program was the Natural Forest Conservation Program (NFCP), which reduced annual timber harvests from natural forests and afforested another tract of mountainside steepland by virtue of mountain closures and artificial planting (Yin and Yin Citation2009).
The Loess Plateau of China, owing to its extremely complex topography and severe water erosion, was selected as a pilot demonstration area for vegetation rehabilitation under the GGP and NFCP projects. In 2000, the soil loss rate in the Loess Plateau was reported to be 5000–10000 Mg km−2 per year, with some places even reaching 20000 Mg km−2 per year (Fu and Chen Citation2000). As a consequence of population expansion, severe soil erosion, caused primarily by poor vegetation and intensive cultivation, has resulted in land degradation as well as grain reduction (Fu and Gulinck Citation1994). At present, the total soil organic carbon (TOC) contents in the Loess Plateau average only 10 g C kg−1 (Wu et al. Citation2005, Chen et al. Citation2007).
Currently, multiple researchers have conducted studies on the effects of the GGP and NFCP on soil properties in the Loess Plateau region. However, most of them only focused on soil properties under a single-species afforestation (SSA) mode (i.e. carbon and nitrogen contents in the soil under a single tree species, planted on farmland slopes) (Wei et al. Citation2009, An et al. Citation2010, Fu et al. Citation2010, Liu et al. Citation2010, Zhang et al. Citation2011). Few, if any, studies concerned the soil properties under a mixed-species afforestation (MSA) mode (Zhang and Chen Citation2007). Because of the implementation of the GGP and NFCP projects, mixed-species afforestation (MSA) modes in the Loess Plateau are quite common. The GGP used MSA to highlight the importance of land conversions by establishing commercial and ecological forests of mixed species, such as apricots, peashrubs or Chinese pine, in the retired farm steeplands (Although GGP focused on converting retired farmland into commercial and ecological forests, grass and shrub cover were also selected as managed forms of reversion to fallow when appropriate.) Another program, the NFCP, also used MSA to stress the necessity for the conservation of natural forests by choosing Seabuckthorn (Hippophae rhammoides) for its thorny branches, which acted as a natural mountain closure by preventing humans from growing crops on the hills. The differences between these two afforestation modes can give rise to different plant residues in both quantity and quality, which predictably influences the amount of SOM and its utilization by microorganisms (Dube et al. Citation2009). Thus, the effects of MSA mode on soil properties should not be ignored by ecologists when evaluating the ecological effects of the GGP and NFCP.
In addition, much research cannot convincingly demonstrate the effects of afforestation on the content changes of SOM, SOC, and TN (Gong et al. Citation2006, Han et al. Citation2009, Liu et al. Citation2010, Jiao et al. Citation2011). First of all, some research failed to prove the comparability of afforested farmland slopes (Liu et al. Citation2010, Jiao et al. Citation2011). Different slope properties (i.e. gradient, slope shape, or slope length) may actually result in different quantities of soil nutrients removed or deposited by runoff and sediments. Moreover, different land-use histories, especially the different farming methods throughout years of cultivation, crop types and fertilizer application lead to variation in the initial SOM before afforestation begins (Kucharik and Jelinski Citation2009). Thus, if researchers cannot provide information regarding pre-afforestation conditions, readers may feel puzzled: did afforestation cause the difference in soil nutrients or were the original soil nutrients conditions different before afforestation? In short, to elucidate how afforestation affects soil properties, ecologists should recognize that soils are often considered a black box where multiple interacting processes take place (Andrén et al. Citation2008). Therefore, successful research in addressing this issue should satisfy one prerequisite: soil samples should be collected on the hillslopes with the same soil types, same slope and same land-use histories.
To address the aforementioned problem while gaining a better understanding of soil properties in MSA mode, a unique study site in the Yangjuangou watershed of the Loess Plateau was chosen to collect soil samples. This site was previously a cropland slope that grew maize and millet from 1949–1999. In 2000, under the enforcement of the GGP and NFCP, the slope was revegetated. Three adjacent afforestation transects (including two SSA modes and one MSA mode) in this slope were identified in 2008 as the sampling sites. Thus, our study can guarantee all afforestation transects shared the same land-use histories and slope properties. Soil properties in the MSA mode were presumed to be different from those in the SSA mode. Therefore, in order to test our hypothesis and provide scientific evidence for the comprehensive assessment of the GGP and NFCP projects, our objectives in this study were to understand how the different afforestation modes and slope positions affected the C:N ratios, and SOC and TN contents.
Materials and methods
Study site
This study was conducted in the Yangjuangou watershed (36° 42′ N, 109° 31′ E) located in the middle of the Loess Plateau, Shaanxi, China (). It comprises an area of approximately 2 km2 in the hilly-gully, loess region. Characterized by a semi-arid continental climate, this region has an average annual rainfall of 535 mm with 70% from July to September. There are significant topographic variations within the steep hills and deep ravines, creating an elevational difference between the highest and lowest point of 275 m. The gully density in this site is 2.74 km km−2. Soils in the study area originated mainly from loess with low anti-erodibility. Soil texture ranges from fine silt to silt (Fu et al. Citation2000, Citation2009).
Before 1999, the land use in the Yangjuangou watershed was dominated by crops. With the enforcement of the Grain for Green Project (GGP) in 2000, the widespread afforestation was implemented to control the severe soil erosion and rehabilitate the degraded environment. At present, nearly all the slope cropland was converted into forests or grasslands (Wang et al. Citation2011). The major afforestation species include Caragana korshinskii, Hippophae rhammoides, Prunus armeniaca, Robinia pseudoacacia, and Artemisia capillaris.
Sampling design and laboratory analysis
In this study, three adjacent afforestation transects (established in 2000) were identified within a single farmland slope, with a uniform gradient (24 degrees) and aspect (west), and with uniform morphological and genetic soil characteristics. As shown in , Transect 1 (the AC transect, sparse in middle slope) and Transect 2 (the HR transect, uniform coverage) represented SSA mode, covered by Artemisia capillaris and Hippophae rhammoides, respectively, from the foot slope position to the upper slope position; Transect 3, the mixed-species (MX) transect, represented MSA mode, covered with a combination of Prunus armeniaca (in the foot slope), Artemisia capillaris (in the middle), and Hippophae rhammoides (in the upper section).
Soil samples were collected from the three afforestation transects in June 2008 at six depth increments: 0–5 cm, 5–10 cm, 10–20 cm, 20–30 cm, 30–40 cm, and 40–50 cm. Every 10 m along the slope, one soil sampling site was set from foot to the upper section of the slope, for a total of six sampling sites in each transect. Three replicate soil samples in each sampling site were collected to help assess the within-transect variability of soil properties. Thus, 108 soil samples for line transect, a total of 324 soil samples altogether (6 sampling sites×6 depth increments×3 replications×3 line transects), were collected using a 20×5 cm soil auger. Besides, we have also collected three additional soil samples (0–10 cm) within each transect for soil physical properties measurement. Although MSA mode is common in the Loess Plateau, it was almost impossible to locate other retired farmland slopes with similar adjacent transects, slope properties and within reasonable proximity to serve as true replicates.
All soil samples were taken to the laboratory. Soil samples were grained and sieved through a 1mm screen (for measurement of soil chemical properties) and 2mm screen (for measurement of soil physical properties) respectively, then air-dried at room temperature. Soil bulk density was determined by the 100 cm3 ring tube method and soil water content was determined by the oven method (105 °C for 24 h). Soil pH and electrical conductivity were measured at a soil-to-water ratio of 1:2.5. SOC was measured using a potassium bichromate external heating method and TN was determined using the semimicro-Kjeldahl determination method (Bao Citation2000). Soil physical properties within each afforestation transect are presented in .
Table I. Soil pH, electrical conductivity, bulk density and water content in the 0–10 cm soil layer for Artemisia capillaris (AC), Hippophae rhammoides (HR) and mixed-species (MX) transect.
Data analysis
SPSS 18.0 was used for statistical analysis. One-way ANOVA was used to examine the effects of the slope positions and soil depths on the concentrations of SOC and TN within each transect. If significant effects were observed, a separation of means was performed using a least significant difference (LSD) test at p<0.05. The general linear model (GLM) was used to interpret the proportion of the total variation of the soil properties attributable to afforestation transects and slope positions. In addition, the correlation analysis was conducted to assess the relationship among soil variables within each transect.
Results
Soil organic carbon and total nitrogen concentrations
The SOC and TN concentrations decreased with increasing soil depth (). In each transect, both the SOC and TN concentrations exhibited significant differences (p<0.05) among different soil depths in the 0–30 cm soil layer while exhibiting no significant difference in the 30–50 cm soil layer. The highest SOC concentration was obtained in the MX transect for all depths in the 0–50 cm soil profile. However, although the overall SOC concentration of the AC transect (2.60 g kg−1) was similar to that of the HR transect (2.64 g kg−1), they were not always alike at each soil depth. The overall TN concentration was highest in the MX transect (0.40 g kg−1), yet AC and HR had TN content similar overall to MX (0.37 g kg−1 and 0.38 g kg−1 respectively). Not surprisingly, the SOC and TN concentrations were highly correlated in soil profiles of each of the three transects with the following correlation coefficients (r): AC, 0.98; HR and MX, 0.99 (p<0.01).
Table II. Soil organic carbon (SOC) and total nitrogen (TN) concentrations (g kg−1) at different depths and their correlations (r) for Artemisia capillaris (AC), Hippophae rhammoides (HR) and mixed-species (MX) transect. Results are means of three replicates with standard deviations (in parentheses).
In the foot and middle slope, both the SOC and TN concentrations exhibited similar patterns, highest in the MX transect, intermediate in the HR transect, and least in the AC transect. However, in the upper slope, the SOC and TN concentrations did not follow the same trajectory; the HR transect had the least SOC and TN concentrations compared with the AC and MX transects (). Moreover, for the HR and MX transects, both the TN and SOC concentrations were significantly lower in the upper slope than in the middle or foot (p<0.05) while the differences in SOC or TN concentrations between middle and foot slope were always insignificant, presenting a downhill cluster trend. The AC transect is of particular interest, where the SOC concentration was higher in the foot (2.89 g kg−1) and upper slope (2.64 g kg−1) but lower in the middle (2.30 g kg−1), demonstrating a “V” shape. In addition, the distribution of TN concentration in the AC transect was also distinct in that the TN concentration was significantly higher in the upper slope (0.38 g kg−1) than in the foot (0.33 g kg−1) (p<0.05). Interestingly, the TN contents increased from the foot to the upper section of the slope along the AC transect.
Figure 2. Soil organic carbon (SOC) (left) and total nitrogen (TN) (right) at different slope positions for Artemisia capillaris (AC), Hippophae rhammoides (HR), and mixed-species (MX) transect. Error bars denote standard deviations of means (n = 3). Different lowercase letters above vertical bars within each transect are significantly different (p<0.05).
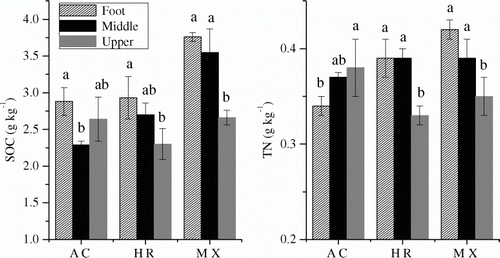
Carbon:nitrogen (C:N) ratios
As shown in , the C:N ratios were highest in the 0–5 cm soil depth for all three transects. However, the vertical distribution of the C:N ratios did not show any clear trends in the soil profile. The MX transect had higher C:N ratios than the AC and HR transects for all soil depths. The overall C:N ratios followed an order of MX (8.33) > AC (6.80) > HR (6.78). The highest C:N ratios were observed in the MX transect at three slope positions. Similar to the distribution pattern of the SOC and TN concentrations, the C:N ratios were significantly lower in the upper or middle slope compared with the foot slope (p<0.05), except for the HR transect, which exhibited no significant difference.
Table III. C:N ratios at different soil depths and slope positions for Artemisia capillaris (AC), Hippophae rhammoides (HR) and mixed-species (MX) transect. Results are means of three replicates with standard deviations (in parentheses).
Comparisons between the effects of afforestation transects with slope positions
According to the results of general linear model (GLM) (), 78.9% and 84.3% (partial eta squared, η2) of the total variance of the SOC and C:N ratios was attributed to the afforestation transects. However, for TN concentration, afforestation transects only accounted for 35.6% of the total variance, yet the effect sizes of the slope positions and the interaction between the transects and slope positions were much higher, 0.460 and 0.697, respectively.
Table IV. General linear model (GLM) results showing proportion of the variances of soil organic carbon (SOC), total nitrogen (TN) and C:N ratios explained by the afforestation transects (T) and slope positions (P). η2 (Partial eta squared) is a measure of effect size.
Discussion
Impact of afforestation modes on SOC and TN concentrations
Because of the lack of farmland slopes with the same adjacent afforestation transects in the Yangjuangou watershed, the significance test cannot be used for comparison of soil properties between single-species afforestation and multi-species afforestation mode. However, due to the scarcity of research concerned with the impact of multi-species afforestation on soil properties within the Loess Plateau, the absence of replicas should not impair the ecological importance of this study.
This study showed the SOC concentration in the MX transect was 25.8% and 27.7% higher than that in the AC and HR transects, respectively, reaching 3.32 g kg−1. This can be partially attributed to the multiplex configuration of trees, shrubs, and grass possessed by the multi-species afforestation mode, creating a higher litter input as compared with the single-species afforestation mode (Zak et al. Citation2003). In addition, under multi-species afforestation mode, the plant diversity, soil microbes and enzyme activities may be higher than the other two single-species afforestation modes, which further enhanced the soil nutrients. Sandoval-Pérez et al. (Citation2009) found that the total C, N, P, and acid phosphatase, urease, dehydrogenase contents were generally the highest in forests with the highest plant diversity. Therefore, it is possible that the higher soil microbes and enzyme activities in the MX transect boosted the transformation and cycle of soil properties, resulting in a higher overall SOC concentration.
Although the TN concentration in the multi-species afforestation mode was slightly higher than that in the single-species afforestation modes (0.40 g kg−1 vs 0.37 g kg−1 and 0.38 g kg−1), there was actually little difference in concentration. Our result was inconsistent with previous studies which suggested both the higher SOC and TN concentrations were associated with higher plant diversities (Fornara and Tilman Citation2008, Zhou et al. Citation2011). This may be due to the higher nitrogen absorption in the multi-species afforestation mode, which offsets the additional SOM produced in comparison to the single-species afforestation modes. In addition, as demonstrates, afforestation transect is the major contributor to the total variance in SOC concentration rather than TN concentration (78.9% vs 35.6%). We believe these phenomena can be attributed to the fact that even though the accumulation rate of TN is similar to that of SOC in the abandoned cropland (Murty et al. Citation2002), a longer recovery time for nitrogen may still be required compared with carbon since nitrogen has additional lost pathways, such as nitrate leaching and the release of N2O (Templer et al. Citation2005).
In HR and MX transects, the SOC and TN showed an increasing trend from the upper slope position to the foot slope position, which was in agreement with previous research (Hao et al. Citation2002, De Gryze et al. Citation2008). This was probably because of the high contents of clay in the soil at the foot slope. Fine particles (such as clay) in the top soil of the upper slope are easily carried away by runoff during erosion. Thus, the contents of clay-sized soil particles are more often higher in the foot slope (Khormali et al. Citation2009). Usually clay will bind with SOM appearing in forms of an organic-clay complex (Fullen et al. Citation2006). As a result, along with clay, the SOM tends to become enriched in foot slope. Since both the SOC and TN are derived from the SOM, the soil nutrients in the foot slope would be higher compared with the middle and upper sections.
In this study, the SOC in the AC transect was higher in the foot and upper slope but lower in the middle, demonstrating a “V” shape, while the TN increased from the foot to the upper slope. Actually, the vegetation cover is an important factor responsible for the unique distribution pattern of the SOC in the AC transect. Compared with the upper and foot slope, the vegetation in the middle was sparser. Therefore, the loss of SOC in the middle may far outweigh that in the upper slope because of water erosion, which caused higher SOC concentration in the upper slope. In addition, since the foot slope was a sink for sediment deposition, it held the highest concentration of SOC (). With regard to the distribution pattern of TN, it would be similar to SOC if the TN concentration in the foot slope were higher than the middle and upper sections. Hence, the core problem is to elucidate why the TN concentration was the lowest in the foot slope in the AC transect. More leaching and more vertical infiltration in the foot slope that caused lower TN concentrations compared with the middle and upper slopes should be the reasons, which were also mentioned by Moges and Holden (Citation2008).
In short, considering the distribution patterns of soil properties at different slope positions, multi-species afforestation mode and single-species afforestation mode did not make much difference for preventing soil carbon and nitrogen losses induced by erosion (except AC transect). However, multi-species afforestation mode can accumulate more SOC compared with single-species afforestation mode after 10 years of vegetation restoration. Therefore, from these perspectives, multi-species afforestation mode could be a better choice for the GGP and NFCP although long-term observations are still needed at this site.
Impact of afforestation modes on C:N ratios
Soil C:N ratio represents the relative proportion of the two elements in soil. In this study, given the minute difference of TN concentrations among three transects, the higher C:N ratio in multi-species afforestation mode could be attributed to the higher SOC accumulation compared with single-species afforestation mode. In addition, the C:N ratios in our research were no more than 10:1, which can be interpreted in three ways. First, this was the indication of easily decomposable SOM incorporated in the soil in the three afforestation transects, or the symbol of small unavailable carbon (Yimer et al. Citation2007, Chu and Grogan Citation2009). Second, according to Chapin (Chapin et al. Citation2002), microbes require soil substrates with C:N ratios of 25:1 in order to guarantee their nitrogen needs. Thus, there may be an excess release of nitrogen under the three afforestation transects from microbes by decomposing SOM. This theory can also partially explain why TN concentrations in the three transects were almost the same in our study. Third, the lower C:N ratios in our study indicated that a long-term study to cope with the nitrogen dynamic associated with possible botanical interferences in this area is necessary since the nitrogen in the soil has not attained steady state after only 10 years of afforestation.
The potential of the SOC and C:N ratio as indicators for evaluating the vegetation restoration programs
Both the SOC and C:N ratio have been suggested to be useful indicators in assessing soil quality by previous research. According to the studies from the high plains, loess hills and Palouse prairie in the USA, SOC has been reported to be an indicator that can provide greatest potential when monitoring soil quality (Brejda et al. Citation2000a, Citation2000b). Similarly, Rajan et al. (Citation2010) found that the SOC was the most reliable one for monitoring land quality after estimating 24 soil quality indicators. For the C:N ratio, it has been proposed as an indicator reflecting nitrate leaching (Dise et al. Citation1998, Curtis et al. Citation2004). Actually, both the decomposition of the SOM and the corresponding C and N availability are influenced by the soil C:N ratio. Since the C:N ratio is usually stable in soil (i.e. dramatic changes would not occur over short space and time scale), objective reflection on soil quality would be expected by selecting this indicator (Black et al. Citation2010).
In our study, the SOC concentration and C:N ratio in multi-species afforestation mode were about 30% and 20% higher than that in single-species afforestation mode. Hence, the soil conditions following different afforestation modes can be distinguished by the SOC and C:N ratio respectively. We believed this fact was of significant value for evaluating the vegetation restoration programs in the Loess Plateau and similar semi-arid environments. Since monitoring environment impacts (such as soil quality) is an integral part of assessing ecological restoration programs, the selection of appropriate indicators is critical (Yin et al. Citation2010). On the one hand, as aforementioned, the SOC and C:N ratio are two reliable indicators for monitoring soil quality. On the other hand, we have to admit that a combination of soil physical, chemical, and biological indicators may provide more accurate and more immediate information regarding changes in soil environment. However, just as Sparling et al. (Citation2003) has mentioned, when resources were limited, the sufficiently responsive indicators instead of the more complex soil indicators should be used. Thus, we recommended SOC and C:N ratio as indicators for evaluating vegetation restoration programs because the function of indicators should be “adequate” rather than “accurate” by the practical consideration for a long-term monitoring study.
Acknowledgements
This work was funded by the National Natural Science Foundation of China (Nos 40930528 and 40971065) and the CAS/SAFEA International Partnership Program for Creative Research Teams of “Ecosystem Processes and Services.” Our thanks go to Sarah Paine for her improvements of the English. We also thank Dr Chanjuan Hu and Ruiying Chang for their valuable suggestions.
References
- An , S. S. , Mentler , A. , Mayer , H. and Blum , W. E. H. 2010 . Soil aggregation, aggregate stability, organic carbon and nitrogen in different soil aggregate fractions under forest and shrub vegetation on the Loess Plateau, China . Catena , 81 : 226 – 233 .
- Andrén , O. , Kirchmann , H. , Kätterer , T. , Magid , J. , Paul , E. A. and Coleman , D. C. 2008 . Visions of a more precise soil biology . European Journal of Soil Science , 59 : 380 – 390 .
- Bai , Z. G. and Dent , D. 2009 . Recent land degradation and improvement in China . AMBIO: A Journal of the Human Environment , 38 : 150 – 156 .
- Bao , S. D. 2000 . Analysis method of soil agricultural chemistry , 3rd Edn , Beijing : Chinese Agricultural Press .
- Black , H. I. J. , Britton , A. , Helliwell , R. A. , Langan , S. , Taylor , A. , & Booth , P. D. 2010 . Consultancy Service: To establish soil indicators to assess the impact of atmospheric deposition on environmentally sensitive areas . Scottish Environment Protection Agency Commissioned Report .
- Brejda , J. J. , Karlen , D. L. , Smith , J. L. and Allan , D. L. 2000a . Identification of regional soil quality factors and indicators II. Northern Mississippi Loess Hills and Palouse Prairie . Soil Science Society of America Journal , 64 : 2125 – 2135 .
- Brejda , J. J. , Moorman , T. B. , Karlen , D. L. and Dao , T. H. 2000b . Identification of regional soil quality factors and indicators I. Central and Southern High Plains . Soil Science Society of America Journal , 64 : 2115 – 2124 .
- Cao , S. X. 2011 . Impact of China's large-scale ecological restoration program on the environment and society in arid and semiarid areas of China: achievements, problems, synthesis, and applications . Critical Reviews in Environmental Science and Technology , 41 : 317 – 335 .
- Chapin , F. S. , Matson , P. A. and Mooney , H. A. 2002 . Terrestrial Nutrient Cycling: Principles of Terrestrial Ecosystem Ecology , New York : Springer .
- Chen , L. D. , Wei , W. , Fu , B. J. and Lu , Y. H. 2007 . Soil and water conservation on the Loess Plateau in China: review and perspective . Progress in Physical Geography , 31 : 389 – 403 .
- Chu , H. and Grogan , P. 2009 . Soil microbial biomass, nutrient availability and nitrogen mineralization potential among vegetation-types in a low arctic tundra landscape . Plant and Soil , 329 : 411 – 420 .
- Curtis , C. J. , Emmett , B. A. , Reynolds , B. and Shilland , J. 2004 . Nitrate leaching from moorland soils: can soil C:N ratios indicate N saturation? . Water, Air, and Soil Pollution: Focus , 4 : 359 – 369 .
- De Gryze , S. , Six , J. , Bossuyt , H. , Van Oost , K. and Merckx , R. 2008 . The relationship between landform and the distribution of soil C, N and P under conventional and minimum tillage . Geoderma , 144 : 180 – 188 .
- Dise , N. B. , Matzner , E. and Forsius , M. 1998 . Evaluation of organic horizon C:N ratio as an indicator of nitrate leaching in conifer forests across Europe . Environmental Pollution , 102 : 453 – 456 .
- Dube , F. , Zagal , E. , Stolpe , N. and Espinosa , M. 2009 . The influence of land-use change on the organic carbon distribution and microbial respiration in a volcanic soil of the Chilean Patagonia . Forest Ecology and Management , 257 : 1695 – 1704 .
- Fornara , D. A. and Tilman , D. 2008 . Plant functional composition influences rates of soil carbon and nitrogen accumulation . Journal of Ecology , 96 : 314 – 322 .
- Fu , B. J. and Chen , L. D. 2000 . Agricultural landscape spatial pattern analysis in the semi-arid hill area of the Loess Plateau, China . Journal of Arid Environments , 44 : 291 – 303 .
- Fu , B. J. , Chen , L. D. , Ma , K. M. , Zhou , H. F. and Wang , J. 2000 . The relationships between land use and soil conditions in the hilly area of the loess plateau in northern Shaanxi, China . Catena , 39 : 69 – 78 .
- Fu , B. J. and Gulinck , H. 1994 . Land evaluation in an area of severe erosion: The Loess Plateau of China . Land Degradation and Development , 5 : 33 – 40 .
- Fu , B.-J. , Wang , Y.-F. , Lu , Y.-H. , He , C.-S. , Chen , L.-D. and Song , C.-J. 2009 . The effects of land-use combinations on soil erosion: a case study in the Loess Plateau of China . Progress in Physical Geography , 33 : 793 – 804 .
- Fu , X. L. , Shao , M. A. , Wei , X. R. and Horton , R. 2010 . Soil organic carbon and total nitrogen as affected by vegetation types in Northern Loess Plateau of China . Geoderma , 155 : 31 – 35 .
- Fullen , M. A. , Booth , C. A. and Brandsma , R. T. 2006 . Long-term effects of grass ley set-aside on erosion rates and soil organic matter on sandy soils in east Shropshire, UK . Soil and Tillage Research , 89 : 122 – 128 .
- Gong , J. , Chen , L. , Fu , B. , Huang , Y. , Huang , Z. and Peng , H. 2006 . Effect of land use on soil nutrients in the loess hilly area of the Loess Plateau, China . Land Degradation and Development , 17 : 453 – 465 .
- Han , X. W. , Tsunekawa , A. , Tsubo , M. and Li , S. Q. 2009 . Effects of land-cover type and topography on soil organic carbon storage on Northern Loess Plateau, China . Acta Agriculturae Scandinavica, Section B – Soil & Plant Science , 60 : 326 – 334 .
- Hao , Y. , Lal , R. , Owens , L. B. , Izaurralde , R. C. , Post , W. M. and Hothem , D. L. 2002 . Effect of cropland management and slope position on soil organic carbon pool at the North Appalachian Experimental Watersheds . Soil and Tillage Research , 68 : 133 – 142 .
- Jiao , F. , Wen , Z.-M. and An , S.-S. 2011 . Changes in soil properties across a chronosequence of vegetation restoration on the Loess Plateau of China . Catena , 86 : 110 – 116 .
- Khormali , F. , Ajami , M. , Ayoubi , S. , Srinivasarao , C. and Wani , S. P. 2009 . Role of deforestation and hillslope position on soil quality attributes of loess-derived soils in Golestan province, Iran . Agriculture, Ecosystems and Environment , 134 : 178 – 189 .
- Kucharik , C. J. and Jelinski , N. A. 2009 . Land-use effects on soil carbon and nitrogen on a US Midwestern Floodplain . Soil Science Society of America Journal , 73 : 217 – 225 .
- Liu , J. G. and Diamond , J. 2005 . China's environment in a globalizing world . Nature , 435 : 1179 – 1186 .
- Liu , X. , Li , F.-M. , Liu , D.-Q. and Sun , G.-J. 2010 . Soil organic carbon, carbon fractions and nutrients as affected by land use in semi-arid region of Loess Plateau of China . Pedosphere , 20 : 146 – 152 .
- Moges , A. and Holden , N. 2008 . Soil fertility in relation to slope position and agricultural land use: A case study of Umbulo Catchment in Southern Ethiopia . Environmental Management , 42 : 753 – 763 .
- Murty , D. , Kirschbaum , M. U. F. , McMurtrie , R. E. and McGilvray , H. 2002 . Does conversion of forest to agricultural land change soil carbon and nitrogen? A review of the literature . Global Change Biology , 8 : 105 – 123 .
- Rajan , K. , Natarajan , A. , Anil Kumar , K. S. , Badrinath , M. S. and Gowda , R. C. 2010 . Soil organic carbon – the most reliable indicator for monitoring land degradation by soil erosion . Current Science , 99 : 823 – 827 .
- Sandoval-Pérez , A. L. , Gavito , M. E. , García-Oliva , F. and Jaramillo , V. J. 2009 . Carbon, nitrogen, phosphorus and enzymatic activity under different land uses in a tropical, dry ecosystem . Soil Use and Management , 25 : 419 – 426 .
- Sparling , G. , Ross , D. , Trustrum , N. , Arnold , G. , West , A. , Speir , T. and Schipper , L. 2003 . Recovery of topsoil characteristics after landslip erosion in dry hill country of New Zealand, and a test of the space-for-time hypothesis . Soil Biology and Biochemistry , 35 : 1575 – 1586 .
- Templer , P. H. , Groffman , P. M. , Flecker , A. S. and Power , A. G. 2005 . Land use change and soil nutrient transformations in the Los Haitises region of the Dominican Republic . Soil Biology and Biochemistry , 37 : 215 – 225 .
- Wang , Y. F. , Fu , B. J. , Lü , Y. H. and Chen , L. D. 2011 . Effects of vegetation restoration on soil organic carbon sequestration at multiple scales in semi-arid Loess Plateau, China . Catena , 85 : 58 – 66 .
- Wei , X. R. , Shao , M. A. , Fu , X. L. , Horton , R. , Li , Y. and Zhang , X. C. 2009 . Distribution of soil organic C, N and P in three adjacent land use patterns in the northern Loess Plateau, China . Biogeochemistry , 96 : 149 – 162 .
- Wu , T. Y. , Schoenau , J. J. , Li , F. M. , Qian , P. Y. , Malhi , S. S. and Shi , Y. C. 2005 . Influence of fertilization and organic amendments on organic-carbon fractions in Heilu soil on the Loess Plateau of China . Journal of Plant Nutrition and Soil Science , 168 : 100 – 107 .
- Yimer , F. , Ledin , S. and Abdelkadir , A. 2007 . Changes in soil organic carbon and total nitrogen contents in three adjacent land use types in the Bale Mountains, south-eastern highlands of Ethiopia . Forest Ecology and Management , 242 : 337 – 342 .
- Yin , R. S. and Yin , G. P. 2009 . “ China's ecological restoration programs: Initiation, implementation, and challenges ” . In An integrated assessment of China's ecological restoration programs , Edited by: Yin , R. S. 1 – 19 . Berlin : Springer .
- Yin , R. S. , Yin , G. P. and Li , L. Y. 2010 . Assessing China's ecological restoration programs: What's been done and what remains to be done? . Environment Management , 45 : 442 – 453 .
- Zak , D. R. , Holmes , W. E. , White , D. C. , Peacock , A. D. and Tilman , D. 2003 . Plant diversity, soil microbial communities, and ecosystem function: Are there any links? . Ecology , 84 : 2042 – 2050 .
- Zhang , C. , Xue , S. , Liu , G.-B. and Song , Z.-L. 2011 . A comparison of soil qualities of different revegetation types in the Loess Plateau, China . Plant and Soil , 347 : 163 – 178 .
- Zhang , J. T. and Chen , T. G. 2007 . Effects of mixed Hippophae rhamnoides on community and soil in planted forests in the Eastern Loess Plateau, China . Ecological Engineering , 31 : 115 – 121 .
- Zhou , Z.-Y. , Li , F.-R. , Chen , S.-K. , Zhang , H.-R. and Li , G. 2011 . Dynamics of vegetation and soil carbon and nitrogen accumulation over 26 years under controlled grazing in a desert shrubland . Plant and Soil , 341 : 257 – 268 .