Abstract
Current understanding of plant nutrient stoichiometry mainly focus on C, N, and P and in humid and tropic regions. This study was conducted to investigate nutrient stoichiometry in a semaird small watershed. Three dominant plants, Medicago sativa, Stipa bungeana, and Artemisia sacrorum, sampled along a natural nutrient gradient, were analyzed with respect to plant nutrient stoichiometry (N:P, N:K, and K:P). The results showed that nutrient stoichiometry varied greatly between plants and within the same plant at the small watershed scale. At the natural nutrient gradient, N:P of the N limited plant is mainly determined by plant N, while that of P or P+N limited plants were dominated by plant P. The N:K and K:P of both N limited and P or N+P limited plants were mainly controlled by plant K with weak relations to nutrient limitations. Aboveground biomass directly influences nutrient stoichiometry, while extractable soil nutrients exert their impacts on stoichiometry through aboveground biomass.
Introduction
Plants need nutrients to meet their physiological requirements and develop their vital functions (Larcher, Citation1995). The availability of nutrients to plants often varies significantly in space and time at a variety of scales. However, tissue concentrations of major nutrients, such as N, P, and K, are usually maintained within much more restricted limits (Chapin et al., Citation1987). Therefore, plant nutrient status and stoichiometry have been widely used as indicators to assess nutrient limitation and availability (Koerselman & Meuleman, Citation1996; Venterink et al., Citation2002, Citation2003; Güsewell, Citation2004; Knecht & Göransson, Citation2004; Ellison Citation2006), plant growth (Ågren Citation2004, Citation2008; Niklas & Cobb, Citation2005; Niklas et al., Citation2005; Niklas Citation2006), ecosystem processes at different levels from cellular metabolism to ecosystem productivity (Sterner & Elser, Citation2002; Dodds et al., Citation2004), and even pollution impacts (Kirkham, Citation2001; Pitcairn et al., Citation2001; Bragazza et al., Citation2004; Skinner et al., Citation2006; Britton & Fisher, Citation2007). In addition, nutrient stoichiometry can provide important insights into processes that control patterns of species distributions, abundances, and population dynamics (Venterink et al., Citation2003; Moe et al., Citation2005; Watanabe et al., Citation2007; Ågren, Citation2008). Due to these important applications, nutrient stoichiometry has been well investigated in past decades. At the global scale, Reich and Oleksyn (Citation2004) reported an average N:P ratio of 13.8, while Elser et al. (Citation2000) estimated of a lower value of 12.7. Elser et al. (Citation2000) also summarized N:P ratios for terrestrial and freshwater autotrophs and found a range of 2.5–30 for all observations and a range of 7.5–15 for more than 50% of the observations. At the regional scale, nutrient stoichiometry varies greatly. Townsend et al. (Citation2007) found a wider foliar N:P range (7.5–43.5) than that of global plants for lowland tropical rain forest. Plant N:P in the Loess Plateau is 15.4 on the average but varies between 7 and 29 (Zheng & Shangguang, Citation2007); while the averages in China and Chinese grassland are 16.3 and 15.3, respectively (Han et al., Citation2005, Citation2008).
Differences in plant species as well as geographical and climatic factors can influence the variation of plant nutrient stoichiometry. The variations in nutrient stoichiometry within plant species have been well acknowledged. The N:P ratio changes greatly with plant functional groups and differs between N-fixing plants and non-N-fixing plants in the Chinese grassland (He et al., Citation2008) and in the Loess Plateau (Zheng & Shangguang, Citation2007). Results from Townsend et al. (Citation2007) in Costa Rica and Brazil showed that the dominant influence on foliar N:P ratios in the tropics is species. Niklas and Cobb (Citation2005) observed statistically significant N and P stoichiometric differences between trees and herbaceous species. With regard to geographical and climatic factors, Reich and Oleksyn (Citation2004) found that foliar N and P concentrations decline and N:P increase toward the equator as average temperature and growing season length increase. They suggest that leaf N and P increase from the warmer tropics to the cooler and drier mid-latitudes, and similarly N:P ratios increase with mean temperature. McGroddy et al. (Citation2004) and Kerkhoff et al. (Citation2005) also observed similar global patterns of foliar N:P. Ågren (Citation2004) suggested that climatic factors can cause both increases and declines in N:P ratio, depending on the current relative growth rate. However, the influence of geographical and climatic factors on nutrient stoichiometry differs with scale studied. Although significant correlations between geographical and climate factors and nutrient stoichiometry were observed at the large scales mentioned above, it seems not to occur at regional or smaller spatial scales. For example, Townsend et al. (Citation2007) observed no relationship between mean foliar N:P values and annual mean rainfall and latitude in tropical rain forests. He et al. (Citation2008) found that climatic variables (growing season temperature and precipitation) explained less than 3% of the total covariance in foliar N:P ratios, and had very little direct influence on foliar N:P ratios in Chinese grassland. Similarly, Zheng and Shangguang (Citation2007) also noted insignificant correlations between climate and leaf N:P ratios in the Chinese Loess Plateau. These observations suggest that plant species and plant growth, rather than geographical and climatic factors, are main drivers of nutrient stoichiometry at small spatial scales.
The previous studies were often conducted at large scales and plant species were taken as a whole regardless of marked differences in nutrient stoichiometry between plant individuals. Because of the spatial variation within a plant species, it is hard to select enough sites with the same plant species at large scales such that effects of plant species can be distinguished from effects of individuals. However, selecting enough sites is possible at a small scale, which offers solutions for studying stoichiometric variations within plants.
The N:P stoichiometry has been well investigated, both theoretically and experimentally, while the knowledge of stoichiometry of other elements is still lacking. As the second most abundant mineral in plants, K is an essential factor in protein synthesis, some enzymes activities, photosynthesis and plant growth, and osmotic mediation in cell expansion and turgor-driven movements (Marschner, Citation1995; Spalding et al., Citation1999). In addition, K is important for maintaining the turgor pressure in plants under drought and salinity and to resist other stress factors (Zhu et al., Citation1998; Hu & Schmidhalter, Citation2005). As a result, K stoichiometry should be further studied.
In this study, we investigated the N, P, and K stoichiometry of three dominant plants, Medicago sativa (MS), Stipa bungeana (SB), and Artemisia sacrorum (AS), in a semiarid small watershed with a natural nutrient gradient in plant species, aboveground biomass, plant and soil nutrient condition in order to test our hypothesis that at a small watershed scale, nutrient stoichiometry not only varies with plant species, but also changes with soil nutrient conditions as well as with plant aboveground biomass, with the latter reflecting plant growth rate.
Materials and methods
Study site and sampling
The study was carried out in the Liudaogou, a small watershed at the north part of the Loess Plateau, China (110°21′–110°23′E, 38°46′–38°51′N). The watershed has an area of 6.89 km2 and is at an altitude between 1 081 and 1 274 m above sea level. The region has a semiarid continental climate with an average annual temperature of 8.4 °C. Monthly mean temperatures range from−9.7 °C in January to 23.7 °C in July. The average annual precipitation is 437 mm and 77% occurs from June to September.
Three dominant plant species, MS, SB, and AS, belonging to Leguminosae, Gramineae, and Compositae, represent legumes, grass, and woody plants in the small watershed. In August 2007, we sampled MS (n=62), SB (n=53), and AS (n=55) randomly along a nutrient gradient in the watershed. This nutrient gradient is caused by the elevation, landscape and the distances from stream. We selected sites subject to minimal grazing and other anthropogenic disturbances. The soil for all sampling sites was Loessial soil, which belong to the major soil order of Regosols according to International Soil Taxonomy (FAO/ISRIC/ISSS, Citation1998). All sites had extensive vegetation cover dominated by MS, SB, or AS. At each site, all current-year leaves were collected in a 1m×1m plot. Soil samples from 0 to 20 cm depth were collected simultaneously with a 5-cm diameter tube auger. Plant samples were oven-dried at 105 °C for 30 min and then at 70 °C for at least 48 h to constant weight. Plant samples were ground with a plant-sample mill into uniformly fine powder and sieved with a 1-mm mesh screen before laboratory analysis. Soil samples were air dried and ground to pass through 1-mm nylon screens prior to laboratory analysis.
Laboratory analysis
For N, P, and K determination, the samples were digested in a solution of H2SO4-H2O2, and then the N concentrations were determined with the Kjeltec analyzer, the P concentrations were colorimetrically analyzed with blue phosphor-molybdate, and the K concentrations by flame photometry (Page et al., Citation1982). All the chemical determinations were repeated three times with the same subsamples. All concentrations will be expressed on a mass basis.
Soil extractable N () were analyzed with a Lachat Flow Analyzer (AutoAnalyzer3-AA3, Seal Analytical, Mequon, WI, USA) after extraction with potassium chloride (Kachurina et al., Citation2000). Extractable P was analyzed according to standard methods described by the Olsen method (Page et al., Citation1982). Extractable K was extracted with 1 mol L−1 neutral ammonium acetate and measured by atomic absorption spectrometry.
Statistical analyses
A one-way analysis of variance was performed to test significant differences in nutrient stoichiometry (N:P, N:K, and K:P) between plant species. Multivariate analysis of variance were used to determine the differences of N:P, N:K, and K:P ratios with aboveground biomass, soil extractable nutrients and plant nutrient concentrations with general linear models (GLM) and F tests. The percent of squares (SS) of each variable to the total sum of squares was used to explain the contributions of the variable to total variation. Correlation and linear regression analysis were used to test relationships between variables and reveal the influence of independent variables on dependent variables. However, the results of correlation and regression analyses offer an apparent influence that cannot be divided into direct and indirect influences, which may provide important information for explaining the relationship. Path analysis was, therefore, carried out to separate the influences into direct and indirect influence by calculating direct and indirect path coefficients. Path analysis is a statistical technique that partitions correlations into direct and indirect effects, and attempts to differentiate between correlation and causation. This technique also features multiple linear regressions and generates standardized partial regression coefficients (path coefficients) (Wright, Citation1934). Direct path coefficients measure the direct effect of an independent variable on a dependent variable, while indirect path coefficients specify the effect of an independent variable passed through other independent variables. A high path coefficient indicates a strong effect on the dependent variable. All the statistical analyses were conducted using SAS software (SAS Institute, Citation1999).
Results
General patterns of nutrient stoichiometry
Across the watershed and three species, the concentrations of N, P, and K were significantly correlated each other (,
,
, n=163, p<0.0001), indicating that all the species respond similarly to soil nutrient availability. The stoichiometry of the three plants varied between plant species and within a species (). The mean values of N:P, N:K, and K:P were similar in MS and SB. but N:P and N:K ratios were significantly higher and K:P was significantly lower in MS and SB than in AS. When tested within plant species, N:P showed small variation (coefficient of variation) and ranged from 10.1 to 20.8 for MS, from 9.8 to 21.0 for SB, and from 7.1 to 17.7 for AS, whereas K:P presents the largest variation and varied from 1.9 to 18.4 for MS, from 1.9 to 7.3 for SB, and from 5.1 to 13.8 for AS.
Table I. Nutrient concentrations and stoichiometry in the three studied plant species.
The limiting nutrient also differed also between different plant species. Venterink et al. (Citation2003) suggested N:P<14.5 and N:K<2.1 as levels for N limitation, N:P>14.5 and K:P>3.4 as P or P+N limitation, N:K>2.1 and K:P<3.4 as K or K+N limitation. Based on these thresholds the mean ratios of nutrients indicate that AS was N limited, while MS and SB were P or P+N limited. However, nutrient limitation varied greatly within plant species (). For MS, 2 out of 62 sample plots were N limited, 26 out of 62 were P or N+P limited, and 14 out of 62 were K or K+N limited; for SB 22 sample plots out of 53 were P or P+N limited and 10 out of 53 were K or K+N limited; for AS, 50 sample plots out of 55 were N limited and 4 out of 55 were P or P+N limited. The remaining sample plots for three plants were not limited by N, P, or K.
Figure 1. Diagram showing the relationship between nutrient stoichiometry in plants vs. the type of nutrient limitation based on the criteria of Venterink et al. (Citation2003).
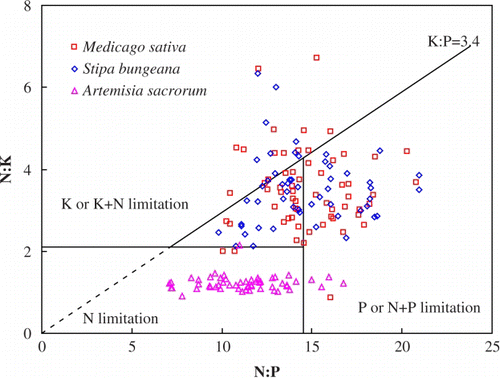
Relationships between nutrient stoichiometry and biomass, plant and soil nutrients
Plant N:P is determined by nutrient limitations. For the N limited plant, AS, plant N concentration explained most to the total covariance (40.9%), which was positively related with N:P at p<0.001. In addition, plant K and biomass explained 31.9 and 23.0% of the total covariance of N:P and were significantly correlated. For the P or P+N limited plants, MS and SB, plant P accounted for 87.1 and 64.2% of the total covariance and were negatively related with N:P in both plants. N:P of SB was also negatively related with soil extractable P, which accounted 19.5% of the total covariance ( and ).
Table II. The general linear models for the effect of aboveground biomass, plant (N, P, K) and soil (Ns, Ps, Ks) nutrients on stoichiometry.
Table III. Correlation coefficients between nutrient ratios and aboveground biomass, plant (N, P, K) and soil nutrient (Ns, Ps, Ks) concentrations.
K stoichiometry (N:K and K:P) was mainly determined by plant K concentration (Tables II and III). Plant K was negatively correlated with N:K for the three plants, contributing 74.1, 45.8, and 41.2% of the total covariance in MS, SB, and AS; whereas it was positively correlated with K:P and explained 72.2, 31.8, and 47.5% of the total covariance in corresponding plants, respectively. For the P or P+N limited plant SB, biomass also explained 33.7 and 30.1% of total covariance for N:K and K:P; for the N limited plant AS, biomass and soil extractable N explained 17.7 and 17.7% of N:K's covariances, while plant N explained 34.9% of K:P's covariance. Generally, effects of biomass and nutrients on stoichiometry followed the order of plant nutrients >biomass >soil nutrients.
Discussion
General patterns of nutrient stoichiometry
The N:P ratios of our observations fell in the range 2.5–30 for terrestrial plants (Elser et al., Citation2000) and 7–29 for the Loess Plateau plants (Zheng & Shangguang, Citation2007), respectively. The average N:P ratio of the three plant species was 13.5, which is lower than that observed in Chinese grassland biomes (15.3) by He et al. (Citation2008). The N:P of MS, SB, and AS was also lower than corresponding values for legumes (19.0), grasses (15.8), and woody plants (15.9) in the same study. Our average N:P ratio is also lower than that observed in the Loess Plateau, 15.4, (Zheng & Shangguang, Citation2007) and China, 16.3, (Han et al., Citation2005), respectively. However, our estimate is close to the global N:P of 13.8 reported by Reich and Oleksyn (Citation2004) and 12.7 as reported by Elser et al. (Citation2000), respectively.
In this study, mean values of soil extractable N and P are markedly lower than those in the Loess Plateau, Chinese grassland and China, resulting in lower plant N and P concentrations (). The effects of plant N are greater than those of plant P, resulting in smaller N:P ratios than those in the Loess Plateau, Chinese grassland and China. Although our plant N and P concentrations were lower than in the two global observations of plant N and P concentrations (), the differences of plant N (10 and 13%, respectively) are smaller than those of plant P (29 and 43%, respectively), leading to similar or higher N:P than at a global scale.
Currently, there is little research about plant N:K and K:P. We have only found values for the Loess Plateau (Zheng & Shangguang, Citation2007) and Vermont, USA (Wakefield et al., Citation2005). Although N:K and K:P ratios were not available for the Loess Plateau, we can calculate from the overall N:K and K:P of 1.4 and 10.4, respectively, of which N:K was lower and K:P was higher than our observations. Our N:K is 44% lower and K:P is 94% higher than what Wakefield et al. (Citation2005) observed in Sarracenia purpurea due to its lower plant K but similar plant N and P concentrations. In a review of carnivorous plants, Ellison (Citation2006) calculated average N:P, N:K, and K:P ratios of 18.0, 1.0, and 18.6, respectively, for seven plant species. The rather large differences between these estimates and our observations might be a result of the differences in nutrient acquisition strategies.
Table IV. Comparison of plant nutrient concentrations in some studies.
Among the three plants, AS had high N and very high P and K concentrations in plant, MS had a high N and low K concentrations, while SB had the lowest concentration of these elements (). Therefore, MS and SB had similar nutrient ratios, but AS had smaller mean N:P and N:K and greater mean K:P ratio than MS and SB. The similar N:P ratio between MS and SB is not consistent with observations in which legumes had significantly higher N:P ratios in Chinese grassland, where the N:P of legumes is about 28% higher than that of non-legumes (He et al., Citation2008). Legumes do have higher plant N concentrations than other plants both in our results and in others (Crews, Citation1999; Vitousek & Field, Citation1999; Martinelli et al., Citation2000; Townsend et al., Citation2007; He et al., Citation2008). However, the higher N concentrations in legumes do not necessarily lead to predictable differences in canopy N:P values (Townsend et al., Citation2007).
Main factors shaping nutrient stoichiometry
At the global scale, nutrient stoichiometry seems to be affected mainly by climate, which dominates the growth of plant and nutrient uptake (Ågren, Citation2008), while Townsend et al. (Citation2007) suggest that local-scale species diversity, rather than environmental factors, may exert the strongest control on variation in foliar nutrient stoichiometry at the smaller scale of a tropical forests. Other recent research also shows that climatic and geographical features (altitude and latitude) have weak or no relationship with plant stoichiometry in specific regions (Han et al., Citation2005; Zheng & Shangguang, Citation2007; He et al., Citation2008). Therefore, for a given plant species, nutrient stoichiometry is largely influenced by plant growth and nutrient availability at the local (watershed) scale where climate and geophysical factors change slightly.
A “dilution” of nutrient contents as plants grow has been well recognized both intra- and inter-specifically (Ohnmeiss & Baldwin, Citation1994; McJannet et al., Citation1995; Lutze & Gifford, Citation1998). This is clearly observed in our study (). N, P, and K concentrations declined with increasing aboveground biomass in all the three plant species. However, the strength of the dilution varied with species and element. The dilution was weakest, and perhaps even absent for all elements with SB, whereas AS showed the strongest dilution for N and K and MS for P. In addition, the decrease of these elements for MS and AS followed the order of N>K>P, while that for SB followed the order of K>N>P, as reflected by the slopes of the regression equations in .
Unlike nutrient concentrations in plants, N:K were positively related with aboveground biomass and K:P were negatively related with it (), while N:P were not related with it for all species. Niklas et al. (Citation2005) found that the N:P ratio decreases with growth rates. This pattern would be consistent with our results for N:P in SB and AS based on the assumption that aboveground biomass reflects the growth rate because we sampled plants at the same time. The linear relationship between leaf growth rate and leaf mass observed by Niklas et al. (Citation2005) support our assumption.
The relations between plant stoichiometry and soil nutrients are not consistent in the present study. Orgeas et al. (Citation2002) found correlations between nutrient stoichiometry and soil N under field conditions. Shaver and Lechowicz (Citation1985) and Wookey et al. (Citation1995) observed such correlations under experimental conditions. Méndez and Karlsson (Citation2005) also reported that nutrient concentrations were positively related with soil N in Pinguicula vulgaris. However, Bowman et al. (Citation2003) failed to find significant relationships between soil and plant stoichiometry in an alpine ecosystem as did Güsewell and Koerselman (Citation2002) in wetlands.
Although we determined only weak correlations between soil and plant nutrient concentrations [the correlation coefficients between plant and soil N is 0.011 (p=0.885), between plant and soil P is−0.089 (p=0.260), between plant and soil K is−0.159 (p=0.042), n=170], soil extractable nutrients were significantly correlated with the nutrients accumulated in plants (), which is determined by both aboveground biomass and plant nutrients concentrations and due to the significant correlations between soil extractable nutrients and biomass (). The results in show that aboveground biomass has the largest direct path coefficient and large indirect path coefficient from soil extractable nutrients to plant accumulated nutrients, suggesting that the observed correlations between soil extractable nutrients and accumulated nutrients in plants are driven by the aboveground biomass. In addition, path analysis of aboveground biomass and soil extractable nutrients to nutrient stoichiometry also shows that the correlation between soil extractable nutrients and plant nutrient stoichiometry is linked by aboveground biomass because aboveground biomass has large direct and indirect path coefficients to them ().
Table V. Path coefficients of soil nutrient and biomass to nutrients accumulated in plants.
Table VI. Path coefficients of soil extractable nutrients to aboveground biomass.
Table VII. Path coefficients of aboveground biomass and soil extractable nutrients to nutrient stoichiometry.
At the small (watershed) scale, plant nutrient stoichiometry varied greatly both between plant species and within the species along natural nutrient gradients. N:P of the N limited plant is mainly determined by plant N, while that of P or P+N limited plants were dominated by plant P. N:K and K:P of both N limited and P or N+P limited plants were mainly controlled by plant K and less related with nutrient limitations. Aboveground biomass directly influenced nutrient stoichiometry, while extractable soil nutrients exert their impacts on stoichiometry through aboveground biomass. At the small watershed scale in the semiarid region, the availability of soil nutrient varied greatly with sites, therefore, the difference in the factors influencing stoichiometry at the site level and watershed level should be further separated so as to establish a more general pattern of nutrient stoichiometry in semiarid watershed.
Acknowledgements
The authors thank Professor Göran Ågren from the Department of Ecology, Swedish University of Agricultural Sciences, and reviewers for their comments and suggestions that improved the quality of this paper. This study was supported by the National Natural Science Foundation of China (40901145, 41271315, 40801111), Science and Technology Development Research Program in Shaanxi Province (2011kjxx25), the Program from Northwest A&F University and from the Institute of Soil and Water Conservation, CAS & MWR.
References
- Ågren , G. I. 2004 . The C:N:P stoichiometry of autotrophs – Theory and observations . Ecology Letters , 7 : 185 – 191 . doi: 10.1111/j.1461-0248.2004.00567.x
- Ågren , G. I. 2008 . Stoichiometry and nutrition of plant growth in natural communities . Annual Review of Ecology, Evolution, and Systematics , 39 : 153 – 170 . doi: 10.1146/annurev.ecolsys.39.110707.173515
- Bowman , W. D. , Bahnj , L. and Damm , M. 2003 . Apine landscape variation in foliar nitrogen and phosphorus concentrations and the relation to soil nitrogen and phosphorus availability . Arctic Antarctic and Alpine Research , 35 : 144 – 149 . doi: 10.1657/1523-0430(2003)035[0144:ALVIFN]2.0.CO;2
- Bragazza , L. , Tahvanainen , T. , Kutnar , L. , Rydin , H. , Limpens , J. , Hájek , M. , Grosvernier , P. , Hájek , T. , Hajkova , P. , Hansen , I. , Iacumin , P. and Gerdol , R. 2004 . Nutritional constraints in ombrotrophic Sphagnum plants under increasing atmospheric nitrogen deposition in Europe . New Phytologist , 163 : 609 – 616 . doi: 10.1111/j.1469-8137.2004.01154.x
- Britton , A. and Fisher , J. 2007 . N P stoichiometry of low-alpine heathland: Usefulness for bio-monitoring and prediction of pollution impacts . Biological Conservation , 138 : 100 – 108 . doi: 10.1016/j.biocon.2007.04.006
- Chapin , F. S. , Bloom , A. J. , Field , C. B. and Waring , R. H. 1987 . Plant responses to multiple environmental factors . BioScience , 37 : 49 – 57 . doi: 10.2307/1310177
- Crews , T. E. 1999 . The presence of nitrogen fixing legumes in terrestrial communities: Evolutionary vs. ecological considerations . Biogeochemistry , 46 : 233 – 246 .
- Dodds , W. K. , Marti , E. , Tank , J. L. , Pontius , J. , Hamilton , S. K. , Grimm , N. B. , Bowden , W. B. , McDowell , W. H. , Peterson , B. J. , Valett , H. M. , Webster , J. R. and Gregory , S. 2004 . Carbon and nitrogen stoichiometry and nitrogen cycling rates in streams . Oecologia , 140 : 458 – 467 . doi: 10.1007/s00442-004-1599-y
- Ellison , A. M. 2006 . Nutrient limitation and stoichiometry of Carnivorous plants . Plant Biology , 8 : 740 – 747 . doi: 10.1055/s-2006-923956
- Elser , J. J. , Fagan , W. F. , Denno , R. F. , Dobberfuhl , D. A. , Folarin , A. , Huberty , A. , Interlandi , S. , Kilham , S. S. , McCauley , E. , Schulz , K. L. , Siemann , E. H. and Sterner , R. W. 2000 . Nutritional constraints in terrestrial and freshwater food webs . Nature , 408 : 578 – 580 . doi: 10.1038/35046058
- FAO/ISRIC/ISSS . 1998 . World Reference Base for Soil Resources ( Rome : World Soil Resources Reports ).
- Güsewell , S. 2004 . N:P ratios in terrestrial plants: Variation and functional significance . New Phytologist , 164 : 243 – 266 . doi: 10.1111/j.1469-8137.2004.01192.x
- Güsewell , S. and Koerselman , W. 2002 . Variation in nitrogen and phosphorus concentrations of wetland plants . Perspectives in Plant Ecology, Evolution and Systematics , 5 : 37 – 61 . doi: 10.1078/1433-8319-0000022
- Han , W. , Fang , J. , Guo , D. and Zhang , Y. 2005 . Leaf nitrogen and phosphorus stoichiometry across 753 terrestrial plant species in China . New Phytologist , 168 : 377 – 385 . doi: 10.1111/j.1469-8137.2005.01530.x
- He , J. S. , Wang , L. , Flynn , D. F. B. , Wang , X. P. , Ma , W. H. and Fang , J. Y. 2008 . Leaf nitrogen:phosphorus stoichiometry across Chinese grassland biomes . Oecologia , 155 : 301 – 310 . doi: 10.1007/s00442-007-0912-y
- Hu , Y. and Schmidhalter , U. 2005 . Drought and salinity: A comparison of their effects on mineral nutrition of plants . Journal of Plant Nutrition and Soil Science , 168 : 541 – 549 . doi: 10.1002/jpln.200420516
- Kachurina , O. M. , Zhang , H. , Raun , W. R. and Krenzer , E. G. 2000 . Simultaneous determination of soil aluminum, ammonium- and nitrate-nitrogen using 1 M potassium chloride extraction . Communications in Soil Science and Plant Analysis , 31 : 893 – 903 . doi: 10.1080/00103620009370485
- Kerkhoff , A. J. , Enquist , B. J. , Elser , J. J. and Fagan , W. F. 2005 . Plant allometry, stoichiometry and the temperature-dependence of primary productivity . Global Ecology and Biogeography , 14 : 585 – 598 . doi: 10.1111/j.1466-822X.2005.00187.x
- Kirkham , F. W. 2001 . Nitrogen uptake and nutrient limitation in six hill moorland species in relation to atmospheric nitrogen deposition in England and Wales . Journal of Ecology , 89 : 1041 – 1053 . doi: 10.1111/j.1365-2745.2001.00626.x
- Knecht , M. F. and Göransson , A. 2004 . Terrestrial plants require nutrients in similar proportions . Tree Physiology , 24 : 447 – 460 . doi: 10.1093/treephys/24.4.447
- Koerselman , W. and Meuleman , A. F. M. 1996 . The vegetation N:P ratio: A new tool to detect the nature of nutrient limitation . Journal of Applied Ecology , 33 : 1441 – 1450 . doi: 10.2307/2404783
- Larcher , W. 1995 . Physiological Plant Ecology: Ecophysiology and Stress Physiology of Functional Groups , 3rd ed. , Berlin , , Germany : Springer-Verlag .
- Lutze , J. L. and Gifford , R. M. 1998 . Acquisition and allocation of carbon and nitrogen by Danthonia richardsonii in response to restricted nitrogen supply and CO2 enrichment . Plant and Cell Environment , 21 : 1133 – 1141 . doi: 10.1046/j.1365-3040.1998.00343.x
- Marschner , H. 1995 . Mineral Nutrition of Higher Plants , London : Academic Press .
- Martinelli , L. A. , Almeida , S. , Brown , I. F. , Moreira , M. Z. , Victoria , R. L. , Filoso , S. , Ferreira , C. A. C. and Thomas , W. W. 2000 . Variation in nutrient distribution and potential nutrient losses by selective logging in a humid tropical forest of Rondonia, Brazil . Biotropica , 32 : 597 – 613 . doi: 10.1646/0006-3606(2000)032[0597:VINDAP]2.0.CO;2
- McGroddy , M. E. , Daufresne , T. and Hedin , L. O. 2004 . Scaling of C:N:P stoichiometry in forests worldwide: Implications of terrestrial Redfield-type ratios . Ecology , 85 : 2390 – 2401 . doi: 10.1890/03-0351
- McJannet , C. L. , Keddy , P. A. and Pick , F. R. 1995 . Nitrogen and phosphorus tissue concentrations in 41 wetland plants: A comparison across habitats and functional groups . Functional Ecology , 9 : 231 – 238 . doi: 10.2307/2390569
- Méndez , M. and Karlsson , P. S. 2005 . Nutrient stoichiometry in Pinguicula vulgaris: Nutrient availability, plant size, and reproductive status . Ecology , 86 : 982 – 991 . doi: 10.1890/04-0354
- Moe , S. J. , Stelzer , R. S. , Forman , M. R. , Harpole , W. S. , Daufresne , T. and Yoshida , T. 2005 . Recent advances in ecological stoichiometry: Insights for population and community ecology . Oikos , 109 : 29 – 39 . doi: 10.1111/j.0030-1299.2005.14056.x
- Niklas , K. J. 2006 . Plant allometry, leaf nitrogen and phosphorus stoichiometry, and interspecific trends in annual growth rates . Annals of Botany , 97 : 155 – 163 . doi: 10.1093/aob/mcj021
- Niklas , K. J. and Cobb , E. D. 2005 . N, P, and C stoichiometry of Eranthis hyemalis (Ranunculaceae) and the allometry of plant growth . American Journal of Botany , 92 : 1256 – 1263 . doi: 10.3732/ajb.92.8.1256
- Niklas , K. J. , Owens , T. , Reich , P. B. and Cobb , E. D. 2005 . Nitrogen/phosphorus leaf stoichiometry and the scaling of plant growth . Ecology Letters , 8 : 636 – 642 . doi: 10.1111/j.1461-0248.2005.00759.x
- Ohnmeiss , T. E. and Baldwin , I. T. 1994 . The allometry of nitrogen allocation to growth and an inducible defense under nitrogen-limited growth . Ecology , 75 : 995 – 1002 . doi: 10.2307/1939423
- Orgeas , J. , Ourcival , J. M. and Bonin , G. 2002 . Seasonal and spatial patterns of foliar nutrients in cork oak (Quercus suber L.) growing on siliceous soils in Provence (France) . Plant Ecology , 164 : 201 – 211 . doi: 10.1023/A:1021278421821
- Page , A. L. , Miller , R. H. and Kenney , D. R. 1982 . Methods of Soil Analysis Part 2. Agronomy Monographs , Vol. 9 , Madison , WI : American Society of Agronomy .
- Pitcairn , C. E. R. , Leith , I. D. , Fowler , D. , Hargreaves , K. J. , Moghaddam , M. , Kennedy , V. H. and Granat , L. 2001 . Foliar nitrogen as an indicator of nitrogen deposition and critical loads exceedance on a European Scale . Water, Air, and Soil Pollution , 130 : 1037 – 1042 . doi: 10.1023/A:1013908312369
- Reich , P. B. and Oleksyn , J. 2004 . Global patterns of plant leaf N and P in relation to temperature and latitude . Proceedings of the National Academy of Sciences USA , 101 : 11001 – 11006 . doi: 10.1073/pnas.0403588101
- SAS Institute Inc . 1999 . SAS User's Guide . Version 8 ( Cary , NC : SAS Institute, Inc .).
- Shaver , G. R. and Lechowicz , M. J. 1985 . A multivariate approach to plant mineral nutrition: Dose–response relationships and nutrient dominance in factorial experiments . Canada Journal of Botany , 63 : 2138 – 2143 . doi: 10.1139/b85-302
- Skinner , R. A. , Ineson , P. , Jones , H. , Sleep , D. , Leith , I. D. and Sheppard , L. J. 2006 . Heathland vegetation as a bio-monitor for nitrogen deposition and source attribution using δ15N values . Atmospheric Environment , 40 : 498 – 507 . doi: 10.1016/j.atmosenv.2005.09.054
- Spalding , E. P. , Hirsch , R. E. , Lewis , D. R. , Qi , Z. , Sussman , M. R. and Lewis , B. D. 1999 . Potassium uptake supporting plant growth in the absence of AKT1 channel activity: Inhibition by ammonium and stimulation by sodium . Journal of General Physiology , 113 : 909 – 918 . doi: 10.1085/jgp.113.6.909
- Sterner , R. W. and Elser , J. J. 2002 . Ecological Stoichiometry: The Biology of Elements from Molecules to the Biosphere , Princeton , NJ : Princeton University Press .
- Townsend , A. R. , Cleveland , C. C. , Asner , G. P. and Bustamante , M. M. C. 2007 . Controls over foliar N:P ratios in tropical rain forests . Ecology , 88 : 107 – 118 . doi: 10.1890/0012-9658(2007)88[107:COFNRI]2.0.CO;2
- Venterink , H. O. , Pieterse , N. M. , Belgers , J. D. M. , Wassen , M. J. and De Ruiter , P. C. 2002 . N, P, and K budgets along nutrient availability and productivity gradients in wetlands . Ecology Application , 12 : 1010 – 1026 . doi: 10.1890/1051-0761(2002)012[1010:NPAKBA]2.0.CO;2
- Venterink , H. O. , Wassen , M. J. , Verkroost , A. W. M. and De Ruiter , P. C. 2003 . Species richness–productivity patterns differ between N-, P-, and K-limited wetlands . Ecology , 84 : 2191 – 2199 . doi: 10.1890/01-0639
- Vitousek , P. M. and Field , C. B. 1999 . Ecosystem constraints to symbiotic nitrogen fixers: A simple model and its implications . Biogeochemistry , 46 : 179 – 202 .
- Wakefield , A. E. , Gotelli , N. J. , Wittman , S. E. and Ellison , A. M. 2005 . Prey addition alters nutrient stoichiometry of the carnivorous plant Sarracenia purpurea . Ecology , 86 : 1737 – 1743 . doi: 10.1890/04-1673
- Watanabe , T. , Broadley , M. R. , Jansen , S. , White , P. J. , Takada , J. , Satake , K. , Takamatsu , T. , Tuah , S. J. and Osaki , M. 2007 . Evolutionary control of leaf element composition in plants . New Phytologist , 174 : 516 – 523 . doi: 10.1111/j.1469-8137.2007.02078.x
- Wookey , P. A. , Robinson , C. H. , Parsons , A. N. , Welker , J. M. , Press , M. C. , Callaghan , T. V. and Lee , J. A. 1995 . Environmental constraints on the growth, photosynthesis and reproductive development of Dryas octopetala at a high Arctic polar semi-desert, Svalbard . Oecologia , 102 : 478 – 489 . doi: 10.1007/BF00341360
- Wright , S. 1934 . The method of path coefficients . Annals of Mathematical Statistics , 5 : 161 – 215 . doi: 10.1214/aoms/1177732676
- Zheng , S. and Shangguang , Z. 2007 . Spatial patterns of leaf nutrient traits of the plants in the Loess Plateau of China . Trees , 21 : 357 – 370 . doi: 10.1007/s00468-007-0129-z
- Zhu , J. , Liu , J. and Xiong , L. 1998 . Genetic analysis of salt tolerance in Arabidopsis: Evidence for a critical role of potassium nutrition . Plant Cell , 10 : 1181 – 1191 .