Abstract
Given the numerous environmental problems caused by chemical fertilizer overuse, agricultural practices are shifting toward the development of environmentally friendly nitrogen (N) fertilizers. In this study, a pot experiment was conducted to investigate the effect of poly(γ-glutamic acid) (γ-PGA), an effective fertilizer synergist, on rapeseed (Brassica napus cv. Qinyou No. 9) productivity, soil N pools, and soil enzymes. Seven treatments were implemented: T1, a check without urea; T2, a check with urea only; T3, urea mixed with glutamic acid; T4, urea mixed with 3 mg of γ-PGA per kilogram of soil; T5, urea mixed with 10 mg of γ-PGA per kilogram of soil; T6, urea coated with γ-PGA (0.9%, m/m); and T7, urea coated with γ-PGA (3.1%, m/m). The results demonstrated that the application of γ-PGA increased the above-ground fresh weight of rapeseed by 7.57–9.26% and the grain yield per pot by 6.46–10.98% compared with T2. The rapeseed grain yield significantly increased from 15.94 ± 0.55 g pot−1 in T2 to 17.69 ± 0.78 g pot−1 in T7. With the increase in pod numbers and seed numbers per pod, the seed yield was significantly improved after γ-PGA application. Culturable soil microbial count and microbial diversity were increased by γ-PGA application. In addition, the effects of application method of γ-PGA have a more important role on the soil microflora than its amount. The reduced loss of N can be attributed to the capacity of γ-PGA to increase the “ turnover pool.” More mineral N in the soil was immobilized by clays, soil microbes, and γ-PGA at the early growth stage, and the immobilized N was then released at the later growth stage for plant growth. Moreover, urease activity also increased with γ-PGA application. These findings indicated that the urea coated with γ-PGA could be a better choice for agricultural applications.
Introduction
Fertilizers have a vital role in the sustainable development of agriculture. However, the fertilizer nitrogen (N) uptake efficiency by grain crops is usually less than 50% (Chen & Mi Citation2012). The extensive application of chemical fertilizers in farmlands to increase crop productivity has resulted in an adverse impact on the ecology of agricultural systems and even reduced the nutritional quality of the crops (Wang et al. Citation2011). With the increasing public concern on human health and environmental protection, people prefer to use the N fertilizers that are environment friendly, possess high N utilization efficiency, and good for crop production. Several strategies have been used to reduce N loss, such as the application of inhibitors or coated fertilizers (Dahlin & Marstorp Citation2012; Han et al. Citation2013). However, these fertilizer technologies have a number of disadvantages, for example, controlled release fertilizers are considered too expensive for use in grain and oilseed production (Lammel Citation2005). Thus, an environment-friendly fertilizer synergist is required. Poly(γ-glutamic acid), or γ-PGA, is an anionic polyamide that consists of D- and L-glutamic acid units connected by γ-amide linkages produced by microbes. Given its biodegradable, nontoxic (Shih et al. Citation2001), and more excellent environmental characteristics compared with thermal polyaspartate and other polyamino acids, γ-PGA has attracted significant attention for its potential application as an environment-friendly fertilizer synergist (Chen et al. Citation2005; King et al. Citation2011).
Soil microbial biomass, the living part of the soil organic matter, has an important role in soil fertility, both as a source and as a sink for mineral nutrients (Huang & Song Citation2010; Chen et al. Citation2013). On one hand, microbes can optimize the energy provided by C sources to increase the N immobilization (Dinnes et al. Citation2002). On the other hand, the decomposition of microbes can increase the availability of N (Körner & Arnone Citation1992). Soil enzyme activities can indicate soil quality because of their rapid response to the change in soil management and their intimate relationship with soil biology (Kalambukattu et al. Citation2013). Ammonium () can also be fixed by clay minerals, especially the 2:1 clay minerals.
fixation and release have a crucial role in N-use efficiency (Juang et al. Citation2001). Previous studies have shown that N-use efficiency and plant growth can be increased by γ-PGA (Wang et al. Citation2008; Xu et al. Citation2011) and other polyamino acids (Kinnersley et al. Citation1994; Koskan et al. Citation1998; Funk Citation2009). However, little is known about the effects of γ-PGA on the diversity of soil microbes as well as its role in soil fertility. Thus, the diversity of soil microbes and the changes in the content of different forms of nitrogen after γ-PGA application should be determined to better understand the mechanism of γ-PGA.
In the present study, we report the full investigation on the effect of γ-PGA on microbial diversity and nitrogen pools to better understand the mechanism of γ-PGA application on microbial diversity, as well as its role in soil fertility. The effect of γ-PGA on rapeseed productivity, soil microbes, and nitrogen pools were studied. The microbial diversity and content of different forms of N in the soil were also compared. The mechanism of the increased N-use efficiency by γ-PGA was explained based on the research findings with a thematic diagram.
Materials and methods
Pot experiment
The pot experiment was established in a greenhouse of the College of Food Science and Light Industry, Nanjing University of Technology (118°38′30″ E, 32°04′42″ N), Nanjing, China. Rapeseed (Brassica napus cv. Qinyou No. 9) was transplanted in plastic pots (28 cm diameter × 30 cm high) from 10 November 2011 to 3 May 2012. The greenhouse utilized natural light. The soil was a Fluvisol with 11.8% sand, 68.1% silt, and 20.1% clay (determined by pipette method), which was air dried, ground, and sieved through a 1-cm sieve. The characteristics of the experimental soil were as follows: pH 6.65 (1:5 soil/water ratio); organic matter, 12.13 g kg−1 soil; alkaline hydrolysis N, 94.6 mg kg−1 soil; Olsen P, 21.6 mg kg−1 soil; available K, 91.3 mg kg−1 soil; and fixed ammonium N (-N), 101.5 mg kg−1 soil.
Experimental design
Two rapeseed seedlings were transplanted to each pot at the three-leaf stage. The pot experiment consisted of seven treatments involving γ-PGA. Each treatment was replicated in 15 pots, with 3 pots for each plant and soil sampling, and each pot contained 7 kg of experimental soil. The fertilizer treatments were as follows: T1, a check without urea; T2, a check with urea only; T3, urea mixed with 3 mg of glutamic acid per kilogram of soil; T4, urea mixed with 3 mg of γ-PGA per kilogram of soil; T5, urea mixed with 10 mg of γ-PGA per kilogram of soil; T6, urea coated with γ-PGA (PGAU1); and T7, PGAU2. The γ-PGA dose was selected according to the results of our previous experiment (different amounts of γ-PGA were applied in the soil for rapeseed growth, with the levels of γ-PGA being 0, 1, 3, 10, 20, and 50 mg per kilogram soil, respectively. Chlorophyll contents, total biomass, and grain yield were analyzed. The results showed that 3 or 10 mg of γ-PGA per kilogram of soil significantly affected plant growth). The dose of glutamic acid, a unit of γ-PGA, was selected equal to the low amount of γ-PGA. PGAU1 and PGAU2 were both urea coated with purified γ-PGA, with the addition levels of γ-PGA in PGAU1 and PGAU2 being 0.9% and 3.1%, respectively. PGAU1 and PGAU2 were prepared by turning on the blower power of the coating machine, and then adjusting the heating power to 500 W. The γ-PGA concentration was adjusted to 40 g/l, and the rotation speed of the coating machine was set to 40 rpm. Two kilograms of urea was coated with 50 ml of γ-PGA using a BY-400 coating machine, consequently yielding PGAU1. Similarly, 2 kg of urea was coated with 250 ml of γ-PGA using the coating machine, resulting in PGAU2. The coated urea was stored in a desiccator for use after the coating. Urea was then added to each pot as the N fertilizer at a rate of 150.00 mg N per kilogram of soil before transplantation. Superphosphate and potassium sulfate were applied in each treatment at the rates of 100.00 mg P2O5 and 150.00 mg K2O kg−1 soil, respectively. All pots were arranged weekly in a randomized manner during the experiment. All chemical reagents used in the experiment were of analytical grade, and deionized water was used throughout the experiment.
At the early seedling stage (10 December 2011), late seedling stage (10 January 2012), bud stage (8 February 2012), blooming stage (12 March 2012), and maturity stage (3 May 2012), the plants in each pot were harvested at ground level. The below-ground parts of the rapeseed were carefully removed from the soil and its fresh weight was determined after removing the substrate on the root surface. Each soil sample was separated into two parts. One part was sieved through a 2.00-mm sieve and stored at 4°C for microbial quantity, urease activity, and content of different forms of nitrogen analysis, whereas the other part was frozen (−20°C) for denaturing gradient gel electrophoresis (DGGE) analysis.
The chlorophyll contents and biomass of the potted rapeseed were determined at the late seedling stage, whereas the grain yield, total biomass per pot, and yield components including pod, seeds, and 1000-seed weight were determined at maturity.
Soil and plant sampling analysis
Number of pods per pot and seeds per pod were counted from 50 randomly selected pods after hand threshing. The weight of the 500 seeds was obtained to determine the 1000-grain weight. Chlorophyll content (Chl) was measured with a chlorophyll meter (CCM-200, OptiSciences, Inc.).
Culturable soil bacteria, fungi, and actinomycetes were determined by counting the colony-forming units on agar plates by dilution plating methods (El-Tarabily et al. Citation1996). Five grams of soil sample was suspended in 50 ml of sterile water, and the suspension was then 10-fold serially diluted. The culture media for bacteria, actinomycetes, and fungi were beef-extract peptone medium, Cause's No. 1 synthetic medium, and Rose Bengal medium, respectively.
The soil samples of each time intervals were used to extract soil total DNA. Soil DNA was extracted from 0.5 g of soil using the Ultra Clean Soil DNA isolation Kit (MoBio Loker Ave West, Carlsbad, CA, USA), according to the instructions of the manufacturer. The extracts were characterized by electrophoresis on 1.5% agarose gels, and the DNA was visualized by UV transillumination (Gel Doc2000, BioRad, Hercules, CA, USA). All the DNAs were stored at −20°C before further analysis. Polymerase chain reactions (PCRs) were performed using an Eppendorf C1000 Touch Thermal Cycler (Eppendorf, Hamburg, Germany). Approximately 50 µl of the reaction mixtures consisted of 5 µl of 10× PCR buffer (Mg2 + -free), 4 µl of 2.5 mM deoxyribonucleotide triphosphates, 0.5 µl of Taq polymerase (5 U µl−1, Takara Biotechnology, Japan), 1 µl of each primer at 50 mM, and 1 µl of DNA template (10–15 ng). The products were characterized by electrophoresis on 1.5% agarose gels. The PCR-DGGE conditions and primer sequences are shown in (Liu et al. Citation2012).
Table 1. PCR-DGGE conditions.
The DGGE gels were run for 12 h at 100 V in a 1× TAE buffer at a constant temperature of 60°C. The gels were then stained for 20 min in 150 ml 1× TAE buffer containing 100 ng ml−1 EB. Visualization and digital photography were performed as described earlier for agarose gels.
Ten grams of soil was extracted with 50.00 ml KCl (2.00 M) by shaking for 1 h to determine the -N and
-N contents. The filtered soil extracts were analyzed using a Lachat flow injection analyzer (Henriksen & Selmer-Olsen Citation1970). Soil microbial biomass nitrogen (SMBN) was determined by the chloroform-fumigation-extraction method (Wu Citation2006). Briefly, 40 g of soil was fumigated with CH3OH-free CHCl3 for 24 h at 25°C (unfumigated soil as the control). After the removal of the CHCl3 vapor, the fumigated and unfumigated soil samples were extracted with 0.5 M K2SO4 solution. The total soluble N in the filtrate was oxidized with alkaline persulfate oxidation, and then measured by dual-wavelength ultraviolet spectrophotometer. SMBN was calculated as the difference in the N values of the filtrates between the fumigated and unfumigated soil. The K
N factor for SMBN was 0.45 (Wu Citation2006). Fixed
was determined using the Silva and Bremner method (Citation1996), whereas urease activity was determined by the method described by Guan (Citation1986).
Statistical analysis
Analysis of variance (ANOVA) was used to test the differences in each parameter among the different treatments. Statistical analysis was performed by one-way ANOVA, followed by Duncan's test (P < 0.05). All statistical procedures were conducted using SPSS 17.0 for Windows. The results of the DGGE profiles were analyzed using the Gelcompar II software (Applied Maths, Austin, TX, USA). Shannon index (H′) was calculated from the DGGE profiles using the following formula:
Results
Effects of γ-PGA on the biological characteristics of potted rapeseed at the late seedling and maturity stages
The chlorophyll content and fresh weight of the underground and above-ground per pot were increased after application of γ-PGA (). Compared with T2, the plants treated with γ-PGA showed an increase of 4.71–16.04% in the chlorophyll content of rapeseed. The underground fresh weight per pot was increased by 15.89–24.03% with γ-PGA application (). The above-ground fresh weight per pot for the sample treated with γ-PGA increased from 7.57% to 9.26% compared with that treated with T2. The highest above-ground fresh weight per pot significantly increased from 42.55 ± 1.15 g pot−1 in T2 to 46.49 ± 2.55 g pot−1 in T7. However, significant difference was observed between T2 and T3, suggesting that the above-ground fresh weight per pot can be significantly increased by γ-PGA, but not by glutamic acid, which is a unit of γ-PGA.
Table 2. Effects of urea, glutamic acid and Poly(γ-glutamic acid) on the Chlorophyll contents and biomass of potted rapeseed at the late seedling stage.
At maturity, all γ-PGA treatments resulted in increased total biomass, pods, seeds, and yield per pot compared with T2 (). The number of pods per pot significantly increased by 3.03–4.10% after γ-PGA application, but increased only by 0.69% after glutamic acid application. With γ-PGA application, the number of seeds per pod increased by 3.45–4.93%. All treatments using γ-PGA exhibited an increased crop yield by 6.46–10.98%, whereas glutamic acid application increased crop yield by only 0.63%. The crop yield significantly increased from 15.94 ± 0.55 g pot−1 in T2 to 17.69 ± 0.78 g pot−1 in T7. PGAU exhibited better performance in increasing crop yield than the urea physically mixed with γ-PGA. A significant yield increase was observed with the application of γ-PGA due to the higher numbers of pods per pot and the seeds per pod.
Table 3. Effects of urea, glutamic acid, and Poly(γ-glutamic acid) on yield and yield components of potted rapeseed at the maturity stage.
Effects of γ-PGA on the diversities of culturable soil microbes
Compared with T1, the number of cultivable microbes increased after applying fertilizer, especially the addition of γ-PGA (). Furthermore, the soil culturable bacteria and actinomyces treated with γ-PGA can be significantly improved by 15.18–22.51% and 74.92–107.93%, respectively, compared with those in T2 at the early seedling stage. The number of soil culturable bacteria, fungi, and actinomycetes treated with γ-PGA at the late seedling stage reached the maximum in their whole growth period, which were improved by 183.10–216.43%, 73.18–110.61%, and 134.17–149.84%, respectively, compared with T2 (P < 0.05). However, soil culturable fungi and actinomycetes were 4.15–8.29% and 5.15–8.90%, respectively, lower than T2 at the bud stage. These results indicated that the quantity of soil culturable microbes can be improved by γ-PGA in the early growth stage of rapeseed.
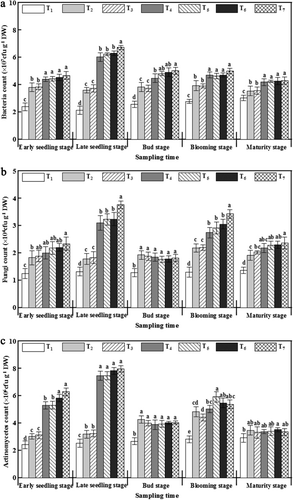
Diversity of soil microbes by DGGE clustering at the late seedling and blooming stages
The most important growth stages of rapeseed are the late seedling and blooming stages. The former is a basis, whereas the latter is a critical period of vegetation and reproduction (Zhang et al. Citation2013). The DGGE clustering analysis of the soil bacteria showed that the soil bacterial community diversity was obviously affected by γ-PGA application (). In addition, T4 and T5 exhibited the highest similarity of microbial community structure in the two periods. At the same time T6 and T7 had the highest similarity. The changes in fungi community diversity showed a similar trend with that of the bacteria. The similarity of the fungal community diversity in T4 and T5 was 88.32% at the late seedling stage, whereas the similarity between T6 and T7 was 94.69% in the blooming stage. These results showed that the effects of the application method of γ-PGA on the soil microbial community structure were greater than its application amount.
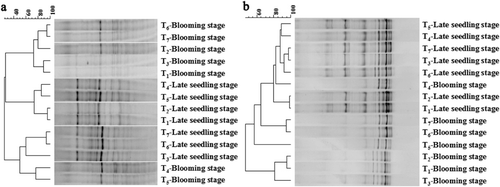
Compared with T1, the band number and Shannon index of the bacterial and fungal communities of T4–T7 were improved, whereas those of T2 and T3 were similar to T1 (). Both the bacteria and fungi band numbers in the T7 treatment were highest, which were 92.86% and 52.38%, respectively, higher than that of T2 at the late seedling stage. The Shannon index of the bacteria and the fungi in γ-PGA treatment group (T4–T7) were increased by 13.16–21.49% and 3.88–8.14%, respectively, compared with that of the control T2. The band number and Shannon index of both bacteria and fungi in the γ-PGA treatment group at the blooming stage exhibited similar trends with that at the late seedling stage. These results indicated that soil microbial community diversity can be increased by γ-PGA application.
Table 4. Effect of poly(γ-glutamic acid) on diversity of soil bacteria and fungi at late seedling stage and blooming stage.
Effects of γ-PGA on the content of different forms of N and urease in potted soil
In all the treatments, the levels of -N tended to be highest at the early seedling stage (). The levels of
-N with applied γ-PGA were higher than those under T2 for the entire growth period. The levels of
-N in treatments with γ-PGA were 5.34–8.21 mg kg−1 higher than those for T2 at the early seedling stage, which was the maximum difference throughout the entire growth period.
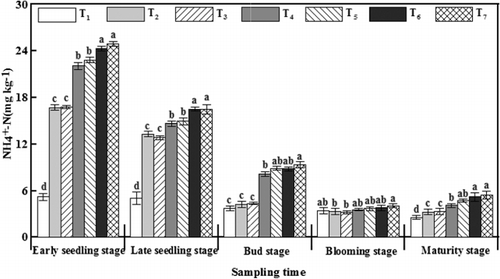
Nitrate N (-N) treated with γ-PGA increased from the early seedling stage, reached its maximum at the late seedling stage, and then continuously decreased to a minimum at maturity (). The levels of
-N treated with γ-PGA were 5.86–6.58 mg kg−1 lower than those under T2 at the early seedling stage, which was the maximum difference throughout the entire growth period. However, the amount of
-N after γ-PGA application was 3.21–4.92 mg kg−1 higher than that under T2 at the bud stage.
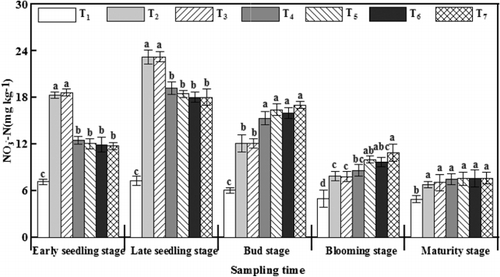
During the whole growth stage, the levels of SMBN treated with γ-PGA showed an upward trend from the early seedling stage to the late seedling stage, and then started to go downhill and maintained a relatively stable level (). This change was consistent with the changes of soil microbial community diversity after γ-PGA application ( and , ). Compared with T2, SMBN was significantly improved after γ-PGA application both at the early seedling and the late seedling stages. At the late seedling stage, the SMBN content was increased by 9.27–12.62 mg kg−1 after γ-PGA application, which was the maximum difference throughout the entire growth period. However, the content of SMBN after γ-PGA application decreased by 6.69–7.17 mg kg−1 at the bud stage, which had no significant difference with T2. These results indicated that more N in the soil was immobilized by the microbes after γ-PGA application at the early growth stage, and the immobilized N was then released for plant growth.
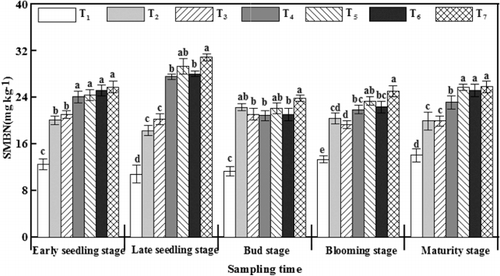
Similar to the results of the changes in SMBN, fixed -N was also significantly increased by γ-PGA application at the early seedling stage and late seedling stage compared with T2 (). The levels of fixed
-N treated with γ-PGA were 14.91–24.78 mg kg−1 higher than those under T2 at the early seedling stage, which was the maximum difference throughout the entire growth period. However, no significant difference among all treatments was observed at the blooming stage. The results suggested that more
-N content was fixed by clay minerals after γ-PGA application at the earlier growth stage of rapeseed, and then the fixed N was gradually released again.
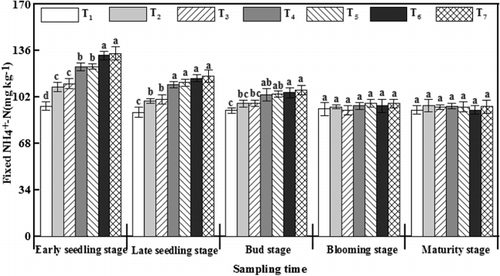
The urease activity in all the treatments increased from the early seedling stage, reached its maximum at the late seedling stage, and then continuously decreased to a minimum at maturity (). Compared with T2, urease activity was improved after γ-PGA application, and at late seedling stage and bud stage, it was significantly improved by 0.26–0.29 mg g−1 and 0.23–0.24 mg g−1 after PGAU application, respectively. Moreover, more amide nitrogen can be decomposed for rapeseed growth after the application of γ-PGA, especially PGAU, based on the above results.
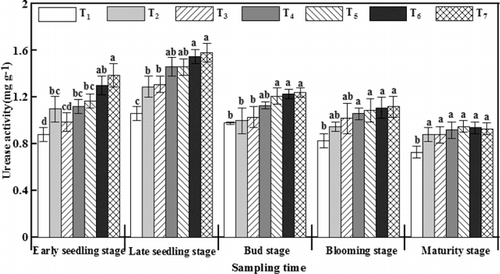
Discussion
To date, little research has been performed to ascertain the effects of γ-PGA on soil microbial diversity and nitrogen pools. Our results demonstrated obvious changes in the number of cultivable soil microbes, soil microbial community diversity, and content of different forms of N under γ-PGA application. The reduced N loss can be attributed to the capacity of the “ turnover pool” increased by γ-PGA. Furthermore, PGAU had the greatest positive effect.
Response of soil microbial diversity to γ-PGA application
Soil microorganisms are critical for the maintenance of soil function (Li et al. Citation2011), and the high soil microbial diversity is beneficial for improving the stability, productivity, and sustainability of the ecosystem (Ibekwe et al. Citation2002; Setala & McLean Citation2004). In our study, the soil microbial community diversity was increased by γ-PGA application, suggesting that the stability of soil ecosystem may be improved by γ-PGA. These findings were consistent with the recent studies that soil microbial community diversity can be changed by humic acid and other organic polymers (Puglisi et al. Citation2009; Geisseler et al. Citation2010; Zhang et al. Citation2012). Positive relationships between soil microbial population sizes, biomass, diversity, and plant biomass have been detected (Bardgett et al. Citation1999; Wang et al. Citation2012). Our results here showed that the biomass of rapeseed, the number of cultivable soil microbes, and the microbial diversity were increased by γ-PGA, which supports the above opinions.
Studies have reported that the soil microbial community structure and diversity can be greatly affected by types and application of fertilizer (Zhong et al. Citation2010; Chakraborty et al. Citation2011; Chhabra et al. Citation2013). Moreover, the amount of nutrient inputs and the nutrient condition of the soil may alter the microbial community structure and activities (Zhang et al. Citation2012; Berthrong et al. Citation2013). The polymer membrane of the coated fertilizer had slow-release fertilizer nutrient when the fertilizer was coated by polymer material (Zou et al. Citation2009; Tao et al. Citation2011). In this study, the application method of PGAU had greater effects on rapeseed growth and soil microbial community structure than that of urea physically mixed with γ-PGA. PGAU and urea physically mixed with γ-PGA are two different types of fertilizers because of the different methods of manufacture, indicating that they have different release mechanisms of fertilizer nutrient. In addition, the fertilizer synergist coated on the surface of the fertilizer works better than the direct physical mixing with fertilizer because of its less separation from fertilizer (Chen et al. Citation2008; Wu & Liu Citation2008; Akiyama et al. Citation2013). Therefore, PGAU can be a better choice for increasing the plant yield and stability of the soil ecosystem.
Response of different forms of N to γ-PGA application
In soil, urea is hydrolyzed by urease with an accumulation of ammonium (), and then
is converted to
(Zaman et al. Citation2008). The fixation and release of
can have a crucial role for the efficiency of fertilizer N (Juang et al. Citation2001). Our results in this study showed that fixed
-N was increased by γ-PGA application for the early plant growth stages, suggesting that γ-PGA can improve the N utilization efficiency. γ-PGA is a very unusual anionic biopolymer containing numerous carboxyl groups in the side chains (Bajaj & Singhal Citation2011).
can be adsorbed by the electrostatic potential field created by the carboxyl groups of γ-PGA or directly interact with the carboxylic sites at the early seedling stage (Ho et al. Citation2006; He et al. Citation2008). With the degradation of γ-PGA, the adsorbed
would be released again (Bhattacharya et al. Citation1998). Based on the above views, the γ-PGA applied with fertilizers can promote the fixation of N for the early plant growth stages. As a result, the N loss was reduced, the fertilizer efficiency was increased, and the crop yield was improved.
Soil urease, a kind of amidase used to catalyze the hydrolysis of the peptide bond of organic compounds containing nitrogen, can be applied as an indicator of organic nitrogen transformation in soil (Frame et al. Citation2012). Studies have shown that soil enzyme activities can be enhanced by humic acid and other organic polymers (Puglisi et al. Citation2009). Our study found that soil urease activity can also be increased by γ-PGA application, which correlated with the soil microbial quantity, biomass, and diversity (Ascher et al. Citation2009).
The γ-PGA, clay minerals, and soil microbes can act as an “ turnover pool” that can supply or store fixed or released
().
is transferred between the “
turnover pool” and an inorganic N pool in a dynamic balance. When the mineral N in the inorganic N pool was deficient,
in the “
turnover pool” can transfer into the inorganic N pool to make up for the deficiency in plant-available N and vice versa. In our experiment, more mineral N in the soil was immobilized by clays, soil microbes, and γ-PGA at the earlier growth stage. Then the immobilized N was released at the later growth stage for plant growth. Thus, the capacity of the “
turnover pool” was improved with γ-PGA application, which could reduce the loss of N and improve the fertilizer efficiency.
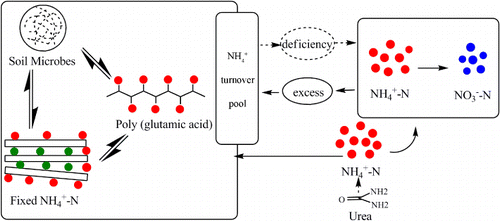
In conclusion, γ-PGA, an environment-friendly fertilizer synergist, can increase the yield of plant and improve diversity of the microbial community. Therefore, it can be a better choice for modern agriculture.
Acknowledgments
This work was funded by the National Basic Research Program of China (973) (No. 2013CB733600), the National Basic Research Program of China (973) (No. 2013CB733504), Graduate Student Innovation Project of Jiangsu Province (No. CXZZ13_0463), Program for Changjiang Scholars and Innovative Research Team in University (IRT1066), the National Nature Science Foundation of China (No. 21006050), Jiangsu Provincial Basic Research Program (Natural Science Foundation) (No. SBK200910195), the National Key Technology R&D Program (2011BAD23B04), and Nanjing Innovation Fund for Technology Based Firms (2012-062).
References
- Akiyama H, Morimoto S, Hayatsu M, Hayakawa A, Sudo S, Yagi K. 2013. Nitrification, ammonia-oxidizing communities, and N2O and CH4 fluxes in an imperfectly drained agricultural field fertilized with coated urea with and without dicyandiamide. Biol Fert Soils. 49:213–223. 10.1007/s00374-012-0713-2
- Ascher J, Ceccherini MT, Landi L, Mench M, Pietramellara G, Nannipieri P, Renella G. 2009. Composition, biomass and activity of microflora, and leaf yields and foliar elemental concentrations of lettuce, after in situ stabilization of an arsenic-contaminated soil. Appl Soil Ecol. 41:351–359. 10.1016/j.apsoil.2009.01.001
- Bajaj I, Singhal R. 2011. Poly (glutamic acid) - an emerging biopolymer of commercial interest. Bioresource Technol. 102:5551–5561. 10.1016/j.biortech.2011.02.047
- Bardgett RD, Mawdsley JL, Edwards S, Hobbs PJ, Rodwell JS, Davies WJ. 1999. Plant species and nitrogen effects on soil biological properties of temperate upland grasslands. Funct Ecol. 13:650–660. 10.1046/j.1365-2435.1999.00362.x
- Berthrong ST, Buckley DH, Drinkwater LE. 2013. Agricultural management and labile carbon additions affect soil microbial community structure and interact with carbon and nitrogen cycling. Microbial Ecol. 66:158–170. 10.1007/s00248-013-0225-0
- Bhattacharya D, Hestekin JA, Brushaber P, Cullen L, Bachas LG, Sikdar SK. 1998. Novel poly-glutamic acid functionalized microfiltration membranes for sorption of heavy-metals at high capacity. J Membrane Sci. 141:121–135. 10.1016/S0376-7388(97)00301-3
- Chakraborty A, Chakrabarti K, Chakraborty A, Ghosh S. 2011. Effect of long-term fertilizers and manure application on microbial biomass and microbial activity of a tropical agricultural soil. Biol Fert Soils. 47:227–233. 10.1007/s00374-010-0509-1
- Chen D, Suter H, Islam A, Edis R, Freney JR, Walker CN. 2008. Prospects of improving efficiency of fertiliser nitrogen in Australian agriculture: a review of enhanced efficiency fertilisers. Soil Res. 46:289–301. 10.1071/SR07197
- Chen FJ, Mi GH. 2012. Comparison of nitrogen accumulation and nitrogen utilization efficiency between elite inbred lines and the landraces of maize. Acta Agric Scand Sect B-Soil Plant Sci. 62:565–569.
- Chen X, Chen S, Sun M, Yu Z. 2005. High yield of poly-gamma-glutamic acid from Bacillus subtilis by solid-state fermentation using swine manure as the basis of a solid substrate. Bioresource Technol. 96:1872–1879. 10.1016/j.biortech.2005.01.033
- Chen Y, Ren CG, Yang B, Peng Y, Dai CC. 2013. Priming effects of the endophytic fungus phomopsis liquidambari on soil mineral N transformations. Microbial Ecol. 65:161–170. 10.1007/s00248-012-0093-z
- Chhabra S, Brazil D, Morrissey J, Burke J, O'Gara F, Dowling DN. 2013. Fertilization management affects the alkaline phosphatase bacterial community in barley rhizosphere soil. Biol Fert Soils. 49:31–39. 10.1007/s00374-012-0693-2
- Dahlin AS, Marstorp H. 2012. N release pattern from green manures can be modified through species composition. Acta Agric Scand Sect B-Soil Plant Sci. 62:659–665.
- Dinnes DL, Karlen DL, Jaynes DB, Kaspar TC, Hatfield JL, Colvin TS, Cambardella CA. 2002. Nitrogen management strategies to reduce nitrate leaching in tile-drained Midwestern soils. Agron J. 94:153–171. 10.2134/agronj2002.0153
- El-Tarabily KA, Hardy GESJ, Sivasithamparam K, Kurtboke ID. 1996. Microbiological differences between limed and unlimed soils and their relationship with cavity spot disease of carrots (Daucus carota L.) caused by Pythium coloratum in Western Australia. Plant Soil. 183:279–290. 10.1007/BF00011443
- Frame WH, Alley MM, Whitehurst GB, Whitehurst BM, Campbell R. 2012. In vitro evaluation of coatings to control ammonia volatilization from surface-applied urea. Agron J. 104:1201–1207. 10.2134/agronj2012.0009
- Funk R; The Davey Tree Expert Company. 2009 May 19. Fertilizers containing polyamino acid. United States Patent US 7,534,280.
- Geisseler D, Horwath WR, Joergensen RG, Ludwig B. 2010. Pathways of nitrogen utilization by soil microorganisms-A review. Soil Biol Biochem. 42:2058–2067. 10.1016/j.soilbio.2010.08.021
- Guan SY. 1986. Soil enzymes and their research methodology. Beijing: Agricultural Press.
- Han XM, Zhang DP, Cao ZP, Dehne HW. 2013. Fusarium populations in soil under long-term organic and chemical fertilizer treatments. Acta Agric Scand Sect B-Soil Pl. 63:253–260.
- He GH, Yang TX, Yang Z; Bei Jing Lv Meng Patent and Trademark Firm. 2008 Jan 09. Methods of improving crop growth, seed regeneration, and soil regeneration. China Patent CN 101099491A. Chinese.
- Henriksen A, Selmer-Olsen AR. 1970. Automatic methods for determining nitrate and nitrite in water and soil extracts. Analyst. 95:514–518. 10.1039/an9709500514
- Ho GH, Ho TI, Hsieh KH, Su YC, Lin PY, Yang J, Yang KH, Yang SC. 2006. γ-Polyglutamic acid produced by Bacillus subtilis (natto): structural characteristics, chemical properties and biological functionalities. J Chin Chem Soc. 53:1363–1384.
- Huang JY, Song CC. 2010. Effects of land use on soil water soluble organic C and microbial biomass C concentrations in the Sanjiang Plain in northeast China. Acta Agric Scand Sect B-Soil Plant Sci. 60:182–188.
- Ibekwe AM, Kennedy AC, Frohne PS, Papiernik SK, Yang CH, Crowley DE. 2002. Microbial diversity along a transect of agronomic zones. FEMS Microbiol Ecol. 39:183–191. 10.1111/j.1574-6941.2002.tb00921.x
- Juang TC, Wang MK, Chen HJ, Tan CC. 2001. Ammonium fixation by surface soils and clays. Soil Sci. 166:345–352. 10.1097/00010694-200105000-00005
- Kalambukattu JG, Singh RD, Patra AK, Arunkumar K, Pote J. 2013. Land use practices in the Central Himalayan region affect soil enzyme activities and their relationship with carbon pools. Acta Agric Scand Sect B-Soil Pl. 63:403–408.
- King WE, Fister RP, Norris SJ. 2011 Aug 25. Slow-release fertilizer and method of making and using same. United States patent US 12,862,328.
- Kinnersley AM, Koskan LP, Strom DJ, Meah ARY; Donlar Corporation. 1994 Sep 27. Composition and method for enhanced fertilizer uptake by plants. United States patent US 5,350,735.
- Körner C, Arnone JA. 1992. Responses to elevated carbon dioxide in artificial tropical ecosystems. Science. 257:1672–1675. 10.1126/science.257.5077.1672
- Koskan LP, Meah ARY, Sanders JL, Ross RJ; Donlar Corporation. 1998 Jul 21. Method and composition for enhanced hydroponic plant productivity with polyamino acids. United States patent US 5,783,523.
- Lammel J. 2005. Cost of the different options available to the farmers: current situation and prospects. IFA International Workshop on Enhanced-Efficiency Fertilizers, Frankfurt, Germany, International Fertilizer Industry Association, Paris, France.
- Li QH, Wu FZ, Yang Y, Wang XZ. 2011. Effects of rotation and interplanting on soil bacterial communities and cucumber yield. Acta Agric Scand Sect B-Soil Pl. 57:733–747.
- Liu X, Guo KL, Zhang HX. 2012. Determination of microbial diversity in Daqu, a fermentation starter culture of Maotai liquor, using nested PCR-denaturing gradient gel electrophoresis. World J Microbiol Biotechnol. 28:2375–2381. 10.1007/s11274-012-1045-y
- Puglisi E, Fragoulis G, Ricciuti P, Cappa F, Spaccini R, Piccolo A, Trevisan M, Crecchio C. 2009. Effects of a humic acid and its size-fractions on the bacterial community of soil rhizosphere under maize (Zea mays L.). Chemosphere. 77:829–837. 10.1016/j.chemosphere.2009.07.077
- Setala H, McLean MA. 2004. Decomposition rate of organic substrates in relation to the species diversity of soil saprophytic fungi. Oecologia. 139:98–107. 10.1007/s00442-003-1478-y
- Shih IL, Van YT, Yeh LC, Lin HG, Chang YN. 2001. Production of a biopolymer flocculant from Bacillus licheniformis and its flocculation properties. Bioresource Technol. 78:267–272. 10.1016/S0960-8524(01)00027-X
- Silva JA, Bremner JM. 1996. Determination and isotope-ratio analysis of different forms of nitrogen in soils: 5. Fixed ammonium. Soil Sci Soc Am J. 30:587–594. 10.2136/sssaj1966.03615995003000050017x
- Tao S, Liu J, Jin K, Qiu X, Zhang Y, Ren X, Hu S. 2011. Preparation and characterization of triple polymer-coated controlled-release urea with water-retention property and enhanced durability. J Appl Polym Sci. 120:2103–2111. 10.1002/app.33366
- Wang D, Xu ZZ, Zhao JY, Wang YF, Yu ZW. 2011. Excessive nitrogen application decreases grain yield and increases nitrogen loss in a wheat–soil system. Acta Agric Scand Sect B-Soil Pl. 61:681–692.
- Wang J, Li X, Zhang J, Yao T, Wei D, Wang Y, Wang J. 2012. Effect of root exudates on beneficial microorganisms-evidence from a continuous soybean monoculture. Plant Ecol. 213:1883–1892. 10.1007/s11258-012-0088-3
- Wang Q, Chen S, Zhang J, Sun M, Liu Z, Yu Z. 2008. Co-producing lipopeptides and poly-γ-glutamic acid by solid-state fermentation of Bacillus subtilis using soybean and sweetpotato residues and its biocontrol and fertilizer synergistic effects. Bioresource Technol. 99:3318–3323. 10.1016/j.biortech.2007.05.052
- Wu JS. 2006. The determining method and application of soil microbial biomass. Beijing: Meteorology Press.
- Wu L, Liu M. 2008. Preparation and properties of chitosan-coated NPK compound fertilizer with controlled-release and water-retention. Carbohydr Polym. 72:240–247. 10.1016/j.carbpol.2007.08.020
- Xu H, Xu ZQ, Feng XH, Ouyang PK; Nan Jing Su Gao Patent And Trademark Firm. 2011 Dec 14. A drought resistance seed coating agent containing γ-PGA and its preparation method. China Patent CN 102276364A. Chinese.
- Zaman M, Nguyen ML, Blennerhassett JD, Quin BF. 2008. Reducing NH3, N2O and NO3--N losses from a pasture soil with urease or nitrification inhibitors and elemental S-amended nitrogenous fertilizers. Biol Fert Soils. 44:693–705. 10.1007/s00374-007-0252-4
- Zhang Q.-C., Shamsi IH, Xu DT, Wang GH, Lin XY, Jilani G, Hussain N, Chaudhry AN. 2012. Chemical fertilizer and organic manure inputs in soil exhibit a vice versa pattern of microbial community structure. Appl Soil Ecol. 57:1–8. 10.1016/j.apsoil.2012.02.012
- Zhang X, Liu F, He Y, Gong X. 2013. Detecting macronutrients content and distribution in oilseed rape leaves based on hyperspectral imaging. Biosyst Eng. 115:56–65. 10.1016/j.biosystemseng.2013.02.007
- Zhong WH, Gu T, Wang W, Zhang B, Lin XG, Huang QR, Shen WS. 2010. The effects of mineral fertilizer and organic manure on soil microbial community and diversity. Plant Soil. 326:511–522. 10.1007/s11104-009-9988-y
- Zou HT, Wang YS, Song HW, Han YY, Yu N, Zhang YL, Dang XL, Huang Y, Zhang YL. 2009. The production of organic-inorganic compound film-coated urea and the characteristics of its nutrient release. Agric Sci China. 8:703–708. 10.1016/S1671-2927(08)60268-0