Abstract
The effect of four nitrogen (N) availabilities on growth, leaf N concentration, N productivity (dry matter production per unit time and unit N taken up), shoot:root ratio and carbohydrate storage was studied in velvet bentgrass (Agrostis canina ‘Legendary’), creeping bentgrass (A. stolonifera ‘Independence’), slender creeping red fescue (Festuca rubra ssp. trichophylla ‘Cezanne’) and chewings fescue (F. rubra ssp. commutata ‘Center’). In growth chamber experiments, plants were grown for 3 weeks in sand with 12.5, 25, 50 or 200 mg N L−1 in the irrigation water and at two mowing intensities, cut at 5 mm twice per week or uncut. It proved possible to control important turf grass traits such as shoot growth rate, shoot:root ratio, leaf morphology and carbohydrate storage through leaf N concentration. The relationship between leaf N concentration and aboveground growth was linear for both cut and uncut turf. The relative N demand of the studied species, based on their N productivity, was 1:0.67:0.67:0.37 for creeping bentgrass, velvet bentgrass, chewings fescue and slender creeping red fescue, respectively. Clipping significantly reduced N productivity, and hence turf N demand. The lowest possible leaf N concentration without adverse effects on plant health and appearance was between 3.1 and 3.5% of dry matter (DM) in both bentgrasses and fescues. This value can be used as a target in minimizing fertilizer usage and N leaching losses. In conclusion, fertilization based on the influence of leaf N concentration on growth-related processes offers possibilities to control growth in a predictable and desirable manner under varying climate and growth conditions. This could provide more environmentally friendly and economic fertilization regimes and also better playing quality.
Abbreviations
Bve=Velvet bentgrass | = |
Bcr=Creeping bentgrass, DM=dry matter |
Fcr=Slender creeping red fescue | = |
Fch=Chewings fescue, LSD=least significant difference, N1, N2, N4, N16=fertilization regimes with 12.5, 25, 50 and 200 mg N L−1, respectively |
SLA=Specific Leaf Area | = |
m2 kg−1 leaf mass. |
Introduction
Timing and application rate of fertilizers should be managed throughout the growing season in order to achieve high turf quality without adverse effects on the environment. Scrutiny of the literature reveals major discrepancies between studies with regard to the range of N and other plant nutrients tolerated by commonly used high maintenance turf grasses (Jones Citation1980, Smith et al. Citation1985, Mills and Jones Citation1996). However, the relationship between plant N concentration and growth has been studied extensively in other plant species (e.g. Ingestad and Lund Citation1986, Ingestad and Ågren Citation1995, Glimskär and Ericsson Citation1999). Growth, biomass allocation and carbohydrate storage in plant tissues are reported to be linearly related to plant N concentration (McDonald et al. Citation1986, Ericsson Citation1995, Ericsson et al. Citation1996). The optimum leaf N concentration, i.e. the lowest possible N concentration that allows growth at a maximum rate, is a unique species character (Ingestad Citation1991). This also holds true for the minimum leaf N concentration that prevents negative impacts on plant health and appearance. The slope of the linear relationship between N concentration and growth rate, i.e. N productivity (dry matter production per unit time and unit N in plant; Ågren and Ingestad Citation1987), is also species-specific and positively correlated to the genotypic growth capacity of the plant species. Nitrogen productivity is influenced by climate and decreases when the availability of, for example, light, temperature, water and nutrients becomes less favourable for growth, and when turf growth is hampered, for example by lower clipping height, intensive playing activity or other stress factors (Ingestad and Lund Citation1986). Both leaf size and relative shoot growth are positively correlated with shoot N concentration, while leaf thickness (specific leaf area, SLA, m2 kg−1 leaf mass) and thereby leaf stiffness and relative root growth increase in response to N shortage (McDonald et al. Citation1992). Through a negative correlation between N concentration and carbohydrate storage, shoot N concentration can play a role for plant vigour (McDonald et al. Citation1986). A positive relationship between plant carbohydrate concentration and levels of defensive chemicals and resistance to insect attacks has been demonstrated in young willow plants (Waring et al. Citation1985, Larsson et al. Citation1986).
The influence of shoot N concentration on growth-related processes in plants seems to be of a general nature during the vegetative growth stage. For example Glimskär and Ericsson (Citation1999) studied growth strategies in forbs and grasses in response to N availability and found that the relationship between plant N concentration and both growth and specific leaf area followed the same patterns as demonstrated for woody plant species (Ingestad and Ågren Citation1988, McDonald et al. Citation1992, Ericsson et al. Citation1996). If the observed correlations between tissue N concentration and various growth and plant composition characteristics also apply for short-clipped turf grass, this could constitute an efficient tool for planning fertilization management practices not only for adequate growth, but also for quality aspects such as a vigorous root system, enhanced ball roll through leaf stiffness and greater stress resistance.
The relationship between leaf N concentration and the traits mentioned above has to our knowledge not been systematically studied in turf grass species and for turf influenced by mowing. The information presented by Ingestad and co-workers has been used in Sweden in pilot field experiments with creeping bentgrass (Agrostis stolonifera) (Ericsson Citation2005). The results to date are promising, but improved knowledge is needed for several species in order to improve fertilizer use efficiency by reducing the current use of plant nutrients while promoting turf vigour by supporting a desirable shoot:root ratio and keeping a high carbohydrate storage in the tissues.
This paper reports the first of two studies performed under laboratory and field conditions, respectively, the overall aim of which was to develop recommendations for demand-driven fertilization of turf based on the theories developed by Ingestad and co-workers describing the influence of leaf N concentration on different plant growth characteristics. The study described here tested the hypothesis that the relationship between leaf N concentration and growth rate is also linear for frequently clipped turf and sought to determine species-specific N productivity values under optimum climate conditions and use these for ranking turf grass species with regard to N demand. The effect of different leaf N concentrations on biomass allocation between roots and shoots and fructan storage and the influence of cutting were also evaluated. Four commonly used cold temperate turf grass species (two fescues and two bentgrasses) were used in the study.
Materials and methods
Plant material and growth conditions
The effects of N availability on growth, leaf N concentration and carbon (C) allocation between root and shoot were studied in velvet bentgrass (Bve; A. canina L., var. ‘Legendary’), creeping bentgrass (Bcr; Agrostis stolonifera L., var. ‘Independence’), slender creeping red fescue (Fcr; Festuca rubra ssp. trichophylla L., var. ‘Cezanne’), and chewings fescue (Fch; F. rubra ssp. commutata L., var. ‘Center’). The experiment was carried out in a growth chamber with a photoperiod of 16/8 h d/n, light intensity 300 µmol m−2s−1 (OSRAM HQI-E/D 250W), air temperature 20/15 °C d/n and relative air humidity 70%.
Turf establishment
The grasses were grown in 0.3-L plastic pots with a surface area of 44 cm2. Washed sand with a particle size of 1–2 mm was used as growth substrate. The small seeds of the bentgrasses (25 mg pot−1) were placed on the sand surface, while the larger fescue seeds (70 mg pot−1) were covered by a 2 mm thick sand layer. The pots were placed on trays and the substrate was wetted with pure water through sub-surface irrigation, i.e. water was sucked up by capillary forces in the soil. The trays were covered by a transparent plastic sheet during germination. The seedlings received free access to all nutrients in order to rapidly develop a strong turf. This was achieved by twice-weekly addition of a complete, balanced nutrient solution () at a concentration of 200 mg N L−1. The establishment phase lasted for 4 weeks. During this period the grass was cut twice per week and the cutting height was gradually decreased from 20 mm to 5 mm.
Table I. Amounts of elements in the nutrient solution used in experiments (Wallco 51-10-43 + microa).
Treatments
Four levels of N availability were created after the establishment phase through subsurface irrigation as described in the above paragraph twice a week with water containing 12.5, 25, 50 or 200 mg N L−1 (treatments N1, N2, N4 and N16, respectively). Excess water on the trays was removed after approximately 10 minutes. The same balanced nutrient solution as in the establishment phase was used, and hence all nutrients were added in proportion to N. For each N regime, 12 pots per species were used. The following 3 weeks were regarded as an acclimation phase to the different N regimes. During this period, growth rate, carbon allocation and N concentration in the tissues adjusted to the rate of N supply. The turf was cut twice a week at 5 mm height during and at the end of the acclimation phase. During the following 3 weeks, clippings were collected from the cut treatment twice per week from 6 pots per fertilization treatment and species, dried (48 h at 70 °C), pooled and weighed to determine the biomass production. The turf in the remaining pots was allowed to grow undisturbed. For the uncut treatment, all leaf parts 5 mm above the soil surface were removed 3 weeks after the acclimation phase, dried (48 h at 70 °C) and weighed. The root systems in all pots were washed free from sand and root dry mass, including basal leaf parts, was determined after 3 days drying at 70 °C.
Chemical analysis
The N content in pooled clippings (cut treatment; n=6) and whole leaves (uncut treatment; n=4) was determined with an elemental analyser (CN2000, LECO Corporation, USA).
Non-structural carbohydrates were determined in pooled and milled leaf samples from Bcr and Fcr using the method described by Steen and Larsson (Citation1986), where a two-step extraction procedure was applied. Glucose, fructose and sucrose were determined in the first extract and residual fructans in the second extract.
Statistical analysis
The general linear model procedure (GLM) of the statistical analysis system (SAS) was used to analyse the variance (Version 9.2, SAS Institute, Cary, NC). The significance of differences between the different N fertilization intensities and species was estimated using the Tukey test and probabilities equal to or less than 0.05 were considered significant. Assumptions of normal distribution and variance homogeneity were tested graphically using residual plots. Regressions between leaf N concentration and leaf biomass were done using SigmaPlot (Version 12.0, Systat Software Inc., San Jose, CA).
Results
Turf appearance
Visual observations showed that all species grew vigorously and developed dark-green leaves during the establishment phase when grown under free access to all nutrients (N16). Deficiency symptoms, in the form of chloroses and necroses, developed in older leaves both during and after the phase of growth acclimation as a result of growth-limiting N conditions. These symptoms became pronounced in the bentgrasses at N2 and in both fescues at N1. Leaf length and width in all species studied were reduced at N1–N4 compared with N16 and roots became thinner and branching more intense in response to N shortage (visual observations).
Biomass production
As averages of the fertilization regimes, uncut plants produced 4.5 (Bve) to 5.8 (Fcr) times more leaf biomass during the experimental period than plants cut twice a week, while root biomass was less influenced by cutting and resulted on average in 1.1 (Bve) to 1.5 (Fcr) times higher root biomass for the uncut treatment (a and b). The different species reacted to different extents to increased N fertilization. For the cut treatment, the bentgrasses showed a strong increase in leaf biomass with increasing N fertilization, while root biomass decreased at the highest N level. The leaf biomass of the cut fescues also increased with increased N fertilization, but to a lower extent. However, root biomass of the cut fescues remained unchanged or even increased at increasing N fertilization rates. Leaf biomass of uncut treatments also reacted strongly to N fertilization, and at the highest N fertilization rate (N16), Bcr clearly showed the highest leaf biomass production. For all four grass species, the reaction of root biomass to N fertilization was less pronounced in the uncut compared with the cut treatment, although the uncut grass species had significantly higher root biomass at N16 (on average for the grass species; p<0.01).
Figure 1. Shoot and root biomass per pot (g dry matter, DM) of the different grass varieties (Fcr=red fescue var. ‘Cezanne’, Fch=red fescue var. ‘Center’, Bve=velvet bentgrass var. ‘Legendary’, Bcr=creeping bentgrass var. ‘Independence’), at different fertilization regimes (N1, N2, N4, N16 = 12.5, 25, 50, and 200 mg N L−1 respectively in the irrigation water) for (a) cut and (b) uncut grass. Different small letters show significant differences in root and shoot biomass, respectively, between different N fertilization regimes within the four grass species (α = 0.05). ns = not significant.
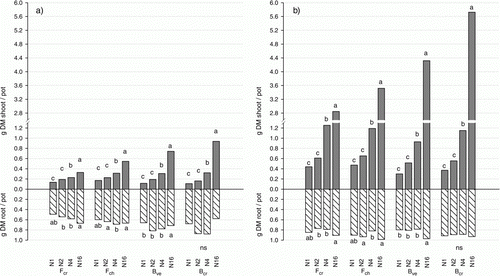
Dry matter partitioning
The proportional allocation of shoot to root biomass increased in response to N supply and for all fertilization regimes it was higher in the uncut treatment (). On average for all species, leaf biomass accounted for 18 and 31% of the total biomass at N1 and 48 and 80% at N16 in the cut and uncut treatment, respectively. Shoot:root ratio for the uncut treatment was generally higher than for the cut treatment. At N1 and N2, the fescues showed higher shoot:root ratio than the bentgrasses, but as the increase in shoot:root ratio due to increased N fertilization was more pronounced for the bentgrasses, they showed the highest shoot:root ratio at N16 ().
Leaf N concentration
Leaf N concentration was strongly influenced by N fertilization and grass species. On average for the species, with increased N fertilization from N1 to N16 leaf N concentration increased from 2.3 to 5.5% of leaf DM for the cut treatment and from 1.3 to 4.1% for the uncut treatment (a and b). The cut fescues showed significantly higher leaf N concentration than the bentgrasses at N1, N2 and N4, but at N16 the bentgrasses showed significantly the highest N concentration. For the uncut treatment this also tended to be true, but differences between the species were not always significant.
Plant growth versus leaf N concentration
The amount of biomass produced during the 3-week experimental period was positively and linearly correlated with the rate of N supply and leaf N concentration (). The slope of this correlation reflects the N productivity of the grass species. The steep slope shown by Bcr denoted a high N productivity, and hence N requirement (d), while Fcr showed the lowest slope and hence the lowest N productivity (a). The relative N demand of the four species, based on their N productivity, was 1:0.67:0.67:0.37 for creeping bentgrass, velvet bentgrass, chewings fescue and slender creeping red fescue, respectively. Considering the lowest N fertilization rate without visible deficiency symptoms as the growth minimum, the corresponding N concentration in clippings was similar for all species and varied between 3.1 and 3.5% N in leaf DM (). However, the N concentration at maximum growth varied between the grass species. The fescues showed an N concentration of 5.1% at growth maximum and the corresponding value for the bentgrasses was 5.9%. In absolute terms the growth capacity of Fcr was about one-third that of Bcr. The leaf N concentration in intact plants at N1–N16 was considerably lower than in plants cut twice a week.
Figure 4. Correlation between leaf N concentration and leaf biomass production after 3 weeks at the different N fertilization rates for the cut turf grass species (a) Fcr, (b) Fch, (c) Bve and (d) Bcr and the N concentration at the lowest growth rate showing no pronounced deficiency symptoms (growth minimum) and highest growth rate achieved in this experiment (growth maximum). Abbreviations as in . Symbols show means of six replicates for the cut and four replicates for the uncut treatment.
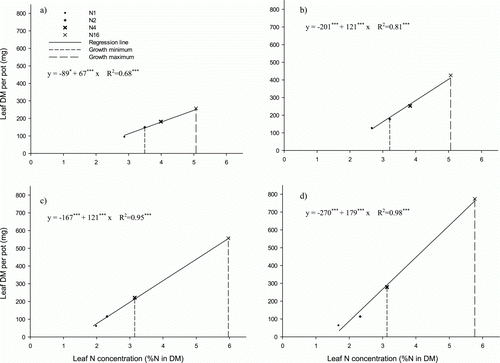
Non-structural carbohydrates and N concentration
The levels of fructans, fructose and glucose in clippings determined in pooled samples from Bcr and Fcr showed an increasing tendency in response to N shortage (). However, this trend could not be statistically proven due to shortage of plant material.
Table II. Content of carbohydrates (% of DM) in clippings (pooled samples) from creeping bentgrass, Bcr, and slender creeping red fescue, Fcr, at different N fertilization levels, 12.5 (N1), 25 (N2), 50 (N4) and 200 (N16) mg N L−1 irrigation water.
Discussion
Our results verified the hypothesis that the relationship between leaf N concentration and above-ground growth rate is linear for frequently mown turf (). Thus the concepts developed by Ingestad and co-workers using data on deciduous tree species and conifers (Ingestad and Lund Citation1986, Ingestad and Ågren Citation1995) can also be used for mown turf grass species. This indicates that it is possible to control many important traits of turf grasses, such as shoot growth, shoot:root ratio, leaf width and stiffness, carbohydrate storage and possibly also the ability to withstand attacks from pests and diseases, through leaf N concentration. In absolute amounts, root production was not closely correlated with N supply (), but on a relative basis plants clearly allocated fewer resources to roots under N-rich conditions than when N was limited (). This agrees with results reported previously for turf grasses (Schlossberg and Karnok Citation2001) and other plant species (Ingestad and Lund Citation1986, Ericsson Citation1995, Glimskär and Ericsson Citation1999).
Target N concentration
An important finding in our study was the common limit for leaf N concentration of between 3.1 and 3.5% in DM to achieve a suitably functioning and healthy looking turf of bentgrasses and fescues. Concentrations between 3.1 and 3.5% N in DM of clippings can thus be used as a target value in order to minimize fertilizer usage and potential N losses due to excess N in the soil. However, values within the range 5–6% N in leaves can be temporarily aimed for when establishing new turf or when repairing turf damaged by winter conditions or other stresses.
Differences between species
Our data support the observation by Ingestad and Lund (Citation1986) and Ingestad and Ågren (Citation1995) that leaf N concentration at growth maximum is higher in fast-growing species than in slow-growing species (). Nitrogen productivity, here defined as aboveground growth per unit time and unit N in leaves, was therefore higher in Bcr compared with Fcr (). Growth and light interception are closely linked processes (Monteith Citation1977, Linder Citation1985) and the ability to grow is correlated with the production cost per unit photosynthetic leaf area (SLA, m2 kg−1 leaf mass). Glimskär and Ericsson (Citation1999), who studied plasticity in five grassland species, found a strong and positive relationship between SLA and relative growth rate at non-limiting N conditions for both grasses and herbs. The thin and broad leaves of bentgrasses compared with the narrow leaves of fescues may offer an explanation for the observed difference in growth capacity between bentgrasses and fescues. No clear difference in leaf morphology was observed between the two fescues, but the ability of Fch to produce more leaves per area unit may make this species a more efficient colonizer of the pot surface than Fcr, and hence more efficient in capturing light (Aamlid and Molteberg Citation2011).
When comparing the different species, the faster-growing bentgrasses allocated relatively more biomass to roots at N1, N2 and N4 than the fescues (). This confirmed that faster-growing species do not reach the target N concentration of 3.1–3.5% in DM at lower N fertilization levels (). As a reaction to this stress situation, they increase allocation of biomass to the roots. In many experiments, fast-growing species have been shown to be more plastic in biomass partitioning in response to N availability (Chapin Citation1980, Bloom et al. Citation1985, Lambers and Poorter Citation1992). In accordance, the fast-growing bentgrasses in the present study clearly had a more pronounced ability to allocate biomass to shoots under favourable N conditions compared with the fescues (). The range of N availabilities created in this study was not large enough to determine whether bentgrasses are also more plastic with regard to biomass allocation to roots at low N availabilities.
The data presented in this study cannot be directly translated into fertilizer recommendations in the form of kg N per 100 m2 and indeed this was not our intention. Each golf course is unique with regard to climate and the soil factors driving growth. However, the species ranking with regard to N productivity (slope of the regressions in ), and hence N requirement, can most probably be applied at any golf course if the optimal N regime of one of the species ranked is known. In the present experiment, Bcr proved most N-demanding and required 63% more N than Fcr and 33% more than Bve and Fch ().
Influence of cutting
This study showed that reducing the leaf area through cutting affects the leaf N concentration positively when the N regime is kept the same (). A reduction in leaf area leads to a decrease in growth capacity and therefore also to a decrease in N demand. As a consequence, N supply must be reduced to meet the decreased growth rate and thereby prevent the leaf N concentration increasing, which would otherwise negatively affect shoot:root ratio (), carbohydrate storage () and leaf morphology (SLA). The same consequence would follow with other stress factors, e.g. shading, drought, lower clipping height and unfavourable temperatures.
Figure 5. Correlations between leaf N concentration of the grasses (%N in DM) and their relative aboveground biomass growth (growth at N1, N2, N4, and N16 in relation to N1) for the cut and uncut (a) fescue species (Fch and Fcr) and (b) bentgrass species (Bcr and Bve). Abbreviations as in . Symbols show means of six replicates for the cut and four replicates for the uncut treatment.
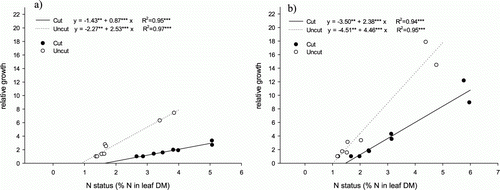
When the same N fertilization regime is maintained, a reduction in leaf area could be expected to change the biomass allocation pattern in favour of shoots. However, we found an opposite allocation pattern. Although the absolute amounts of root biomass were in the same range for the cut and the uncut treatments (), the relative allocation to roots was higher for the cut treatment (). This was true independently of species or fertilization level. However, the root weights determined at the end of the experiment not only represent growth during the experimental period but also root production during turf establishment and the phase of growth acclimation to the different levels of N supply. Determination of root biomass in a representative sample at the beginning of the experimental period would have made it possible to calculate the actual root production and hence compare actual biomass allocation pattern with theoretical. Alternatively, a longer experimental period would give a more accurate picture of root production during the phase when growth is acclimatized to the N supply.
Our intention was to create non-limiting nutrient conditions by saturating the sand twice a week with a nutrient solution containing 200 mg N L−1. N concentrations in the order of 4% in DM of a very slow-growing grass (Danthonia decumbens) and 6% in DM of a fast-growing bentgrass (Agrostis cappilaris) have been reported for non-limiting nutrient conditions by Glimskär and Ericsson (Citation1999). The recorded N concentration in clippings was 5% in DM of fescues and 6% in DM of bentgrasses, indicating that this goal had been fulfilled. The significantly lower N concentrations at N16 in the leaf biomass of both fescues and bentgrasses compared with plants cut twice per week demonstrate that the N requirements of uncut plants were not met with this N regime. However, this shortcoming had no influence on N productivity estimates for uncut species, and thus no influence on the comparison of data obtained under the two clipping regimes.
Carbohydrate storage
The N concentration in leaves is generally negatively correlated to the level of stored carbohydrates in the tissues (McDonald et al. Citation1986, Ericsson Citation1995, Ericsson et al. Citation1996). A similar trend was observed in this study and the levels of fructans increased in response to N limitation. Unfortunately, this trend could not be statistically proven due to shortage of plant material.
Playing quality
According to visual observations, the growth-limiting N supply also reduced leaf width and increased leaf stiffness, i.e. increased production costs per unit leaf area. Reduced SLA is commonly reported in plants grown under N-limiting conditions (McDonald et al. Citation1992, Glimskär and Ericsson Citation1999). Ball roll, and hence playing quality, will therefore most probably also benefit from a reduction in N supply. Reducing N supply instead of lowering clipping height may thus offer an alternative method when improved ball roll is required.
Conclusions
This growth chamber study showed that the relationship between leaf N concentration and aboveground growth is linear for frequently cut turf grass. Therefore it is possible to control many important turf grass traits, such as growth rate, shoot:root ratio, leaf morphology and carbohydrate storage, through the leaf N concentration. The slope of the linear relationship between leaf N concentration and growth, i.e. N productivity, can be used as a tool when ranking turf grass species with regard to N demand. In all, demand-driven fertilization, based on the influence of leaf N concentration on growth-related processes, offers possibilities to control growth in a predictable and desirable manner. This could lead to a more environmentally friendly and economic method of fertilization, and also to better playing quality.
Acknowledgements
The authors wish to thank Monika Kähr, Jaroslav Martinek, Inger Juremalm and Börje Ericson for skilful technical assistance with plant cultivation and chemical analyses. The investigation was financially supported by grants from the Scandinavian Turfgrass and Environment Research Foundation (STERF) and the Departments of Urban and Rural Development and Soil and Environment at the Swedish University of Agricultural Sciences.
References
- Aamlid , T. S. and Molteberg , B. 2011 . Turfgrass species and varieties for Scandinavian golf greens . Soil and Plant Science , 61 : 143 – 152 .
- Ågren , G. I. and Ingestad , T. 1987 . Root:shoot ratio as a balance between nitrogen productivity and photosynthesis . Plant, Cell and Environment , 10 : 579 – 586 .
- Bloom , A. J. , Chapin , F. S. I. and Mooney , H. A. 1985 . Resource limitation in plants . Annual Review of Ecological Systems , 16 : 363 – 392 .
- Chapin , F. S. I. 1980 . The mineral nutrition of wild plants . Annual Review of Ecological Systems , 11 : 233 – 260 .
- Ericsson , T. 1995 . Growth and shoot:root ratio of seedlings in relation to nutrient availability . Plant and Soil, 168–169 205 – 214 .
- Ericsson , T. 2005 . Effects of demand-driven fertilization on growth, appearance, and nitrogen use efficiency of turf grass . Available at http://sterf.golf.se (In Swedish)
- Ericsson , T. , Rytter , L. and Vapaavuori , E. 1996 . Physiology of carbon allocation in trees . Biomass and Bioenergy , 2 : 115 – 127 .
- Glimskär , A. and Ericsson , T. 1999 . Relative nitrogen limitation at steady-state nutrition as a determinant of plasticity in five grassland plant species . Annals of Botany , 84 : 413 – 420 .
- Ingestad , T. 1991 . Nutrition and growth of forest trees . Tappi Journal , 74 : 55 – 62 .
- Ingestad , T. and Ågren , G. I. 1988 . Nutrient uptake and allocation at steady state nutrition . Physiologia Plantarum , 72 : 450 – 459 .
- Ingestad , T. & Ågren , G. I. 1995 . Plant nutrition and growth: basic principles . Plant and Soil, 168–169 15 – 20 .
- Ingestad , T. and Lund , A.-B. 1986 . Theory and techniques for steady state mineral nutrition and growth of plants . Scandinavian Journal of Forest Research , 1 : 439 – 453 .
- Jones , J. R. 1980 . Turf analysis . Golf Course Management , 48 : 29 – 32 .
- Lambers , H. and Poorter , H. 1992 . Inherent variation in growth rate between higher plants: a search for physiological causes and ecological consequences . Advances in Ecological Research , 23 : 187 – 261 .
- Larsson , S. , Wirén , A. , Lundgren , L. and Ericsson , T. 1986 . Effects of light and nutrient stress on leaf phenolic chemistry in Salix dasyclados and susceptibility to Galerucella lineola (Coleoptera) . Oikos , 47 : 205 – 210 .
- Linder , S. 1985 . “ Potential and actual production in Australian forest stands ” . In Research for forest management , Edited by: Landsberg , J. J. and Parsons , W. 11 – 35 . Melbourne : CSIRO .
- McDonald , A. J. S. , Ericsson , A. and Lohammar , T. 1986 . Dependence of starch storage on nutrient availability and photon flux density in small birch (Betula pendula Roth.) . Plant Cell and Environment , 9 : 433 – 438 .
- McDonald , A. J. S. , Lohammar , T. and Ingestad , T. 1992 . Net assimilation rate and shoot area development in birch (Betula pendula Roth.) at different steady-state values of nutrition and photon flux density . Trees , 6 : 1 – 6 .
- Mills , H. A., & Jones , J. B. Jr 1996 . Plant analysis handbook II . Athens , GA : Micro-Macro Publ ., Inc .
- Monteith , J. L. 1977 . Climate and efficiency of crop production in Britain . Philosophical Transactions of Royal Society of London Series B , 281 : 277 – 294 .
- Schlossberg , M. J. and Karnok , K. J. 2001 . Root and shoot performance of three creeping bentgrass cultivars as affected by nitrogen fertility . Journal of Plant Nutrition , 24 : 535 – 548 .
- Smith , G. S. , Cornforth , I. S. and Henderson , H. V. 1985 . Critical leaf concentrations for deficiencies of nitrogen, potassium, phosphorus, sulphur and magnesium in perennial ryegrass . New Phytologist , 101 : 393 – 409 .
- Steen , E. and Larsson , K. 1986 . Carbohydrates in roots and rhizomes of perennial grasses . New Phytologist , 104 : 339 – 346 .
- Waring , R. H. , McDonald , A. J. S. , Larsson , S. , Ericsson , T. , Wiren , A. , Arwidsson , E. , Ericsson , A. and Lohammar , T. 1985 . Differences in chemical composition of plants grown at constant relative growth rates with stable mineral nutrition . Oecologia , 66 : 157 – 160 .