Abstract
Fertilizers and pesticides applied to home lawns can contaminate surface and groundwater making them unfit for aquatic organisms and human consumption. Application of fertilizers and pesticides can improve the aesthetics of a home lawn, improve turfgrass shoot and root density, water use, organic matter accumulation, and other factors known to be influential in urban hydrology. A field study determined the impact of applying home landscape pesticides with or without supplemental irrigation of leaching into groundwater or runoff to surface water. Free-draining lysimeters having a sandy loam soil (coarse-loamy, mixed, active, mesic Lamellic Hapludalf) were used to monitor total volume and nutrients and pesticides losses. Samples from natural precipitation events were analysed for pendimethalin [N-(1-ethylpropyl)-3,4-dimethyl-2, 6-dinitrobenzenamine] applied in spring, mecoprop [2-(4-Chloro-2-methylphenoxy) propionic acid]+2, 4-D (2,4-dichlorophenoxyacetic acid) applied both in late spring and early fall, and bifenthrin [(2-methyl-1,1-biphenyl-3-y1)-methyl-3-(2-chloro-3,3,3-trifluoro-1-propenyl)-2,2-dimethyl cyclopropanecarboxylate] applied in late summer. Pesticide concentrations fluctuated over time, but no differences in concentrations were observed from irrigated or non-irrigated turfgrass for any pesticide. Mecoprop and 2,4-D were the most mobile and values found in runoff during establishment were above maximum contaminant levels (MCL) for U.S. standards. Surface runoff of lawn pesticides were observed at concentrations several magnitudes higher than leachate. The total extent of pesticide runoff was 0.71%, 1.3%, 6.0%, and 0.003% of the amount applied for 2,4-D, mecoprop, pendimethalin and bifenthrin, respectively. With the exception of one sampling period, pendimethalin concentrations in runoff and leachate were low; however, when applied during a period of minimal turf growth and high precipitation, pendimethalin could contribute significantly to surface water pollution.
Introduction
Turfgrass often receives scrutiny for its environmental record including water quality near golf courses, sports complexes, and residential sites. The use of pesticides on turfgrass has a tremendous impact on productivity and quality by reducing the impact weeds, insects, and disease have on color, uniformity, and density (Smith and Bridges Citation1996). Approximately 27×106 kg of active pesticide ingredients are applied to turf annually in the USA to control damage caused by pests (Petrovic and Larsson-Kovach Citation1996).
Factors that can affect pesticide and nutrient loss include: time interval between chemical application and precipitation event; amount and duration of precipitation event; antecedent soil moisture; slope; amount and method of chemical application; timing of chemical application in regard to plant uptake; chemical properties; rate of field degradation/transformation; soil properties; and vegetation type or density (Cole et al. Citation1997). Significant portions of turf-applied pesticides can become mobile in soil solution (Smith and Bridges Citation1996, Cole et al. Citation1997, Starrett et al. 2000). Most often the highest concentrations of pesticides in runoff and leachate occur during the first rain event following application and concentrations diminish with time (Smith and Bridges Citation1996). In a simulated rainfall experiment, Smith and Bridges (1996) found that detec concentrations of mecoprop in a greenhouse lysimeter study only occurred in the leachate for 21 d after treatments were applied. For herbicides 2, 4-D (2,4-dichlorophenoxyacetic acid), mecoprop [2-(4-chloro-2-methylphenoxy)propionic acid], and dicamba (3,6-dichloro-2-methoxybenzoic acid)], the total analyte transported from the greenhouse lysimeters was less than 1.0% of the herbicide applied.
However Baris et al. (2010) found limited evidence that pesticide use as part of turf management on golf courses was causing significant impacts on ground water quality. Additionally, pesticides known to be associated with the turfgrass industry have been measured in 85% of storm runoff events in urban watersheds (dicamba, mecoprop, and dimethylamine salt of 2,4-dichlorophenoxyacetic acid [2,4-D]) (Wotzka et al. Citation1994).
The pathway of nutrient and pesticide loss can be complicated and related to several environmental and physical factors. Turf systems (verdure, thatch, dense roots) can act similarly to a living filter and minimize the amounts of pesticides entering surface and groundwater (Baris et al. Citation2010) which results in a limited amount of pesticide movement offsite. Cisar and Snyder (Citation1996) studied the mobility of pesticides to a USGA golf green and found that less than 0.1% of organophosphate pesticides [chlorpyrifos (0,0-diethyl-0-3,5,6-trichloro-2-pyridyl phosporothioate)], isazophos [0-[5-chloro-l-(methylethyl)-lff-l,2,4-triazol-3yI] 0,0-diethyl phosphorothioate], isofenphos [l-methylethyl 2-[[ethoxy(l-methylethyl) amino] phosphinothioyl]oxy] benzoate], and ethoprop (0-ethyl 5,5-dipropyl phosphorodithioate] applied were recovered in percolate water, regardless of variations in rainfall and total percolation. They concluded that most of the pesticide was retained in the thatch layer and degraded over time. Chlorpyrifos leaching followed a similar trend when only 0.2% of the total was lost during the first application and only 0.08% was lost during the second application even though the percolation volume increased fivefold. Leaching of isazophos and isofenphos applications resulted in less than 0.1% of total applied for both application dates indicating the relatively low mobility of some pesticides even in a sand-based root-zone where infiltration and percolation rates are high.
Model (TurfPQ) predicted runoff losses of pesticides in turfgrass appear to be relatively small. Haith and Rossi (Citation2003) found mean annual pesticide runoff loads did not exceed 3% of the annual applications for any pesticide or site, and applications applied to moss were substantially less than 1% of the application. However, based on field results, TurfPQ underestimates the degree of turf pesticide runoff (Kramer et al. Citation2009). In a simulated runoff study on bermudagrass conducted by Smith and Bridges (1996), over 75% of all the herbicides collected in runoff were transported from the plots during the first rainfall event, and only samples collected over the first 192 hours after treatment contained concentrations above the minimum detectable levels. The concentration of 2, 4-D in the runoff water was a factor above the recommended maximum contaminant level (MCL) of 70 µg L−1 which suggest the potential for waterway contamination and environmental implications. Maximum contaminant levels are standards that are set by the United States Environmental Protection Agency (US EPA) for drinking water quality. An MCL is the legal threshold limit on the amount of a substance that is allowed in public water systems.
The risk to surface and subsurface waterways appears to be small when pesticides are applied to turfgrass at appropriate rates and large precipitation events do not directly follow the application dates.
Turfgrass management practices such as core cultivation and irrigation can influence the extent of pesticide runoff. Rice et al. (Citation2011) found that hollow-tine core cultivation reduced pesticide runoff from golf fairway turf. Irrigation applied immediately after a pesticide application can significantly increase pesticide runoff from turf (Starrett et al. Citation1996, Edwards et al. Citation1998). However, there is no information on how routine irrigation would impact pesticide runoff. Irrigation during dry periods will increase the antecedent soil moisture content and increase the risk of greater runoff.
The objective of this study was to examine the potential losses of commonly applied lawn pesticides, used to control insects and broadleaf and grassy weeds, in response to irrigation on Kentucky bluegrass (Poa pratensis L.) lawns. The goal was to determine if there are differences in hydrology of lawns with and without supplemental irrigation and how irrigation affects the hydrological mobility of commonly used pesticides in the land care.
Materials and methods
The study was conducted at the Turf and Landscape Research Center at Cornell University in Ithaca, NY from 27 August 2007 to 30 November 2009. A southwest-facing hill-slope with a coarse-loamy, mixed, active, mesic Lamellic Hapludalf soil having a slope range of 13–15% (as determined by topographic elevation measurements with a transit) and measuring 39 m×6.1 m was stripped of all sod to a depth of 3.8 cm. Topsoil was removed with a bulldozer and stockpiled for later use. The subsoil was removed to a depth of 45 cm and the underground subsoil was thoroughly compacted with the bulldozer by repeatedly driving over the site. The remaining subsoil and topsoil was replaced and compacted in 15 cm lifts with the bulldozer to ensure consistent compaction levels and consistent bulk densities<1.55 g cm−3 and to provide conditions similar to a new home construction site. The range in soil properties in the surface 60 mm over the test area were: Morgan extractable P of 1.8–2.9 mg kg−1, macroporosity of 0.012–0.024 m3 m3, soil organic matter of 45.6–57.3 g kg−1 and soil pH of 7.0–7.1.
The hill slope was subdivided into eight 1.2 m wide×6.1 m long plots. Lysimeters were constructed similar to Miltner et al. (Citation1996) made of polyvinyl chloride (PVC) pipe with a 61.0 cm diameter and 91.4 cm length. PVC was driven into the ground vertically in the top 1.2 m section of the hill slope, using a loader until the top of the lysimeters was flush with the surface of the subsoil. In half of the plots the lysimeters were removed with the soil core intact, a 10-cm gravel layer was placed at the bottom, and a flat sheet of 12.7 mm thick PVC was attached to the base. If a soil core was not removed intact, the soil was removed in 15-cm lifts and placed in the lysimeters in the same sequence it was removed, and then tamped to ensure the same quantity of soil fit into the lysimeters to maintain the bulk density of the soil profile. Approximately one half of the lysimeters or two of the four replicates were removed with the core intact and the other half were removed by hand. The lysimeters were lowered back into the excavated site.
A trench was placed on the downhill slope of the lysimeters to give access to the base and a 12.7-mm diameter exit drain was placed at the bottom of each lysimeter. Polyethylene pipe (12.7-mm dia.) was connected to each exit drain and tubing was run downhill to a common collection area for leachate sampling in 4000 mL plastic containers. The entire area was covered in approximately 7–8 cm of topsoil and hand raked in preparation for establishments.
The study consisted of a randomized complete block design with the variation in hill-slope used as the blocking factor to provide four blocks with two treatments per block. Plastic landscape edging was placed between each plot to a depth of 50 mm to confine flow of surface water within plots.
Treatments
Treatments consisted of Kentucky bluegrass (KB) lawn that received applications of fertilizers (F) and pesticides (P) using consumer lawn products (the Scotts-Miracle Gro Co., Marysville, OH) that provide four fertilizer applications and four pesticide applications per year; pendimethalin [N-(1-ethylpropyl)-3,4-dimethyl-2, 6-dinitrobenzenamine] in spring, mecoprop [2-(4-chloro-2-methylphenoxy)propionic acid]+2, 4-D (2,4-dichlorophenoxyacetic acid) in late spring and early fall, and bifenthrin [(2-methyl-1,1-biphenyl-3-y1)-methyl-3-(2-chloro-3,3,3-trifluoro-1-propenyl)-2,2-dimethyl cyclopropanecarboxylate] in summer, and supplemental irrigation (I).
Irrigation and treatment applications
Irrigation (1.25 cm) was applied when soil moisture content (determined by time domain reflectometry TDR measures, Spectrum Technologies Inc., Plainfield, IL, model: FieldScout TDR 300) decreased below 20% (v/v) relative water content which was determined to be the level of 50% depletion of plant-available water between field capacity and permanent wilting point of the soil as established by a pressure plate test (Brady and Weil Citation1999). The volume of irrigation water applied did not induce runoff losses from the plots. The second treatment was the same as the first described above, but without supplemental irrigation. All plots were mowed weekly at a height of 5 cm with a rotary mower similar to those used in a home lawn and all clippings were removed. Plots received supplemental fertilization and pesticides according to a seasonal schedule described in based on label recommendations of the products. Plots were sodded on 3 July 2007 with sod containing four different KB cultivars (Odyssey, Liberator, Rugby II, and Total Eclipse). All plots were irrigated to facilitate root establishment and prevent wilt during establishment. Daily climatological data was collected at the Cornell's Turfgrass and Landscape Research Center in Ithaca, New York for the 27-month leachate study period ().
Figure 1. Daily climatological data for Cornell's Turfgrass and Landscape Research Center in Ithaca, New York for the 27-month leachate study period.
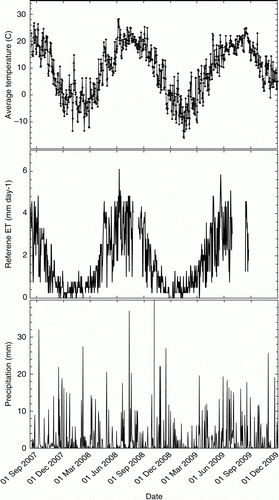
Table I. Application dates and product descriptions of the fertilizer and pesticide combinations applied to the Kentucky bluegrass turfgrass plot.
Runoff and leachate measurements
During establishment, a 12.7-cm wide, 1.20-m long channel drain gutter was placed at the bottom of all plots running perpendicular to the hill slope. The containment gutters were covered with 3.2-mm thick metal sheeting to stop rain and outside contaminants from entering the collection site. The drains for the channel gutters were piped to 100 mL tipping buckets connected to 1000 mL sampling bottles. Tipping buckets were connected to event data loggers (Onset Computer Corp., Pocasset, MA, model HOBO® pendant event logger), calibrated prior to use, and measured the number of tips for the given volume. The number of tips and frequency of tips were measured for each storm event and logged to equate total runoff losses from each plot. Subsamples of leachate and runoff were taken within 6 h of storm events, and frozen immediately until further analysis. Prior to analysis, subsamples were compiled into bulking periods based on the frequency of storms in that time period to obtain 3–6 representative storm samples per time period. Subsamples were bulked proportionally to the volume of runoff and leachate for the given storm events to create bulked subsamples with concentrations proportionate to the representative storm events.
Pesticide analysis
Samples were analysed for the presence of all pesticides applied at the Cornell University Analytical Chemistry Laboratory, New York State Agricultural Experiment Station Geneva, NY 14456. Pendimethalin and bifenthrin were analysed using gas chromatography – electron capture detection (GC/ECD) after the addition of 1 mL of 1.5N HCl to 25 g of water sample. The samples were mixed, and 20 mL of methylene chloride (MeCl2) and 5 g of NaCl were added and shaken for 30 minutes. The samples were then transferred to a separatory funnel and rinsed with an additional 5 mL of MeCl2. After 30 seconds of shaking, layers were allowed to separate and the lower (MeCl2) layer was transferred to a graduated centrifuge tube where samples were evaporated to dryness under nitrogen in a warm (40 °C) water bath. After evaporation, samples were brought up with 2 mL of toluene.
Samples were analysed for 2, 4-D using GC/ECD by extracting 25 mL of the sample water in a 125 mL separatory funnel (with recovery spike added if appropriate) and shaken for one minute with three successive 5 mL portions of MeCl2 added. Methylene chloride was evaporated under nitrogen in a warm water bath and residues were brought up in 2 mL of ethyl acetate. 2-4,-D residues were analysed by methods outlined by Cessna et al. (Citation1985). Mecoprop (MCPP) was analysed using high pressure liquid chromatography (HPLC) after evaporating samples under nitrogen in a water bath (40 °C). Samples were brought up with 2 mL of a 60:40 acetonitrile:water solution then filtered through 0.45µ filter for HPLC analysis as described by Petrovic and Larsson–Kovach (1996) with a detection limit of 0.001 mg L−1.
Soil moisture measurements
Volumetric moisture content of plots were taken periodically using time domain reflectometry (TDR) through a water-content probe (Spectrum Technologies Inc., Plainfield, IL, model: FieldScout TDR 300) with 12-cm deep measurement rods to monitor antecedent moisture conditions and adjust irrigation frequency. Measurements were taken at the top, middle, and bottom of each plot to quantify dynamics that may affect water movement above or below the soil surface.
Pre-established soil infiltration rates were measured within each block on 23 May 2007 using a miniature rain infiltrometer (Ogden et al. Citation1997) and secondary infiltration measurements were taken on 5 June 2008 and 23 September 2009 within each plot to monitor changes in infiltration through the establishment period.
The study was initiated on 10 August 2007 when the first supplemental irrigation event was applied to KB plots receiving irrigation plus pesticides and fertilizer applications and storm events were monitored for 27 months through 1 November 2009.
Statistical analysis
All leaching and runoff pesticide data were subjected to analysis of variance using a mixed model in JMP 8.0 (SAS Institute Citation2009) with repeated measures. Treatment and sample period were treated as fixed effects, while the blocking factor was treated as a random variable in the model. When significant differences were observed, means were separated using Tukey's HSD with α=0.05 probability level.
Soil sample and plot characteristics were subjected to ANOVA with repeated measures with the exception of undisturbed soil core data that was taken only once. If following ANOVA, there was no significant effect of time on a measurement, data for the plot was averaged over time and means were separated using Tukey's HSD test with an α=0.05 probability level when significant effects were observed through ANOVA.
Results and discussion
There were important hydrological differences observed among irrigated and non-irrigated treatments. The application of irrigation to Kentucky bluegrass led to a significantly higher ratio of precipitation occurring as both leachate (A) and runoff (B). The relationship is quite distinct and seasonally based on the sampling periods from which runoff and leachate occurred. The largest differences in runoff and leachate discrepancies occurred during the first nine sample periods which represented the majority of the first calendar year of the study (). Furthermore, leachate volumes were significantly higher under irrigated conditions throughout the course of the study (A). As expected the primary difference observed between the lawns with and without irrigation was the higher soil VWC seen under irrigated KB () along with an increasing soil moisture gradient from the top of the plots to the bottom. The higher VWC observed under irrigated conditions led to an increased potential for soil saturation that resulted in higher leachate volumes and excess runoff to occur. The leachate volumes were consistently higher under irrigated conditions for the entire course of the study (A). The runoff volumes observed were significantly higher under irrigated conditions for the first year of the study, after which there were no differences among the two treatments for the remainder of the study (B).
Figure 2. The observed differences in leachate volume (A) and runoff volume (B) from natural precipitation among irrigated and non-irrigated Kentucky bluegrass turfgrass over a 27-month period. Data break in runoff volume is due to plot renovations. During this period samples were taken and analysed for concentrations; however, no runoff volumes were recorded. Bars represent the standard error of the mean.
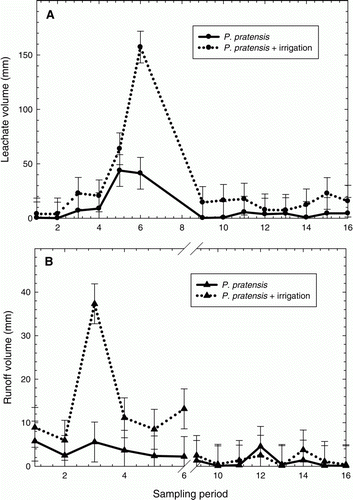
Figure 3. The average soil VWC measured over several dates throughout the growing season under irrigated and non-irrigated Kentucky bluegrass turfgrass. Bars represent the standard error of the mean.
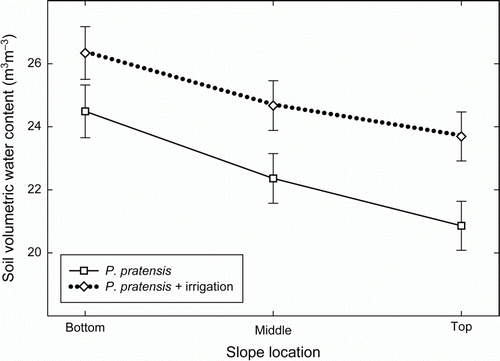
Table II. Leaching and runoff events and corresponding date ranges assigned to sample periods.
Pesticides in leachate
Four pesticides were applied to the KB lawns as part of the preventative pest management program. There were no significant differences observed in the concentrations of any pesticide under irrigated versus non-irrigated KB throughout the study in either runoff or leachate and therefore all data were averaged among the two treatments. Concentrations of pesticides found in leachate varied over time, but higher concentrations were seen directly following applications of the fertilizers containing pesticides () and diminished with time. With the exception of Mecoprop, the transport of these pesticides in leachate to depths that could potentially contaminate subsurface waterways was minimal.
Figure 4. Mean concentrations of four common landscape pesticides found in leachate over time. Due to insignificant differences related to irrigation, values were averaged among treatments that received pesticides with or without irrigation. Bars represent the standard error of the mean. * pesticide application timing.
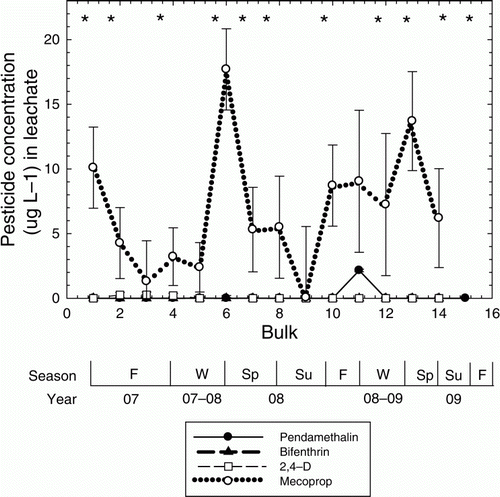
In our study, mecoprop was the most mobile in soil solution and was found in the greatest concentrations in the leachate, contrary to what was expected based on Koc values and water solubility (). Concentration values did not exceed 20.0 µg L−1 throughout the entire study, but did often exceed the US EPA water standard MCL of 10 µg L−1 during several sampling periods (). The largest potential loss of mecoprop in leachate was in sampling periods following the late spring application for broad-leaf weed control. This is also the period of time when there were large leachate volume losses from the plots (). Pendimethalin, bifenthrin, and 2,4-D values in leachate were low and concentrations measured were below US EPA water standards (20, 70 and 10 µg L−1, respectively) and did not show potential for loading significantly to subsurface waterways at any time during the study ().
Table III. Environmental characteristics of four pesticides used in care and maintenance of turfgrass lawn type treatment.
Pesticides in runoff
There was no difference observed in concentrations of pesticides seen in runoff under irrigated or non-irrigated turfgrass, suggesting that an irrigation application designed to meet plant needs will not increase the risk of pesticide runoff. Therefore, data on runoff concentrations were averaged for the two irrigation treatments (). There was a significant effect of sample period on the pesticide concentrations found in runoff, and the establishment period was seen to be an especially critical period in which pesticides could be lost from the environment. There were large peaks in concentrations of both mecoprop and 2, 4-D herbicides in the first fall of the study directly following the first application of the products (). During initial soil infiltration measurements for the plots during establishment, infiltration capacities were<1 cm hr−1, but over time increased dramatically to>10 cm hr−1 for both lawn treatments through the course of the study. This factor influenced the runoff volumes and concentrations of pesticides found in the runoff samples. Beyond the initial fall and for the remainder of the study, runoff concentrations of 2, 4-D and mecoprop were lower with the exception of substantial concentrations of both herbicides observed after the first late spring application (). The values at both of these sampling events were several magnitudes greater than the US water standard MCL for the herbicides and could provide potential harm to aquatic organisms or humans if lost near surface waters. The total extent of 2,4-D and mecoprop runoff was 0.71% and 1.3% of the amount applied, respectively.
Figure 5. Concentrations of four common lawn pesticides found in runoff from Kentucky bluegrass lawn types over a 27-month period. Bars represent the standard error of the mean.
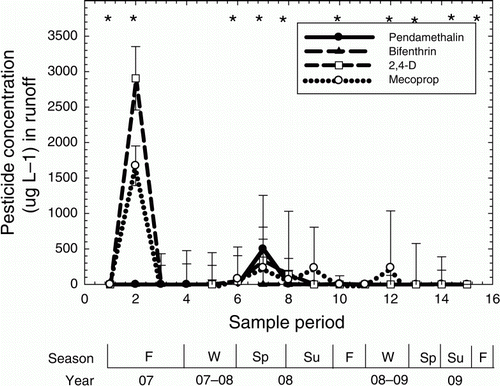
Runoff samples analysed for bifenthrin and pendimethalin were below maximum detection limits (0.001 mg L−1) for the majority of the study, with one large peak of increased pendimethalin concentrations observed after the spring application and during sample period 6 (). The total extent of pendimethalin and bifenthrin runoff was 6.0% and 0.003% of the amount applied, respectively.
The mobility of pesticides in runoff is directly related to the water solubility of the products and hydrologic mobility of 2,4-D and mecoprop was apparent throughout the study as runoff concentrations were substantially higher for these two pesticides.
Conclusion
The hydrological mobility of pesticides applied to the landscape is concerning for aquatic, vegetative, and human health. Differing chemistries of pesticides along with cultural practices applied to the landscape are factors that can impact the transport of herbicides to surface and groundwater from application sites. The process of applying supplemental irrigation to a home lawn has been indicated as a potential mechanism to reduce surface loss of lawn-applied pesticides by washing material off leaves and incorporating them into the soil. This process could reduce the risk of losses through runoff-inducing precipitation events by incorporating products into the soil; allowing for faster plant root uptake or soil immobilization.
In our study, there were trends towards lower concentrations of pesticides in runoff and leachate under irrigated conditions; however, the differences were not statistically significant. The climate of Ithaca, NY is one that receives substantial natural precipitation throughout the growing season, and throughout the course of the study, plots were only irrigated 6–8 times per season. Supplemental irrigation at no time improved the visual density or color of the plots beyond that of the non-irrigated plots and may not have been necessary in the region to improve growth and density beyond what was achieved through natural rainfall. However, supplemental irrigation did maintain a higher average soil VWC than non-irrigated conditions which led to higher leachate and runoff volume losses. This phenomenon could result in higher losses of pesticides, regardless of lower concentrations, because the potential for higher total loading could exist.
References
- Baris , R. D. , Cohen , S. Z. , Barnes , N. L. , Lam , K. and Ma , Q. 2010 . Quantitative analysis of over 20 years of golf course monitoring studies . Environmental Toxicology and Chemistry , 29 : 1224 – 1236 .
- Brady , N. C. and Weil , R. R. 1999 . The nature and property of soils , Prentice-Hall Inc , NJ : Upper Saddle River .
- Cessna , A. J. , Grover , R. , Kerr , L. A. and Aldred , M. L. 1985 . A multi-residue method for the analysis and verification of several herbicides in water . Journal of Agricultural and Food Chemistry , 33 : 504 – 507 .
- Cisar , J. L. and Snyder , G.H. 1996 . Mobility and persistence of pesticides applied to a USGA green. III: Organophosphate recovery in clippings, thatch, soil, and percolate . Crop Science , 36 : 1433 – 1438 .
- Cole , J. T. , Baird , J. H. , Basta , N. T. , Huhnke , R. L. , Storm , D. E. , Johnson , G. V. , Payton , M. E. , Smolen , M. D. , Martin , D. L. and Cole , J. C. 1997 . Influence of buffers on pesticide and nutrient runoff from bermudagrass turf . Journal of Environmental Quality , 26 : 1589 – 1598 .
- Edwards , D. R. , Workman , S. R. and Williams , R. M. 1998 . Response of runoff diazinon concentration to formulation and post-application irrigation . Transaction of American Society of Agricultural Engineers , 41 : 1323 – 1329 .
- Haith , D. A. and Rossi , F. S. 2003 . Risk assessment of pesticide runoff from turf . Journal of Environmental Quality , 32 : 447 – 455 .
- Kramer , K. E. , Rice , P. J. , Horgan , B. P. , Rittenhouse , J. L. and King , K. W. 2009 . Pesticide transport with runoff from turf: Observations compared with TurfPQ model simulation . Journal of Environmental Quality , 38 : 2402 – 2411 .
- Ma , Q. L. , Smith , A. E. , Hook , J. E. , Smith , R. E. and Bridges , D. C. 1999 . Water runoff and pesticide transport from a golf course fairway: Observations vs. Opus model simulations . Journal of Environmental Quality , 28 : 1463 – 1473 .
- Miltner , E. D. , Branham , B. E. , Paul , E. A. and Rieke , P. E. 1996 . Leaching and mass balance of 15N labeled urea applied to Kentucky bluegrass turf . Crop Science , 36 : 1427 – 1433 .
- Ogden , C. B. , van Es , H. M. and Schindelbeck , R. R. 1997 . Miniature rain simulator for measurement of infiltration and runoff . Soil Science Society of America Journal , 61 : 1041 – 1043 .
- Petrovic , A. M. and Larsson-Kovach , I. M. 1996 . Effect of maturing turfgrass soils on the leaching of the herbicide mecoprop . Chemosphere , 33 : 585 – 593 .
- Rice , P. J. , Horgan , B. P. and Rittenhouse , J. L. 2011 . Evaluation of core cultivation practices to reduce ecological risk of pesticides in runoff from Agrostis palustris . Environmental Toxicology and Chemistry , 29 : 1215 – 1223 .
- SAS Institute 2009 . JMP 8.0 . Cary , NC : SAS Institute Inc .
- Smith , A. E. and Bridges , D. C. 1996 . Movement of certain herbicides following application to simulated golf course greens and fairways . Crop Science , 36 : 1439 – 1445 .
- Starrett , S. K. , Christians , N. E. and Austin , T. A. 1996 . Movement of herbicides under two irrigation regimes applied to turfgrass . Journal of Environmental Quality , 254 : 566 – 571 .
- Starrett , S.K. , Christians , N.E. and Al Austin , T. 2000 . Movement of herbicides under two irrigation regimes applied to turfgrass . Advancements in Environmental Research , 4 : 169 – 176 .
- Wotzka , P. J. , Lee , J. , Capel , P. D. , & Lin , M. 1994 . Pesticide concentrations and fluxes in urban watershed. AWRA technical publication series TPS-94-4 , 135 – 145 . Herndon , VA : American Water Resources Association .