Abstract
The interaction of grassland management factors such as plant species, rate of nitrogen (N) fertiliser application and stage of maturity at harvest, will determine the optimal balance of herbage yield, nutritive quality and ensilability for ruminant nutrition and/or industrial applications. This study investigated the effects of N fertiliser input and harvest date on the yield and chemical composition of five common grass species, and made comparisons with red clover. Perennial ryegrass (PRG), Italian ryegrass (IRG), tall fescue, cocksfoot, timothy and red clover were grown under two inorganic N fertiliser inputs (0 kg N ha−1 and 125 kg N ha−1; except red clover) and harvested at five dates (fortnightly from 12 May to 7 July) in the primary growth. Regression analysis of these data allowed comparison of the yield and chemical composition of each grass species at common growth stages, without the confounding effects of variation in maturity between grass species at common harvest dates. Of the grass species investigated, timothy was most productive in terms of dry matter (DM) yield and thus has the potential to provide a cheaper feed per unit DM. However, the most digestible grass species was PRG, with timothy being the lowest, and this could impact on both animal and bioenergy production potential. The most suitable grass species for ensiling was IRG (particularly when grown without fertiliser N) due to its higher water soluble carbohydrate (WSC) concentration and lower buffering capacity (BC) compared to all other grass species. In comparison to the grasses receiving inorganic N fertiliser, red clover had a numerically lower DM yield, but a higher mean DM digestibility and crude protein concentration. The lower WSC concentration and higher BC of the red clover may result in a greater preservation challenge during ensiling.
Introduction
The approximately 0.35 of the utilised agricultural area in Europe under permanent grassland represents an important resource for livestock production (Smit et al., Citation2008), biodiversity and carbon sequestration (Hopkins & Holz, Citation2006), amenity (Green, Citation1990), bioenergy (Murphy & Power, Citation2009) and biorefining (Kromus et al., Citation2004). Three of the main management factors affecting the yield and chemical composition of this grassland are plant species, rate of nitrogen (N) fertiliser application and stage of maturity at harvest.
Harvesting herbage at different growth stages has a major influence on both yield and quality (Buxton, Citation1996). For example, grass harvested at an early vegetative rather than a later growth stage generally has a lower dry matter (DM) yield and fibre content, and a higher digestibility and crude protein (CP) concentration (Buxton & O'Kiely, Citation2003). The optimal stage for harvesting will be determined by the particular grassland use. Thus, grass harvested at earlier growth stages is more suitable for cattle producing high yields of meat (Flynn, Citation1981) or milk (Stakelum & Dillon, Citation1990), and may also be more suitable for biogas production through anaerobic digestion (Murphy et al., Citation2011).
Inorganic N fertiliser is widely used to improve herbage yields (Buxton & O'Kiely, Citation2003). Nitrogen is an essential constituent of proteins, nucleic acids and chlorophyll which are all central to the photosynthetic capacity of plant tissue (Whitehead, Citation1995). However, N fertiliser application can also impact on herbage nutritive quality and ensilability. Thus, increasing N fertiliser application generally increases herbage CP concentration (Wilman & Wright, Citation1983; Keady et al., Citation2000) and buffering capacity (BC; O'Kiely et al., Citation1997), and reduces herbage DM (Whitehead, Citation1995) and water soluble carbohydrate (WSC; Tremblay et al., Citation2005) concentrations.
The majority of reseeded temperate grassland in Western Europe is dominated by perennial ryegrass (Lolium perenne L.) due to its relatively high digestibility, high yield in response to N fertiliser application and ease of preservation as silage due to its relatively high WSC content (Whitehead, Citation1995). However, other grass species (e.g., cocksfoot (Dactylis glomerata L.), tall fescue (Festuca arundinacea Schreb.) and timothy (Phleum pratense L.)) have different physical and chemical characteristics which may offer benefits for non-agricultural uses or be more suited to specific management (e.g., mechanical harvesting, response to fertiliser) or environmental (e.g., temperature, water deficit, solar radiation) conditions. For example, tall fescue exhibits a greater tolerance to drought, heat and cold than perennial or Italian ryegrasses Italian ryegrass (Lolium multiflorum Lam.; Casler & Kallenbach, Citation1995).
Legumes such as red clover (Trifolium pratense L.) also represent a prominent component of some temperate grasslands (Peeters et al., Citation2006). They can be grown alone or in combination with grasses, fix atmospheric N, and can increase herbage yield and quality (Peyraud et al., Citation2009). However, legumes generally have a lower concentration of WSC and a higher BC than grasses, making them more difficult to preserve as silage (Buxton & O'Kiely, Citation2003).
Little information is available on the interactions between grass species and N fertiliser application at common growth stages of the primary growth, and their effect on herbage yield and chemical composition. This information is required when deciding the optimal balance of yield, nutritive quality and ensilability for ruminant nutrition or industrial applications. Thus, the objectives of this study were to (1) quantify the interaction effects of N fertiliser input and harvest dates in the primary growth on the yield and chemical composition of common grass species (using a single variety to represent each species), (2) to compare the yield and chemical composition of these grasses at common growth stages and (3) to relate the yield and chemical composition of these grasses with red clover.
Materials and methods
The experiment comprised replicated field plots that were organised in a split-split plot design with five levels of harvest date as the main plot, two levels of N fertiliser as the sub-plot and five levels of grass species as the sub-sub plot, with triplicate replication. The plots were harvested in two successive years. Monocultures of red clover were also sown in adjacent sub-sub plots.
Experimental plots, harvesting and sampling
In each of three replicate blocks, five common grass species [perennial ryegrass (PRG) (Lolium perenne L. var. Gandalf), Italian ryegrass (IRG) (Lolium multiflorum Lam. var. Prospect), tall fescue (Festuca arundinacea Schreb. var. Fuego), cocksfoot (Dactylis glomerata L. var. Pizza), timothy (Phleum pratense L. var. Erecta)] and one leguminous species [red clover (Trifolium pratense L. Merviot)] were grown in field plots (20 m2) at Grange, Co. Meath, Ireland (53°30′N, 6°40′W, 83 m above sea level). The soil type at Grange was a moderately-drained brown earth and it had a pH of 6.3, Morgan's P of 12.9 mg L−1 and K of 269 mg L−1 (Black & O'Kiely, 2007).
Within each block, the 10 plots per grass species (five for red clover) were managed under two inorganic fertiliser N inputs (zero = 0 kg N ha−1, high = 125 kg N ha−1; applied as urea (460 g N kg−1) in mid-March when soil temperature >6°C; except clover which received no fertiliser N input) and were harvested at five dates (fortnightly from 12 May to 7 July; Harvests 1 to 5) in the primary growth during both 2009 and 2010. There were 165 plots in total. Mean monthly weather conditions during May and July in both years are presented in . At each harvest date, herbage was harvested and weighed using a Haldrup forage plot harvester (J. Haldrup, Løgstor, Denmark) cutting to a 6 cm stubble height. A representative 2 kg sample of each herbage was taken and stored at –18°C prior to chemical analyses.
Table I. Mean monthly weather conditions during harvesting in 2009 and 2010.
After harvesting in 2009, the grass plots received 250 kg ha−1 of a compound fertiliser (240 g N, 25 g P and 100 g K kg−1; Cut Sward, Grassland Fertiliser (Kilkenny) Ltd., Palmerstown, Kilkenny, Ireland), while the red clover plots received 250 kg ha−1 of a fertiliser containing 0 g N, 70 g P and 300 g K kg−1. Subsequent regrowths of all plots were defoliated on the same dates in mid-August and mid-October.
Botanical composition and growth stage
Prior to harvesting, the botanical composition of the herbage on each plot was assessed visually and herbage growth stage was determined for 20 randomly sampled plants from each plot, according to Moore et al. (Citation1991) for grass and Ohlsson and Wedin (Citation1989) for red clover.
Chemical analysis
The DM concentration was estimated following drying in a ventilated oven with forced air circulation at 98°C for 16 h. Replicate samples (200 g) were dried at 40°C for 48 h before being milled (Wiley mill; 1 mm screen). Dried, milled samples were used for the determination of dry matter digestibility (DMD), neutral detergent fibre (NDF), acid detergent fibre (ADF), acid detergent lignin (ADL), ash, BC, CP and WSC concentrations. In vitro DMD was determined by the method of Tilley & Terry (Citation1963) but with the final residue being isolated by filtration (Whatman GF/A 55 mm, pore size 1.6 mm, Whatman International, Maidstone, UK) rather than centrifugation. The NDF (assayed with a heat stable amylase and sodium sulphite), ADF and ADL were determined by the method of Van Soest (Citation1963) using ANKOM filter bag technology (Ankom, Citation2006a,Citationb) and expressed exclusive of residual ash. The ash content was determined by complete combustion in a muffle furnace at 550°C for 5 h while the BC was measured according to the method of Playne & McDonald (Citation1966) using an 809 Titrando Universal Titrator and Titrosampler (Metrohm, Herisau, Switzerland). The CP concentration (N×6.25) was determined using a LECO FP 428 N analyser (Leco Instruments, MI, USA) based on method 990–03 of the (AOAC, Citation1990). Concentrations of WSC were determined using the anthrone method (Thomas, Citation1977) on an Autoanalyser 3 (Bran and Leubbe GmbH, Norderstedt, Germany).
Statistical analysis
Data relating to grasses were analysed as a split-split-plot design using the MIXED procedure of SAS, Version 9.1.2 (SAS, Citation2004). The structure used harvest date as the main-plot, N fertiliser as the sub-plot and grass species as the sub-sub plot, and with the effect of replicate block being accounted for within the main plot. As the same plots were used in both years, a repeated measures statement was used to account for the effect of years. The Tukey adjustment for multiple comparisons was used in testing for differences between means. Regression analysis was used to relate grass yield and chemical composition to growth stage and was undertaken using the MIXED procedure of SAS. Red clover was not included in the above model to allow a more efficient and clear-cut assessment of the interactions between harvest date, N fertiliser and grass species. Data for red clover were analysed separately as a randomised complete block design using the MIXED procedure of SAS.
Results
Botanical composition and growth stage at harvest
Using visual assessment, 0.89 of plots contained >0.90 of their sown species. Of the five grass species investigated, timothy was at the least advanced growth stage at each harvest date (). In contrast, but with the exception of Harvest 1, cocksfoot was at a more advanced growth stage than the other grass species. The PRG and IRG were at a similar growth stage to one another at each harvest date.
Figure 1. Growth stage of five grass species harvested at five sequential dates (fortnightly from 12 May to 7 July; Harvests 1 to 5) in the primary growth in 2009 and 2010. Growth stage was determined according to Moore et al. (1991) where stage 1.0−1.9 = vegetative-leaf development, 2.0–2.9 = elongation–stem elongation and 3.0–3.9 = reproductive-floral development.
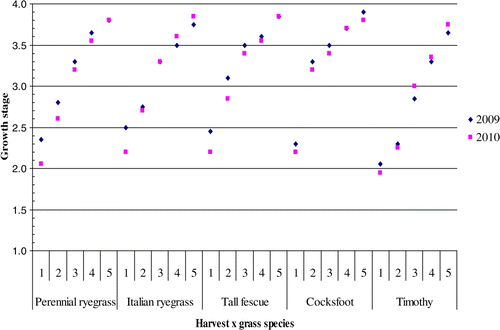
Main factor effects
Harvest date
On average, there was an increase (P<0.001) in DM yield with advancing harvest date (except from Harvest 4 to 5 where there was a decrease (P<0.05)), together with an increase (P<0.001) in herbage DM concentration and an increase (P<0.001) in the concentrations of NDF, ADF and ADL (). In contrast, with advancing harvest date there was a decrease (P<0.001) in DMD, BC and the concentrations of CP and WSC. Ash concentration declined (P<0.01) from Harvest 1 to 5.
Table II. Yield (t DM ha−1) and chemical composition (g kg−1 DM, unless indicated otherwise in footnotes) of five grass species harvested at five dates in the primary growth (averaged across year and nitrogen fertiliser treatment).
Nitrogen fertiliser
Of the two N fertiliser treatments employed, the high N treatment gave a higher (P<0.05) DM yield and resulted in herbage with a higher concentration of ADF (P<0.05), ADL (P<0.01) ash (P<0.001) and CP (P<0.001), and a higher (P < 0.001) BC compared to the zero N fertiliser treatment (). In contrast, herbage DM (P<0.001) and WSC (P<0.001) concentrations were higher for the zero N treatment.
Table III. Yield (t DM ha−1) and chemical composition (g kg−1 DM, unless indicated otherwise in footnotes) of a five grass species grown under two nitrogen fertiliser inputs (averaged across year and harvest date).
Grass species
Of the five grass species investigated, on average cocksfoot had the lowest (P<0.001) DM yield (with no significant difference observed between the other grass species) and DMD (along with IRG and tall fescue), and the highest BC (P<0.001), ash (P<0.001) and CP concentrations (P<0.001, along with timothy; ). The PRG and timothy grasses had similarly higher (P<0.001) DMD values compared to the other grass species. The IRG had the highest DM (P<0.001) and WSC (P<0.001) concentrations, and the lowest (P<0.001) NDF and ADF concentrations and BC. In contrast, timothy had the highest (P<0.001) NDF and ADF concentrations and had a similarly high ADL concentration to cocksfoot. On average, ADL (P<0.05) and CP concentrations were lowest (P<0.001) for the two ryegrass species.
Two-factor interactions
Harvest date×nitrogen fertiliser
Herbage DM yield was higher (P < 0.05) for the high compared with the zero N fertiliser treatment for Harvest 1 and 4 only. In general, herbage CP concentration (P < 0.01) and BC (P < 0.01; Harvests 1 to 3 only) were higher at each harvest date for the high compared to the zero N fertiliser treatment, while the opposite was the case for herbage DM (Harvests 1 to 3 only) and WSC (Harvests 1 and 2 only) concentrations (). Although statistically significant (P < 0.05), there were only small differences in herbage ADF concentration between the two N fertiliser treatments at each harvest date.
Table IV. Yield (t DM ha−1) and chemical composition (g kg−1 DM, unless indicated otherwise in footnotes) of herbage grown under two nitrogen fertiliser inputs and harvested at five dates in the primary growth (averaged across year and grass species).
Harvest date×grass species
In general, grass DM yield was higher at the later rather than the earlier harvest date. An exception to this was observed for each individual grass species between Harvest 4 and 5 where there was a decrease in DM yield for the later harvest date (less so for tall fescue), but this trend was not significant (). The DM yield of cocksfoot was lower (P < 0.05) than all other grasses at Harvests 3 (except PRG) and 5 (except PRG) only. In general for the five grass species investigated, herbage DM concentration increased (P < 0.001) with advancing harvest date. An exception to this trend was for the cocksfoot where a decrease (P < 0.001) in DM concentration was observed from Harvest 4 to 5. The DMD was lower (P < 0.01) in cocksfoot than all other grasses at Harvest 4 only. Conversely, ash concentration was higher (P<0.001) in cocksfoot than all other grass species at Harvest 5 and was higher (P < 0.01) than both ryegrasses at Harvest 3 only. There was a significant increase (P<0.001) in the ash concentration of cocksfoot from Harvest 4 to 5, while at other harvest dates ash concentration did not differ. Further significant interactions between harvest date and grass species were not clear cut.
Nitrogen fertiliser×grass species
With the exception of IRG, the average DM yield of each grass species was higher under the high compared with the zero N fertiliser treatment, but this difference was only significant for the tall fescue (P < 0.05; ). Herbage DM concentration was higher (P < 0.05) for the IRG and timothy under the zero N fertiliser treatment but no significant difference was observed for the other grass species. The NDF concentration of each grass species did not differ between N fertiliser treatments. However, under the zero N fertiliser treatment the NDF concentration was higher (P < 0.05) in timothy than all other grass species, while under the high N fertiliser treatment the NDF concentration did not differ significantly between timothy, tall fescue and cocksfoot. Of the five grass species investigated, a higher (P < 0.05) ADF concentration was observed for the PRG only, under the high compared to the zero N fertiliser treatment. The IRG, grown under both the high and zero N fertiliser treatments, had a higher (P < 0.001) WSC concentration than all other grass species. Under the high N fertiliser treatment the WSC concentration was higher (P < 0.01) in cocksfoot than in timothy, while no difference (P < 0.05) was observed at the zero N treatment. Cocksfoot grown under the zero N fertiliser treatment had a higher (P < 0.001) BC than all other grass species, however, under the high N fertiliser treatment the BC of cocksfoot was only higher (P < 0.001) than IRG, with no difference observed between other grass species.
Three-factor interactions
There were no significant interactions between harvest date, nitrogen fertiliser and grass species for any of the variables measured.
Regression analyses
Regression analysis of the grass data allows a comparison of yield and chemical composition of each grass species at the same phenological growth stage, instead of at the five harvest dates where growth stages differed. shows the calculated DM yield and chemical composition of each of the five grass species regressed at two growth stages, for each of two successive years. These growth stages, 2.7 (stem elongation) and 3.4 (reproductive-floral development), correspond to two dates (24 May and 17 June for 2009 and, 27 May and 18 June for 2010, respectively) in the primary growth at which perennial ryegrass would typically be harvested on Irish farms depending on whether high or moderate digestibility silage was required.
Table V. Calculated (using regression equations; Appendix I) yield (t DM ha−1) and chemical composition (g kg−1 DM, unless indicated otherwise in footnotes) at two contrasting stages of growth for five grass species grown under two nitrogen fertiliser treatments in 2009 and 2010.
From the regression analyses it is clear that year to year variation exists for grass DM, DMD and NDF, ADF and ADL concentrations, while variation between N fertiliser treatments was observed for DM yield, DMD, BC and ADL, ash, CP and WSC concentrations ( and Appendix I).
Red clover
On average, there was an increase (P < 0.01) in the DM yield of red clover from Harvests 1 to 5 (). Herbage DM (P < 0.01; Harvest 1 to 4 only), NDF (P < 0.01), ADF (P < 0.01) and ADL (P < 0.05; Harvests 2 to 5 only) concentrations increased from Harvest 1 to 5. In contrast herbage DMD (P < 0.01) and BC (P < 0.05; Harvest 1 to 4 only) and WSC (P < 0.001) and CP (P < 0.05) concentrations decreased with advancing harvest date. There was no significant difference in ash concentration across the five harvest dates.
Table VI. Growth stage, yield (t DM ha−1) and chemical composition (g kg−1 DM, unless indicated otherwise in footnotes) of red clover harvested at five sequential dates in the primary growth (averaged across year).
Discussion
Harvest date
Increasing grass DM yield with advancing harvest date has been well-documented (Kunelius et al., Citation1974; Binnie et al., Citation1980; Mason & Lachance, Citation1983), and in the current study approximately 0.76 of the increase in DM yield with advancing harvest date was contributed by an increase in NDF yield. The average 0.12 decline in yield from Harvest 4 to 5 agrees with the profile for grass DM yield reported by Han et al. (Citation2003). The mean air temperature for the two weeks preceding Harvest 5 was higher than the two weeks preceding Harvest 4 (16.2 vs. 13.9°C) and could have caused increased respiration and senescence (; Penning de Vries et al., Citation1979). In particular, leaf senescence and remobilisation of storage carbohydrates can lead to a reduction in plant biomass after flowering (Calser & Van Staten, Citation2010).
The average rate of decline in herbage DMD with advancing harvest date (across all grass species) was 3.3 g kg−1 day−1, with the fastest rate of decline between Harvests 2 and 3 corresponding to the change from the elongation to the reproductive growth stages. The PRG digestibility declined at a numerically faster rate (3.9 g kg−1 day−1) than the perennial ryegrass reported by Keating & O'Kiely (Citation2000a; 2.7 g kg−1 day−1), and than the other grass species (3.2 g kg−1 day−1) in the current study. The latter reflects the numerically higher DMD for PRG at Harvest 1. This faster rate of decline for PRG in the current study corresponds to its numerically faster rate of increase in NDF and ADF concentrations (2.9 and 2.4 g kg−1 day−1, respectively) than other grass species (2.3 and 2.1 g kg−1 day−1). However, these rates of increase in NDF and ADF concentrations were lower than reported by Čop et al. (Citation2009; 7.2 and 5.2 g kg−1 DM day−1, respectively).
On average, the decrease in herbage CP concentration with advancing harvest date was greatest (2.1 g kg−1 DM day−1) between the elongation and reproductive growth stages and was least after heading (0.6–0.7 g kg−1 DM day−1), which is in accord with Mason & Lachance (Citation1983).
The decrease in BC and WSC concentration with advancing plant maturity can be attributed to changes in chemical composition resulting from the declining cell content to cell wall ratio. These variables declined at an average daily rate of 3.2 mEq kg−1 DM and 1.6 g kg−1 DM, respectively, which was faster than reported by Muck et al. (Citation1991) for BC (2.5 mEq kg−1 DM day−1) but similar to that reported by Tremblay et al. (Citation2005) for WSC concentration (1.4 g kg−1 DM). When all grass species reached their reproductive growth stage further declines in BC were at a slower rate.
Nitrogen fertiliser
On average in the current study, the DM yield response to the high N fertiliser treatment was relatively small (i.e., 9.9 kg DM kg−1 N applied ha−1 in the current study) compared to the 29.3 kg DM kg−1 N applied ha−1 reported by Keating & O'Kiely (Citation2000b) for perennial ryegrass. However, herbage DM yield in the absence of fertiliser N in the current study was high in comparison to Keating & O'Kiely (Citation2000b; Harvest 2 = 5.77 vs. 2.45 t DM/ha, respectively), thereby resulting in a lower subsequent response of DM yield to fertiliser N and this can be partly explained by the high N supply inherent in the soil at Grange (Travers, Citation1999).
The lower DM concentration of the herbage grown under the high fertiliser N treatment (229 vs. 244 g kg−1 for the high and zero N rates, respectively) may be partly explained by an increase in the amount of internal water in the leaves and partly by the development of a larger crop capable of retaining more superficial water from dew or rainfall (Whitehead, Citation1995). The small differences (across species and harvest date) observed in herbage DMD and NDF in response to increased N fertiliser application agree with Valk et al. (Citation1996) where minimal changes in DMD would be expected under the prevailing conditions of N availability in the soil.
The increase in herbage BC and CP concentration following the application of N fertiliser is well documented (Muck et al., Citation1991). On average, the rate of increase in CP concentration was 0.25 g kg−1 DM per 1 kg fertiliser N added, in accord with Reid (Citation1970) who found that the rate of increase in CP concentration in response to fertiliser N was generally linear from 0 to 673–785 kg N ha−1 rates. The decrease in WSC concentration in response to the high fertiliser N treatment is in accord with Nowakowski (Citation1962) and McGrath (Citation1992) and this can be at least partly attributed to an increased growth rate following fertiliser application. Both the higher BC and the lower WSC concentration of herbage under the high N fertiliser treatment would most likely make these grasses more difficult to preserve as silage (Buxton & O'Kiely, Citation2003).
Grass species
The grass species used in the current study are typical of those growing in temperate grassland regions, with perennial ryegrass being the most dominant sown species. Green et al. (Citation1971) reported that ryegrasses had higher yields than other common grass species, which is in contrast to the current study where with the exception of a lower average DM yield for cocksfoot, there was no difference between the other grass species investigated. The reason for the lower DM yield with cocksfoot are not apparent.
Furthermore, different grass species, especially in temperate conditions, can react differently to N fertiliser application. Generally, ryegrass and cocksfoot varieties are reported to produce the best DM yield response to high rates of N fertiliser input (Reid, Citation1985). However, in the current study, each grass, with the exception of IRG, had a numerically positive response to the high N fertiliser treatment, but tall fescue was the only individual species where the response was statistically significant.
Perennial ryegrass is often preferred for animal production because of its high digestibility and WSC concentration (Whitehead, Citation1995). Thus, in the current study, the high DMD of PRG is in accord with Wilson and Collins (Citation1980) and reflects its relatively low ADF concentration. However, at the same harvest dates the variety of timothy used had an equally high DMD. The higher CP concentration for cocksfoot and timothy compared with the other grass species is in contrast to Davies and Morgan (Citation1982) who found that perennial ryegrass had the higher CP concentration compared to other grasses. In general, the higher WSC concentration and the lower BC observed for the two ryegrass species (and IRG in particular), even under the high N fertiliser treatment, would make them more suitable for silage preservation than the other grasses (Jones, Citation1970a; Buxton & O'Kiely, Citation2003).
Comparing grasses at common growth stages
Due to the difficulty in selecting a variety of each grass species with the same phenological growth stages at each of the five harvest dates, it was necessary to examine the yield and chemical composition of each grass species at calculated common growth stages using regression analysis. In order to equate grasses to the two growth stages selected for PRG, both tall fescue and cocksfoot should be harvested earlier (by 3–4 days and 6–7 days, respectively) while timothy should be harvested later (by 8–9 days) than PRG.
The estimated DM yield of each grass species was greater at the later growth stage compared to the early growth stage (3.5 vs. 2.5; Moore et al., Citation1991) which reflects the greater duration of DM production with the advancement from the elongation to reproductive growth stages. The average DM yield was highest for timothy and lowest for cocksfoot with differences between other grass species being small. This finding indicates that timothy was more productive and, as stated by Wilkins and Humphreys (Citation2003), thus has the potential to provide a cheaper feed per unit DM. In contrast, the lower yield achieved with cocksfoot, even when 125 kg N ha−1 was applied, than for other grasses (even where no N fertiliser was applied), would make this an expensive crop to produce. The DM yield for each grass species at the two chosen growth stages was numerically higher for the high than the zero N fertiliser treatment (7.95 vs. 7.30 t DM ha−1), with this response to added N being larger for all species at the early growth stage. This agrees with Whitehead (Citation1995), who stated that the response to fertiliser N is normally greatest during the period immediately before the date of ear emergence of the grass. Were the absence of an effect of year on yield (Appendix I (1)) to be repeatable over a larger number of years, this would mean that much of the apparent year to year variation in yield that occurs at a target harvest date could be avoided by deciding to harvest when the crop reaches a target growth stage instead.
At early and late standardised phenological growth stages, the mean DMD values across the five grass species (averaged over both years and two fertiliser N input rates) ranged from 721 to 767 and 662 to 709 g kg−1, respectively, reflecting the decrease in the cell content to cell wall ratio and the declining digestibility of the cell wall component (Buxton & Redfearn, Citation1997). This change was reflected by corresponding increases in NDF, ADF and ADL concentrations. Averaged across both years and two input rates of fertiliser N, the ADL values of the five grass species ranged from 8.6 to 31.9 and 18.7 to 42.0 g kg−1 DM at the early and late growth stages, respectively, corresponding to the range averaged across six harvest dates in the primary growth for similar grass species found by Jančik et al. (Citation2008; 8.2–42.2 g kg−1 DM). At both growth stages, PRG was at the upper end of the DMD range, in accord with Jones (Citation1970b), while timothy was at the lower end. This is in contrast to the data in where timothy gave a similarly high DMD value to PRG. This apparent change can be explained by timothy being at a less advanced growth stage at each harvest date and the benefit to DMD that this might entail disappeared when the species were equated to common growth stages. The higher DMD for PRG reflects its numerically lower ADL concentration, in accord with Jones (Citation1970b), while the lower value for timothy reflects the higher concentration of NDF and ADF, in agreement with Morrison (Citation1980). Thus, PRG appears to have a higher digestibility than the other grasses which is an important indicator of forage quality impacting on both animal performance (Casler & Vogel, Citation1999) and bioenergy potential (Prochnow et al., Citation2009).
Whereas it had not been anticipated that applying inorganic N fertiliser would alter DMD, and this was the clear outcome in year 2, fertiliser N appeared to affect DMD for some species in year 1. The reasons for this outcome are unclear, but would have the unwanted effect of making it more difficult to achieve expected DMD values when harvesting at a target phenological growth stage in any particular year.
The CP concentration of cocksfoot was higher than the other grass species in this study but similar to mean CP concentration found for cool-season forage grasses by Minson (Citation1990; 130 g kg−1 DM). This higher concentration of CP in cocksfoot, together with the numerical trend for similar increases in NDF and ash concentration largely reflects the effects of the low concentration of WSC in cocksfoot. In circumstances where grass is to be used as a source of CP, increasing the application rate of inorganic fertiliser can enhance this value. The current analysis shows that the CP concentration response to inorganic N fertiliser appeared similar for all grass species, but was about one-third larger at growth stage 2.7 than 3.4.
If each grass species was harvested at a common growth stage, the challenge to silage preservation would be greatest for the timothy and cocksfoot, as evidenced by their low WSC concentrations and high BC. In contrast, whereas both IRG and PRG had relatively high WSC concentrations, the higher WSC concentration and markedly lower BC of IRG means that the latter has a clear advantage in terms of achieving satisfactory silage preservation. This is in agreement with Wilson and Collins (Citation1980), who reported that only 0.25 of cocksfoot and 0.26 of timothy samples preserved satisfactorily as silage compared with 0.97 of Italian ryegrass and 0.72 for perennial ryegrass. The negative effect of added N on ensilability was more evident at the early growth stage (2.7). This was manifest in a larger reduction in WSC concentration at growth stage 2.7 versus 3.4 in response to applying inorganic N fertiliser despite the absence of any such interaction effect on BC. This implies that the overall negative effect of inorganic N fertiliser on ensilability of grasses is larger at the early growth stage.
Knowing the yield, digestibility, fibre or protein concentration and ensilability values for the five grass species at either rate of fertiliser N application, and their rates of change, allows for selection of appropriate combinations for different ruminant nutrition or industrial use requirements. Thus, for example, high yields of fibre would best be provided by N fertilised timothy harvested at an advanced growth stage while highly digestible and readily ensilage ruminant feed would best be provided by PRG or IRG harvested at a more vegetative growth stage.
Red clover
The lower average numerical yield observed for red clover compared to the five grass species receiving inorganic N fertiliser (6.77 vs. 8.25 t DM ha−1) is in accord with Kunelius and Narasimhalu (Citation1983) who reported that N fertilised ryegrass out-yielded legumes in monoculture. However, this was in contrast to Weldon and O'Kiely (Citation2011), who found that red clover monocultures produced a higher annual DM yield than perennial ryegrass that received 200 kg inorganic N ha−1 year−1.
The digestibility of legumes generally declines with advancing phenological growth stages at a slower rate than for forage grasses (Buxton, Citation1996). However, in this study, red clover digestibility declined at a similar rate (3.2 g kg−1 day−1) to grasses. The finding that, at each harvest date, red clover had a numerically lower NDF and ADF concentration but higher ADL concentration than the grasses indicates a different relationship between herbage fibre concentration and digestibility for these grasses and red clover. This is in agreement with Van Soest (Citation1994) who reported that at the same digestibility legumes contain less plant cell wall but about twice the lignin content of grasses.
In addition, the higher CP concentration in red clover compared with the grasses agrees with Dewhurst et al. (Citation2003) and likely reflects the consequences of assimilation of atmospheric N by Rhizobium bacteria in the legume nodules (Frame & Laidlaw, Citation2011). The lower WSC concentration and higher BC of the red clover would be expected to result in a greater preservation challenge during ensiling than for grasses, in accord with Buxton and O'Kiely (Citation2003).
Conclusion
Regression analysis of the grass data allows comparison of the yield and chemical composition of each grass species at common growth stages, free of the confounding effects of variations in maturity between grass species at the same harvest date. As expected, herbage harvested at the later growth stage gave higher yields (8.55 vs. 6.70 t DM ha−1), with this increase being mainly attributed to an increase in NDF yield (2.35–5.93 t NDF ha−1). Of the five grass species investigated, cocksfoot had the lowest (6.24 t DM ha−1) and timothy the highest (8.69 t DM ha−1) yield in the primary growth, which would make cocksfoot a more expensive crop to produce. Overall, N fertiliser increased the DM yield of herbage (7.30–7.95 t DM ha−1), with this low yield response being partly explained by the high background N supply in the soil. The most digestible grass species after equating grasses to the same growth stage was PRG (738 g kg−1), with timothy being the lowest (692 g kg−1), and this could impact on both animal performance and bioenergy potential. As expected, herbage digestibility was lower at the later growth stage (679 vs. 738 g kg−1) corresponding to higher concentrations of fibre components (NDF, ADF and ADL), with the timothy species on average having the highest concentrations of NDF and ADF.
The most suitable grass species for ensiling was IRG (particularly when grown without fertiliser N) as it had a higher WSC concentration (221 g kg−1 DM) and a lower BC (372 mEq kg−1 DM) compared to the average of the non-ryegrass species (124 g kg−1 DM and 424 mEq kg−1 DM, respectively). Losses during ensilage can impact on subsequent animal and bioenergy production potential. In comparison to the grasses receiving inorganic N fertiliser, red clover had a numerically higher mean DMD, CP concentration and BC, and a lower DM yield and WSC concentration.
Acknowledgements
The authors thank B. Weldon, Grange farm staff and the staff of Grange Laboratories. The statistical analysis guidance of J. Grant is also acknowledged. Funding was provided under the National Development Plan, through the Research Stimulus Fund (#RSF 07 557), administered by the Department of Agriculture, Food & the Marine, Ireland.
References
- Ankom ( 2006a ). Acid Detergent Fiber in Feeds Filter Bag Technique ( Macedon , NY : Ankom Inc ).
- Ankom ( 2006b ). Neutral Detergent Fiber in Feeds Filter Bag Technique ( Macedon , NY : Ankom Inc .).
- Association of Official Analytical Chemists (AOAC) 1990 . Official Methods and Analysis . First supplement (1990) to the 15th edition, method 990–03 . ( Arlington , VA : AOAC ).
- Binnie , R.C. , Chestnutt , D.M.D. and Murdoch , J.C. 1980 . The effect of initial defoliation and 5 height of defoliation on productivity of Lolium perenne swards . Grass and Forage Science , 6 : 267 – 273 .
- Black , A.D. & O'Kiely , P. 2003 . Seedling growth and development characteristics of perennial clover species compared with perennial ryegrass . In Grassland Science in Europe, 12. Proceedings of the 14th General Meeting of the EGF: Permanent and temporary grassland: plant, environment, economy , Ghent, Belgium 596 .
- Buxton , D.R. 1996 . Quality-related characteristics of forages as influenced by plant environment and agronomic factors . Animal Feed Science and Technology , 59 : 37 – 49 .
- Buxton , D.R. and O'Kiely , P. 2003 . “ Preharvest plant factors affecting ensiling ” . In Silage Science and Technology , Edited by: Buxton , D.R. , Muck , R.E. and Harrison , J.H. 199 – 250 . Madison , Wisconsin : American Society of Agronomy, Crop Science Society of America, Soil Science Society of America .
- Buxton , D.R. and Redfearn , D.D. 1997 . Plant limitations to fiber digestion and utilization . Journal of Nutrition , 127 : 814S – 818S .
- Casler , M.D. and Kallenbach , R.L. 1995 . “ Cool-season grasses for humid areas ” . In Forages – the science of grassland agriculture , Edited by: Barnes , R.F. , Nelson , C.J. , Moore , K.J. and Collins , M. 211 – 220 . Ames , Iowa : Blackwell Publishing .
- Casler , M.D. and Vogel , K.P. 1999 . Accomplishments and impact from breeding for increased forage nutritional value . Crop Science , 39 : 12 – 20 .
- Calser , M.D. and Van Staten , E. 2010 . “ Breeding objectives in forages ” . In Fodder Crops and Amenity Grasses , Edited by: Boller , B. , Poselt , U.K. and Veronesi , F. 115 – 136 . London , LLC : Springer Science and Business Media .
- Čop , J. , Lavrenčič , A. and Košmelj , K. 2009 . Morphological development and nutritive value of herbage in five temperate grass species during primary growth: Analysis of time dynamics . Grass and Forage Science , 64 : 122 – 131 .
- Davies , D.A. and Morgan , T.E.H. 1982 . Herbage characteristics of perennial ryegrass, cocksfoot, tall fescue and timothy pastures and their relationship with animal performance under upland conditions . Journal of Agricultural Science , 99 : 153 – 161 .
- Dewhurst , R.J. , Fisher , W.J. , Tweed , J.K.S. and Wilkins , R.J. 2003 . Comparison of grass and legume silages for milk production. 1. Production responses with different levels of concentrate . Journal of Dairy Science , 86 : 2598 – 2611 .
- Flynn , A.V. 1981 . Factors affecting the feeding value of silage . In W. Haresign & D. Lewis , Recent Advances in Animal Nutrition , pp. 81 – 89 . Buttersworths , London .
- Frame , J. and Laidlaw , A.S. 2011 . Improved Grassland Management , 2nd ed , Wiltshire : The Crowood Press Ltd .
- Green , B.H. 1990 . Agricultural intensification and the loss of habitat, species and amenity in British grasslands: A review of historical change and assessment of future prospects . Grass and Forage Science , 45 : 365 – 372 .
- Green , J.O. , Corrall , A.J. & Terry , R.A. 1971 . Relationships Between Stage of Growth, Yield and Forage ( Hurley, Berkshire: Grass species and varieties: Grassland Research Institute )
- Han , D. , O'Kiely , P. and Sun , D.W. 2003 . Linear models for the dry matter yield of the primary growth of a permanent grassland pasture . Irish Journal of Agriculture and Food Research , 42 : 17 – 38 .
- Hopkins , A. and Holz , B. 2006 . Grassland for agriculture and nature conservation: Production, quality and multi-functionality . Agronomy Research , 4 : 3 – 20 .
- Jancik , F. , Homolka , P. , Čermak , B. and Lad , F. 2008 . Determination of indigestible neutral detergent fibre contents of grasses and its prediction from chemical composition . Czech Journal of Animal Science , 53 : 128 – 135 .
- Jones , D.I.H. 1970a . The effect of nitrogen fertilizers on the ensiling characteristics of perennial ryegrass and cocksfoot . Journal of Agricultural Science , 75 : 517 – 521 .
- Jones , D.I.H. 1970b . Cell-wall constituents of some grass species and varieties . Journal of the Science of Food and Agriculture , 21 : 559 – 562 .
- Keady , T.W.J. , Mayne , C.S. and Fitzpatrick , D.A. 2000 . Prediction of silage feeding value from the analysis of the herbage at ensiling and effects of nitrogen fertilizer, date of harvest and additive treatment on grass silage composition . The Journal of Agricultural Science , 134 : 353 – 368 .
- Keating , T. and O'Kiely , P. 2000a . Comparison of old permanent grassland, lolium perenne and lolium multiflorum swards grown for silage: 4. Effects of varying harvesting date . Irish Journal of Agricultural and Food Research , 39 : 55 – 71 .
- Keating , T. and O'Kiely , P. 2000b . Comparison of old permanent grassland, lolium perenne and lolium multiflorum swards grown for silage: 3. Effects of varying fertiliser N application rate . Irish Journal of Agricultural and Food Research , 39 : 35 – 53 .
- Kromus , S. , Wachter , B. , Koschuh , W. , Mandl , M. , Krotscheck , C. and Narodoslawsky , M. 2004 . The green biorefinery Austria – development of an integrated system for green biomass utilization . Chemical and Biochemical Engineering Quarterly , 18 : 7 – 12 .
- Kunelius , H.T. , Macleod , L.B. and Calder , F.W. 1974 . Effects of cutting management on yields, digestibility, crude protein and persistence of timothy, bromegrass, and orchard grass . Canadian Journal of Plant Science , 54 : 55 – 64 .
- Kunelius , H.T. and Narasimhalu , P. 1983 . Yields and quality of Italian and Westerwolds ryegrasses, red clover, alfalfa, birdsfoot trefoil, and Persian clover grown in monocultures and ryegrass legume mixtures . Canadian Journal of Plant Science , 63 : 437 – 442 .
- Mason , W. and Lachance , L. 1983 . Effects of initial harvest date on dry matter yield, in vitro dry 20 matter digestibility and protein in timothy, tall fescue, reed canarygrass, and Kentucky 21 bluegrass . Canadian Journal of Plant Science , 6 : 675 – 685 .
- McGrath , D. 1992 . A note on the influence of nitrogen application and time of cutting on water soluble carbohydrate production by Italian ryegrass . Irish Journal of Agricultural and Food Research , 31 : 189 – 192 .
- Minson , D.J. 1990 . Forage in Ruminant Nutrition , Millbrae , CA : California Academic Press .
- Moore , K.J. , Moser , L.E. , Vogel , K.P. , Waller , S.S. , Johnson , B.E. and Pedersen , J.F. 1991 . Describing and quantifying growth stages of perennial forage grasses . Agronomy Journal , 83 : 1073 – 77 .
- Morrison , I.M. 1980 . Changes in the lignin and hemicellulose concentrations of ten varieties of temperate grasses with increasing maturity . Grass and Forage Science , 35 : 287 – 293 .
- Muck , R.E. , O'Kiely , P. and Wilson , R.K. 1991 . Buffering capacities in permanent pasture grasses . Irish Journal of Agricultural Research , 30 : 129 – 141 .
- Murphy , J.D. and Power , N.M. 2009 . An argument for using biomethane generated from grass as a biofuel in Ireland . Biomass and Bioenergy , 33 : 504 – 512 .
- Murphy , J.D. , Braun , R. , Weiland , P. & Wellinger , A. 2011 . Biogas from crop digestion. Task 37 – Energy from Biogas , ( Paris: IEA Bioenergy )
- Nowakowski , T.Z. 1962 . Effects of nitrogen fertilizers on total nitrogen, soluble nitrogen and soluble carbohydrate contents of grass . Journal of Agricultural Science , 59 : 387 – 392 .
- Ohlsson , C. and Wedin , W.F. 1989 . Phenological staging schemes for predicting red clover quality . Crop Science , 29 : 416 – 420 .
- O'Kiely , P. , O'Riordan , E.G. and Maloney , A.P. 1997 . Grass ensilability indices as affected by the form and rate of inorganic nitrogen fertiliser and the duration to harvesting . Irish Journal of Agricultural and Food Research , 36 : 93
- Peeters , A. , Parente , G. & Le Gall , A. 2006 . Temperate legumes: key-species for sustainable temperate mixtures . In Grassland Science in Europe, 11. Proceedings of the 21st General Meeting of the EGF: Sustainable Grassland Productivity , Badajoz , Spain , pp. 205 – 220 .
- Penning de Vries , F.W.T. , Wiltage , J.M. and Kramer , D. 1979 . Rates of respiration and of increase in structural DM in young wheat, ryegrass and maize plants in relation to temperature, water stress and their sugar content . Annals of Botany , 44 : 595 – 609 .
- Peyraud , J.L. , Le Gall , A. and Luscher , A. 2009 . Potential food production from forage legume-based-systems in Europe: An overview . Irish Journal of Agricultural and Food Research , 48 : 115 – 135 .
- Playne , M.J. and McDonald , P. 1966 . The buffering constituents of herbage and of silage . Journal of the Science of Food and Agriculture , 17 : 264 – 268 .
- Prochnow , A. , Heiermann , M. , Plöchl , M. , Linke , B. , Idler , C. , Amon , T. and Hobbs , P.J. 2009 . Bioenergy from permanent grassland – a review: 1. Biogas . Bioresource Technology , 100 : 4931 – 4944 .
- Reid , D. 1970 . The effects of a wide range of nitrogen application rates on the yields from a perennial ryegrass sward with a without white clover . Journal of Agricultural Science , 74 : 227 – 240 .
- Reid , D. 1985 . A comparison of the yield responses of four grasses to a wide range of nitrogen application rates . Journal of Agricultural Science , 105 : 381 – 387 .
- SAS 2004 . SAS for windows, Version 9.1.2 . ( Cary , NC : Statistical Analysis System, Institute Inc ).
- Smit , H.J. , Metzger , M.J. and Ewert , F. 2008 . Spatial distribution of grassland productivity and land use in Europe . Agricultural Systems , 98 : 208 – 219 .
- Stakelum , G. & Dillon , P. 1990 . Influence of sward structure and digestibility on the intake and performance of lactating and growing cattle . In C.S. Mayne Management Issues for the Grassland Farmer in the 1990's: Occasional Symposium no. 25 . British Grassland Society, Warwickshire, pp. 30 – 42 .
- Thomas , T.A. 1977 . An automated procedure for the determination of soluble carbohydrates in herbage . Journal of the Science of Food and Agriculture , 28 : 639 – 642 .
- Tilley , J.M.A. and Terry , R.A. 1963 . A two-stage technique for the in vitro digestion of forage crops . Journal of the British Grassland Society , 18 : 104 – 111 .
- Travers , G. 1999 . Nitrogen dynamics in grass and grass/clover swards grazed by beef cattle . PhD thesis, The Queens University of Belfast, Belfast .
- Tremblay , G.F. , Bélanger , G. and Drapeau , R. 2005 . Nitrogen fertilizer application and developmental stage affect silage quality of timothy (Phleum pratense l.) . Grass and Forage Science , 60 : 337 – 355 .
- Valk , H. , Kappers , I.E. and Tamminga , S. 1996 . In sacco degradation characteristics of organic matter, neutral detergent fibre and crude protein of fresh grass fertilized with different amounts of nitrogen . Animal Feed Science and Technology , 63 : 63 – 87 .
- Van Soest , P.J. 1963 . Use of detergents in the analysis of fibrous feeds. II. A rapid method for the determination of fibre and lignin . Journal – Association of Official Analytical Chemists , 46 : 829 – 835 .
- Van Soest , P.J. 1994 . Nutritional ecology of the ruminant , 2nd ed , Ithaca , NY : Cornell University Press .
- Weldon , B. & O'Kiely , P. 2011 . Red clover: A promising pasture legume for Ireland . In : Proceedings of the Irish Agricultural Research Forum . Tullamore , Ireland , 82 .
- Whitehead , D.C. 1995 . Grassland Nitrogen , UK : CAB INTERNATIONAL .
- Wilkins , P.W. and Humphreys , M.O. 2003 . Progress in breeding perennial forage grasses for temperate agriculture . The Journal of Agricultural Science , 40 : 129 – 150 .
- Wilman , D. and Wright , P.T. 1983 . Some effects of applied nitrogen on the growth and chemical composition of temperate grasses . Herbage Abstracts , 53 : 387 – 393 .
- Wilson , R.K. and Collins , D.P. 1980 . Chemical composition of silages made from different grass genera . Irish Journal of Agricultural Research , 19 : 75 – 84 .
Appendix I(1–3):
Regression equations for yield and chemical composition against grass growth stage (Moore et al., Citation1991), where stage 1.0–1.9 = vegetative-leaf development, 2.0–2.9 = elongation–stem elongation and 3.0–3.9 = reproductive-floral development. Quadratic equation (i.e., y = a + bx + cx2) where ‘a’ is the intercept and ‘b’ and ‘c’ are the linear and quadratic coefficients; Linear equation (i.e., y = a + bx) where ‘a’ is the intercept and ‘b’ is the linear coefficient (slope). R 2=Coefficient of determination (the variability of the dependent measure (sum of squares total) – the variability of the residuals in the model (sum of squares error)/ sum of squares total).