Abstract
This study examined whether the N release pattern during decomposition of plant materials used as green manures can be modified in the short term through mixing plant materials with different levels of easily degradable compounds. In a lysimeter study, white clover shoots were mixed with perennial ryegrass shoots or chicory roots. Leaching of clover N was decreased more by inclusion of chicory than by ryegrass. However, decreased leaching losses over the non-growing season did not lead to enhanced N uptake by the crop during the following growing season.
Introduction
Nitrogen (N) losses through leaching from agricultural fields, especially during the non-growing season, contribute to eutrophication of water bodies in northern Europe and therefore measures to counteract these N losses have been promoted in recent decades. During the same period, the demand for organically produced crops has increased. This has created a need to find environmentally sound and economically feasible methods to meet crop N requirements not only on organic mixed livestock/arable farms but also on farms without livestock. Legume-rich green manures have the potential to bind large quantities of atmospheric N, which can subsequently be made available to the following crops. However, there is also a risk of N losses through leaching if N release from the green manure is not synchronized with the N requirements of the following crop (Wivstad et al. Citation2005 and references therein). This is a particular concern in northern Europe, where there is a precipitation surplus during the non-growing season.
Plant chemical composition is key to decomposition rate and N release pattern; in the short term (e.g. within weeks after incorporation into the soil), the amounts of easily degradable compounds such as simple carbohydrates, pectins, fructans and proteins will have a large effect on the net N release, whereas the amounts of hemicellulose and cellulose will affect the net N release or immobilization in the longer term. Simultaneous application of additional C sources of high degradability (e.g. certain organic waste products) has been tested as a means of immobilizing N released during decomposition of N-rich crop residues (De Neve et al. Citation2004, Chaves Daguilar et al. Citation2007, Citation2008). Different green manure species and green manures of differing maturity are also known to be decomposed at different rates (Wivstad Citation1997). An alternative approach to addition of waste products may thus be to utilize the composition of the green manure ley itself to modify the N release pattern (Gunnarsson and Marstorp Citation2002), so that losses to the external environment can be decreased. This could be achieved by growing two or more species in mixed stands if a single species which has the desired chemical composition is not found.
The objective of this study was to determine on a meso-scale the N release from three green manure crops of differing composition and its use by a following crop. In outdoor lysimeters, we studied the effect of mixing white clover (Trifolium repens L.) shoots with roots of chicory (Chicorium intybus L.) on N losses during one year following plant material incorporation and on N uptake by grass during the following growing season. For comparison we used a mixture of perennial ryegrass (Lolium perenne L.) and white clover. We specifically tested the hypotheses:
1. | The N release pattern from clover green manure can be modified by mixing with crops rich in easily degradable carbohydrates, such as grass or chicory. | ||||
2. | The N leaching losses over winter can be decreased by use of such mixtures compared with pure clover. | ||||
3. | N uptake during the following growing season decreases as a result of the N immobilization induced. |
To this end and in order to control the total amount and composition of the green manure input we produced biomass of the three plant species separately. This was subsequently applied as equivalent biomass of pure clover or mixed clover + chicory/grass to the different treatments. This meant that N application rates varied between treatments and years. As weather conditions also varied widely between the two years, these experiments can be regarded as two case studies illustrating possible outcomes of green manure application.
Materials and methods
The two experiments were fully randomized and carried out in a lysimeter station where 15N-labelled clover shoots were added to soil, either as pure material or in mixture with ryegrass shoots or chicory roots. Leaching losses and plant utilization of added 15N and total N (totN) in excess of the control level (no plant material added) were determined during one year from addition in either 2001 or 2002.
Plant material
The plant material to be incorporated into soil during the experiment was grown from seed in Mitscherlich pots (Ø 0.25 m) in a roofed outdoor area during summer 2001 and 2002. All pots were fertilized with P and K (1.5 g P and 1.0 g K pot−1). In addition, ryegrass and chicory were fertilized with 0.4 g unlabelled N pot−1. White clover was labelled through weekly additions of 2.5 atom% 15N (15NH4NO3), giving in total 0.77 g (2001) and 0.48 g (2002) totN pot−1. The N concentration of the clover produced in this way differed between the years (). The glucose + fructose content of the clover, ryegrass, and chicory was c. 1.4, 2.0, and 2.4%, respectively, and the content of sucrose + fructan 2.6, 8.4, and 70.6%, respectively.
Table I. Total N (totN) concentration, degree of 15N-labelling (atom% 15N excess), and C concentration of plant material incorporated (air-dry material).
The plant material was harvested when still in the vegetative stage (13 June 2001 and 5 July 2002). Clover leaf discs and stalks were weighed separately when preparing plant material for incorporation into soil. Clover leaf discs were kept intact, whereas clover leaf stalks and ryegrass were cut to c. 2 cm length using a paper guillotine and chicory roots sliced to c. 1 mm discs using a food processor. All plant material was dried at 55 °C in a forced-draught oven and stored dry until incorporation.
Lysimeters
The lysimeters were made from PVC tubing (Ø 0.295 m, 0.85 m length), fitted at the base with double layers of high-density polyethylene filter with an intermediate layer of washed quartz sand. A leachate outlet was also attached at the base. The lysimeters were filled with 60 kg sandy soil (Krusenberg [59° 44′ N, 17° 40′ E], 12.4% clay, 11.0% silt, 76.5% sand, 0.1% fine gravel, 1.1% C, 0.11% N, pHH2O 5.5) sieved to 4 mm, watered and allowed to set to a bulk density of 1.3 kg L−1 soil (average for the column). The lysimeters were inserted into a station having a subterranean vault for sampling of leachate. The upper 10 cm of the gaps between the lysimeter walls and the surrounding soil (lined with slightly larger Ø PVC tubes) were filled with insulation, and subsequently covered with plastic collars.
Treatments
Treatments included pure white clover (Trifolium repens L., cv. Ramona) (treatment W), white clover + perennial ryegrass (Lolium perenne L., cv. Leia) (treatment WG), white clover + chicory (Chicorium intybus L., cv. Grasslands Puna) (treatment WC) and an unamended control (U). The plant material added corresponded to 57.3 g lysimeter−1 (or 8.5 tons dw ha−1, 50/50 clover/ryegrass or chicory in the mixtures), giving approximately equivalent amounts (23.3 g C lysimeter−1) of C through all additions. The plant material was incorporated on 1 November (2001) and 31 October (2002) by excavating the soil to 10 cm depth and mixing it with the plant material, as homogeneously as possible considering the large pieces of plant material, before it was returned to the lysimeters.
Meteorological conditions
The weather differed between the years, in particular between the two winter seasons, where the first (2001–2002) was mild and wet and the second (2002–2003) was colder and drier (). These differences resulted in the soil remaining unfrozen for most of the first winter, whereas it froze directly after green manure incorporation and stayed frozen for almost 5 months during the second winter. In addition, accumulated drainage amount was higher (256 mm) during the first winter and spring than during the second (164 mm). Both growing seasons were characterized by dry spells in early summer and moister conditions later in the growing season.
Sampling/measurements
The temperature at 5-cm soil depth was recorded weekly, except when the lysimeters were covered with snow. Soil moisture was recorded weekly from spring, using gypsum probes that were inserted at 5-cm soil depth before sowing in spring. Leachate was collected as it appeared, with a maximum delay of 7 days from start of a flush. Leachate samples were stored at −20 °C until analysed. Plant-available N was estimated through N uptake by hybrid ryegrass (Lolium×hybridum cv Spark). This was sown at a seed rate of 0.67 g seeds lysimeter−1 on 3 May (2002) and 7 June (2003) and cut five times during the season. The soil was sampled with an auger to 0–10, 10–30 and 30–80-cm depth when the experiments were terminated, c. one year after the respective green manure addition.
Analyses
Total N in leachate was determined on a Mitsubishi TN-05 (Japan). Isotope analysis of leachate N was carried out using an automatic elemental analyser (EA 1110) coupled to a Finnigan MAT Delta Plus isotope ratio mass spectrometer (Thermo Finnigan, Bremen, Germany) after oxidation of organic N and subsequent microdiffusion (Herrmann et al. Citation2007).
Ball-milled plant materials and soils were analysed for total N and C on a Leco CNS-2000 analyser and for N isotope composition by mass spectrometry (see above). The individual plant species of the green manures were also analysed for glucose + fructose and sucrose + fructans (Larsson and Bengtsson Citation1983).
Calculations
Plant uptake and leaching of incorporated clover N and clover N remaining in the soil were estimated using an equation adapted from Barraclough (Citation1995):
where: Ndfclover = N derived from clover
A = totN in crop/leachate/soil
C = atom% 15N excess of crop/leachate/soil
D= atom% 15N excess of incorporated clover
and atom% 15N excess denotes the enrichment of N in each pool relative to that in air.
Statistics
Data were analysed statistically by ANOVA using the JMP 8.0.1 (SAS Institute Inc.) least squares fit procedure and then by Student's t-test whenever the ANOVA indicated significant differences (p<0.05).
Results
Leaching of N
The accumulated leaching losses of totN ranged from 1.58 to 2.45 g lysimeter−1 during 2001–2002, but were only around 10% of that amount during 2002–2003 (a, b). During 2001–2002, the leaching losses of totN were significantly different between all treatments (p<0.001), being highest in the W treatment, followed by WG, WC and U. During 2002–2003, leaching losses of totN (p=0.0167) were significantly higher in the W and the WG treatments than in WC, while leaching losses of totN from U were intermediate and not significantly different from the other treatments.
Figure 2. Leaching and plant uptake of total N (totN) and clover-derived N (mg lysimeter−1) after incorporation of pure white clover (W, diamonds), mixed white clover–perennial ryegrass (WG, squares) and mixed white clover–chicory (WC, triangles) into soil on (a) 1 November 2001 and (b) 31 October 2002. Closed symbols indicate totN and open symbols clover-derived N. Leaching and plant uptake of totN in the unamended control (U, cross). Error bars indicate standard error of the mean (n = 4). Note the different scales on the vertical axes.
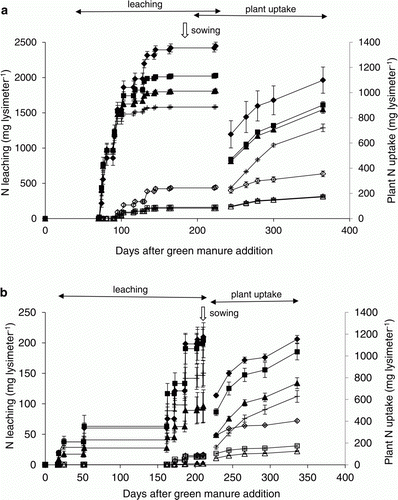
Total leaching of clover-derived N during 2001–2002 was higher in treatment W than in the two mixed treatments (p<0.001), which did not differ significantly from one another. During 2002–2003 leaching losses of clover-derived N (p=0.001) were significantly higher in the W and WG treatments than in WC.
The percentage of clover-derived N lost through leaching followed the same pattern as the total amount leached in 2001–2002, i.e. it was significantly (p=0.0002) higher in the W treatment (17.2%) than in WG (12.6%) and WC (11.6%) (), while it was highest in the WG treatment (1.6%), followed by W (1.0%) and WC (0.2%) (p=0.0026) in 2002–2003.
Figure 3. Fate of clover N after incorporation into soil of green manures consisting of pure white clover (W), mixed white clover–perennial ryegrass (WG) or mixed white clover–chicory (WC). Percentage of clover-derived N recovered in leachate (light grey), in shoots of the following crop (black) and remaining in soil after one year (dark grey).
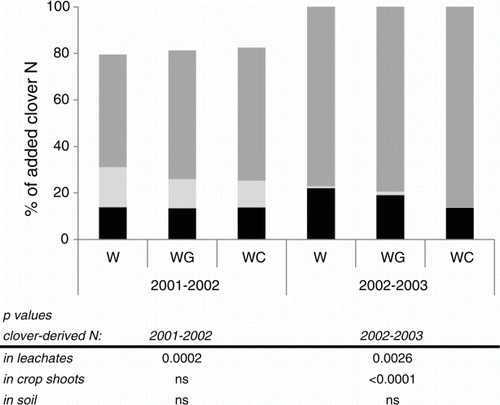
Plant growth and N uptake
Plant growth was restricted by dry weather especially during August 2002 and July 2003, which also reflected on the N uptake. The accumulated ryegrass production in 2002, expressed as g lysimeter−1, was significantly (p=0.0002) higher in the W treatment (82.2 g) than in WC (71.1 g) which was in turn significantly higher than in U (56.2 g), whereas WG (74.2 g) was not significantly different from W or WC. In 2003 all treatments differed significantly (p<0.001) in the order W (63.5 g) > WG (58.2 g) > WC (48.7 g) > U (41.5 g).
Accumulated plant uptake of totN (a) was significantly higher in the W treatment (p=0.005) than in WG, WC and U in 2002, but no significant differences were observed between the latter. In 2003 all treatments differed significantly (p<0.001) in the order W > WG > WC > U.
The amounts of clover-derived N taken up by plants were highest in the W treatment in both years (p<0.001) (a, b). In 2002, there was no difference in plant uptake of clover N between the WG and WC treatments, but in 2003 the uptake was lower in WC than in WG or W.
Plant uptake of clover-derived N was 13–14% for all treatments in 2002 (). During 2003 it was highest in W (22.5%), followed by WG (19.5%) and lowest in WC (13.9%) (p<0.001).
Clover N in the soil
Clover-derived N present in the soil at the end of the growing season in the year following green manure application was higher in the W treatment (1241 and 1449 mg lysimeter−1 in 2002 and 2003, respectively) than in the mixed treatments (average 721 and 779 mg lysimeter−1 in 2002 and 2003, respectively; p<0.001 both years). This corresponded to an average of 54% (2002) and 89% (2003) of the added clover N ().
Missing N
In October 2002, one year after green manure incorporation, an average of 18.9% of the applied clover N had not been recovered in drainage water, plants or soil, with no significant differences between the treatments (). In October 2003, one year after the second green manure incorporation, there were also no significant differences between treatments. However, in that year there was full recovery of added clover N.
Discussion
Previous studies have shown that turnover of plant materials in soil occurs at rates and with patterns determined by, amongst other factors, the composition of the material in question and thus the compounds that make up the biomass (e.g. Marstorp Citation1996, Gunnarsson and Marstorp Citation2002, Gunnarsson et al. Citation2008). Those studies stress the importance of the chemical composition of plant materials, apart from C:N ratio or N concentration, for the N mineralization pattern in the short term. It has been suggested that a way to reduce leaching is to add additional C sources with high C availability (e.g. certain organic waste products) to the N-rich crop residues as a means of immobilizing N (De Neve et al. Citation2004, Chaves Daguilar et al. Citation2007, Citation2008). In our study, plant materials rich in easily degradable carbohydrates were mixed with clover to test the potential usefulness of such companion crops in mixed stands in the field as an alternative to applying external organic waste products.
The long autumn and mild winter of 2001–2002, with high drainage combined with an N-rich clover in 2001, led to very large leaching losses of clover N. However, the proportion of clover N lost was considerably lower with the clover–grass mixture (average 27% lower) and clover–chicory mixture (average 33% lower). During the following winter, when the weather conditions were fundamentally different, the proportion of clover N leached was also significantly lower with the clover–chicory mixture than with the pure clover. This indicates that the release pattern of legume N and the subsequent susceptibility of N released to leaching at ambient weather conditions can be modified by inclusion of companion crops rich in easily degradable carbohydrates in green manure leys.
In the experimental setup chosen, the pure clover treatment inevitably received more N through green manure application than the clover–grass and clover–chicory treatments. However, the latter two treatments, which had approximately the same amount of applied total N, differed in total leaching losses during both years. This illustrates that leaching losses from a legume compartment may indeed be reduced by companion crops and that the chemical composition of such companion crops affects their efficiency in counteracting leaching losses.
Approximately 20% of the incorporated N was not recovered in plants, soil or leaching after the first year of the experiment, indicating that gaseous losses most likely occurred. The conditions after green manure incorporation in that year were conducive to denitrification, with wet and relatively warm soil and a combination of labile N and easily available C sources, all preconditions for efficient denitrification. However, the next year was characterized by full recovery of clover N, suggesting minimal losses.
In 2002, autumn was followed by an early winter, with below-zero temperatures immediately after incorporation of the green manures and early freezing of the soil profile, which then remained frozen until the second week of April 2003. Hence, water drainage and turnover of the incorporated green manures were very low and, as discussed above, N leaching losses subsequently minimal. High crop uptake of N could therefore have been anticipated during the subsequent growing season in treatments where pure clover had been added, as was indeed observed in our study. However, where a mix of clover and chicory had been added the plant uptake of clover-derived N was as low as in the preceding year. This was most likely caused by delayed turnover of the green manure until spring as a result of the early winter, leading to N immobilization during chicory turnover in spring. This shows that plant N uptake can even be decreased despite reduced leaching, if the weather conditions are colder than expected, causing immobilization to occur in spring rather than in autumn. This confirms previous findings that whilst immobilization of N mineralized from crop residues is relatively easy to achieve in the field by addition of organic waste products, the success in promoting N remineralization in the following spring is variable (De Neve et al. 2004, Chaves Daguilar et al. 2007, 2008). It also emphasizes the fundamental impact of the weather on net N release from organic materials/green manures, and the subsequent potential for N losses and N use. As soil temperature and moisture cannot be governed in the field situation, the success of steering N release inevitably varies over time.
Acknowledgements
We gratefully acknowledge the research programme FOOD21, which provided the funding for the project. We also thank SLU laboratory staff for technical assistance.
References
- Barraclough , D. 1995 . 15N isotope dilution techniques to study soil nitrogen transformations and plant uptake . Fertility Research , 42 : 185 – 192 .
- Chaves Daguilar , B. , De Neve , S. , Boeckx , P. , Van Cleemput , O. , & Hofman , G. 2007 . Manipulating nitrogen release from nitrogen- rich crop residues using organic wastes under field conditions . Soil Science Society of America Journal 71 , 1240 – 1250 . doi: 10.2136/sssaj2005.0379
- Chaves Daguilar , B. , De Neve , S. , Boeckx , P. , Dupont , R. , Van Cleemput , O. and Hofman , G. 2008 . Manipulating the N release from 15N-labelled celery residues by using straw and vinasses in Flanders (Belgium). Agriculture . Ecosystems and Environment , 123 : 151 – 160 .
- De Neve , S. , Gaona Saéz , S. , Chaves Daguilar , B. , Sleutel , S. and Hofman , G. 2004 . Manipulating N mineralization from high N crop residues using on- and off-farm organic materials . Soil Biology and Biochemistry , 36 : 127 – 134 .
- Gunnarsson , S. and Marstorp , H. 2002 . Carbohydrate composition of plant materials determines N mineralization . Nutrient Cycling in Agroecosystems , 62 : 175 – 183 .
- Gunnarsson , S. , Marstorp , H. , Dahlin , A. S. , & Witter , E. 2008 . Influence of non-cellulose structural carbohydrate composition on plant material decomposition in soil . Biology and Fertility of Soils 45 , 27 – 36 . doi: 10.1007/s00374-008-0303-5 .
- Herrmann , A. M. , Witter , E. and Kätterer , T. 2007 . Use of acetylene as a nitrification inhibitor to reduce biases in gross N transformation rates in a soil showing rapid disappearance of added ammonium . Soil Biology and Biochemistry , 39 : 2390 – 2400 .
- Larsson , K. & Bengtsson , S. 1983 . Bestämning av lätt tillgängliga kolhydrater i växtmaterial . Statens Lantbrukskemiska Laboratorium, method 22. (In Swedish) .
- Marstorp , H. 1996 . Influence of soluble carbohydrates, free amino acids, and protein content on the decomposition of Lolium multiflorum shoots . Biology and Fertility of Soils , 21 : 257 – 263 .
- Wivstad , M. 1997 . Nitrogen mineralization and crop uptake of N from decomposing 15N labelled red clover and yellow sweet clover plant fractions of different age . Plant and Soil , 208 : 21 – 31 .
- Wivstad , M. , Dahlin , A. S. and Grant , C. 2005 . Perspectives on nutrient management in arable farming systems . Soil Use and Management , 21 : 113 – 121 .