Abstract
This study examined nickel (Ni) concentrations in Swedish soils and cereal grains and how soil properties and different fertilization regimes affect Ni uptake by plants. Analysis of data from the Swedish environmental monitoring program on arable land showed that Ni concentrations in soils were significantly correlated with clay contents (R2 = 0.55; p < 0.001), while the correlation with organic matter content was weak, although significant (R2 = 0.02; p < 0.001). Concentrations of Ni in grains were positively correlated with the concentrations of total Ni in soil (R2 = 0.13; p < 0.001), negatively correlated with soil pH values (R2 = 0.14; p < 0.001), and multiple regression including Ni in soil and soil pH improved the correlation (R2 = 0.34). Therefore, analysis of total Ni concentrations in soils together with pH values can provide some guidance on where low Ni concentrations in crops are most likely to occur. Data from long-term field trials showed that Ni concentrations in wheat grain did not increase significantly after long-term application of cattle manure or sewage sludge compared with mineral fertilized or unfertilized treatments. Differences detected were attributed to pH changes in soil and differences in yields. It was found that Ni in organic amendments was not easily available for crop uptake. Application of inorganic fertilizer (NPK) decreased Ni concentrations in wheat grain compared with the unfertilized treatment, probably due to dilution in high-yielding crops. Concentrations in grains were low, and mean concentrations were 0.18, 0.07, and 1.20 for winter wheat, spring barley, and oats, respectively. For barley, this implies that 77% of the samples from the monitoring program had Ni concentrations below a proposed critical concentration for optimal germination (0.09 mg kg−1). Nickel shortage can be an overlooked problem in Swedish seed production that needs further attention.
Introduction
A first positive crop response to nickel (Ni) addition was reported in the 1940s (Roach & Barclay Citation1946). Since then, a number of studies have focused on the functioning of Ni in plants. In 1987, it was demonstrated that Ni concentrations in barley below 0.09 mg kg−1 decreased seed germination (Brown et al. Citation1987a) and Ni was classified as an essential micronutrient for all higher plants. The only proven function of Ni in higher plants to date is the role as a central atom in the enzyme urease, which catalyzes the hydrolysis of urea to ammonia in plant tissues. Urease is important for the nitrogen (N) metabolism of growing plants and absence or a low Ni concentration causes a decline in urease activity (Eskew et al. Citation1984; Gerendás et al. Citation1999), which leads to an accumulation of urea, primarily in leaf tips causing necrosis (Eskew et al. Citation1984; Walker et al. Citation1985; Brown et al. Citation1987b; Krogmeier et al. Citation1991). Another function where Ni is essential is during remobilization of N from plant tissue to grain (Brown et al. Citation1990). No yield effect of Ni on mature, annual crops has yet been demonstrated. However, application of Ni to soil in pot experiments has been shown to increase dry-matter yield in young cereal crops (harvested after 2–8 weeks), both when urea was used as the source of N (Singh et al. Citation1990; Gheibi et al. Citation2009) and when other mineral-N forms were applied (Brown et al. Citation1987b; Rahman et al. Citation2005; Gheibi et al. Citation2009). Under field conditions, Ni deficiency and yield effects of Ni fertilization have so far only been reported for perennial species, especially pecan trees (Wood et al. Citation2006). Recently, it has also been shown that Ni affects the metabolism of several ureides in woody perennials (Bai et al. Citation2006). Thus, Ni could be an overlooked element in seed production of annual crops, especially on soils with a limited content of plant-available Ni.
The presence of nickel in soil is mainly dependent on the composition of the parent material, with soils formed from acidic rocks, such as granites, often having low concentrations. Clay soils have higher Ni contents than coarse-textured soils and Ni has a relatively high affinity for soil organic matter (Gonnelli & Renella Citation2013). The Ni content in arable topsoils varies between 10 and 4000 kg ha−1 and uptake by crops is relatively low (2–8 g ha−1) (Kabata-Pendias Citation2001; Brown Citation2006). Organic fertilizers, such as farmyard manure and in particular sewage sludge, often contain significant amounts of Ni (app. 20 g Mg−1 dry matter (dm)) relative to plant requirements (Andersson Citation1992; Eriksson Citation2001) although addition of Ni through these amendments is small in relation to the amounts present in soil. A crucial question is therefore whether the Ni added with organic fertilizers is available to plants. Earlier studies have shown conflicting results, some indicating that addition of organic fertilizers such as farmyard manure or sewage sludge increases plant uptake of Ni (Tadesse et al. Citation1991; Izewska Citation2009; Mishra et al. Citation2009), and others showing no changes or a decline in Ni concentration in different crops (Andersson Citation1976; Singh et al. Citation2010; Antonious et al. Citation2012; Pande et al. Citation2012). Several studies have also shown that mineral N application can decrease the concentrations of Ni in crops (Singh et al. Citation1990; L-Baeckström et al. Citation2006).
The objectives of the present study were to determine Ni concentrations in Swedish arable soils and cereal grains through evaluation of samples and existing data sets derived from an environmental monitoring program and relate concentrations to soil properties. In addition, archived crop samples from long-term field trials were used to study crop uptake of Ni affected by organic and inorganic fertilizers. The following questions were addressed in the study: (1) At which concentration range is Ni present in Swedish arable soils and which soil properties influence total Ni contents in soil? (2) Which soil properties affect plant availability of Ni and uptake by cereal crops? (3) How does application of organic and mineral fertilizers influence Ni concentrations in crops? and (4) What Ni concentrations are present in Swedish cereal grains?
Materials and methods
Soil and grain data from the Swedish environmental monitoring program on arable soils
The monitoring program was started in 1988 to evaluate the status of Swedish arable soils at 10-year intervals and to detect possible changes over time by analyzing soils and crops. Sampling points within the program were fixed and selected using a stratified method to represent all arable lands throughout Sweden. The data used in the present study included 1936 soil samples and 1961 grain samples of winter wheat (902 samples), spring barley (622 samples), and oats (437 samples) from two sampling periods (1988–1995 and 2001–2007). The samples were analyzed for all major trace elements and for a number of soil parameters including pH, organic matter content, and clay content. Compilations of the data sets have been published in national reports (Eriksson et al. Citation2000, Citation2010). In the present study, data from soil and cereal grain samples were used for an in-depth evaluation focusing on Ni.
Samples from long-term field experiments applying organic and mineral fertilizers
Running long-term field trials were used to investigate how organic and mineral fertilizers influence the Ni content in cereal crops.
Lanna site in southwestern Sweden
The trial at the Lanna research station in south-west Sweden (58°21N, 13°08E) was started in 1996 to study the effect of fertilizer N and different organic amendments on soil organic matter and crop yield. Soil biological properties are also monitored. The experiment has a randomized block design with four replicates in each treatment and each plot measures 8 m × 14 m. The treatments used in the present study were (A) No fertilization; (B) Ca(NO3)2 at 80 kg N ha−1 year−1; (C) (NH4)2SO4 at 80 kg N ha−1 year−1; (D) cattle manure at 8 Mg dm ha−1 every two years; and (E) sewage sludge at 8 Mg dm ha−1 every two years. The crop rotation consists of oats, spring barley, and winter wheat. Grain samples of winter wheat grown in 2010 were collected and analyzed for Ni.
Petersborg site in southern Sweden
The trial running at Petersborg (55°42N, 14°13E) was started in 1981 to test the impact of sewage sludge on soil and crop properties. Data from the following treatments were used to evaluate the effect of sewage sludge on crop uptake of Ni: (A) No fertilization; (B) sewage sludge at 4 Mg dm ha−1 every four years; (C) sewage sludge at 12 Mg dm ha−1 every four years; (D) mineral NPK at 140 kg N as Ca(NO3)2, 18 kg P and 63 kg K as PK 7-25; and (E) NPK (as D) + sewage sludge (as C). The experiment has a randomized block design with four replicates in each treatment and each plot measures 6 m × 20 m. The crop rotation consists of winter wheat, sugar beet, spring barley, and oil seed rape. Grain samples of winter wheat grown in 2010 were collected and analyzed for Ni.
Soil fertility experiments in southern and central Sweden
The long-term soil fertility experiments which were started between 1957 and 1966 at 10 locations in southern and central Sweden were used to study Ni uptake influenced by N fertilization using archived grain samples. A detailed description of the experimental design and main results are given by Carlgren and Mattsson (Citation2001). The two treatments included in this study are (A) No fertilization; and (B) NPK fertilization at 150 kg N, 30 kg P, and 60 kg K ha−1 year−1. Wheat samples taken from these treatments on 5–6 occasions between 1970 and 1995 were used as replicates in the statistical analysis, since only composite samples from the replicates within each trial have been stored.
Soil and grain analysis
Soil samples were dried, homogenized, and passed through a 2-mm sieve. After digestion in concentrated nitric acid (HNO3), Ni concentrations in soil were determined on an inductively coupled plasma mass spectrometer (ICP-MS; Elan 6100 ACP-MS; Perkin Elmer SCIEX instruments). Ni concentrations in grain samples were analyzed according to the same method. Whole grains were used to avoid contamination at milling.
Statistical analysis
Statistical analysis of data was done with comparative statistics, analysis of variance (single factor), and Tukey′s test using JMP 9 (SAS Institute Inc., Cary, NC, USA). Graphs were prepared using SigmaPlot 12.3 (Systat Software Inc., San Jose, CA, USA).
Since Ni data in soil and grain samples and data for clay and organic matter were skewed, log transformations were performed on these variables prior to statistical analysis. After log transformation, the data showed an approximate normal distribution for all variables. In the correlations made, only samples with Ni concentrations above the chemical detection limit for the ICP-MS instrument were included.
Results
Nickel in Swedish arable soils
The compilation of 1933 soil samples from the monitoring program showed that the mean Ni concentration in topsoil was 13.5 mg kg−1 dm (). The data did not follow a normal distribution, a few samples showed very high Ni concentrations, and the median value was 11.3 mg kg−1. The minimum and maximum values were 0.73 and 62.7, respectively, and 95% of the samples were within the range 2.3–35.4 mg kg−1. Mean Ni concentrations in Swedish arable soils are rather low compared with those in many other countries (Angelone & Bini Citation1992; Kabata-Pendias Citation2001). As shown in , Ni concentrations in soils were positively correlated with clay content (log-transformed data; R2 = 0.55; p < 0.001), which is in agreement with other studies (e.g. Kabata-Pendias Citation2001; Echevarria et al. Citation2006). The correlation between soil organic matter and soil Ni concentrations was weak, but statistically significant (; log-transformed data; R2 = 0.02; p < 0.001). This indicates that even though soil organic matter content has a slight positive correlation with Ni concentration, it does not seem to be an important factor for the Ni content in Swedish arable soils.
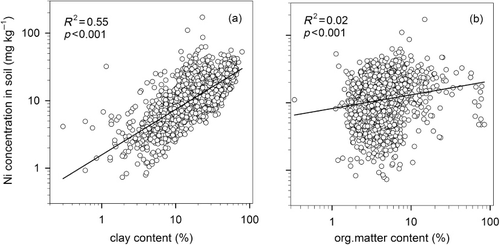
Table 1. Nickel (Ni) concentrations (HNO3 extraction) in Swedish arable topsoils (0–30 cm) and in grains of winter wheat, spring barley, and oats.
Long-term treatment with cattle manure and sewage sludge at the Lanna and Petersborg experimental sites has not led to any significant increase in Ni concentration in topsoil (). Although the amounts of sewage sludge applied in the field trials are up to four-fold higher than the application rate permitted for Swedish agricultural soils (approximately 700 kg dm ha−1 year−1), the amounts of Ni applied with sludge are small compared with total amounts present in the soil. The mean Ni concentration in sewage sludge used in the Petersborg experiment was approximately 20 mg kg−1 dm, which according to Eriksson (Citation2001) and Andersson (Citation2009) is the average Ni concentration in Swedish sewage sludge. The change in soil Ni concentration caused by 30 years of application of sewage sludge (3 Mg dm ha−1 year−1) was estimated to approximately 0.5 mg Ni kg−1 soil (dm), which represents a 6% increase in soil Ni concentration. Since the variation in Ni concentrations in soil is rather large (std. dev. 0.65 in the unfertilized treatment), the Ni increase is difficult to detect.
Table 2. Nickel (Ni) concentrations in soils and in wheat grain (HNO3 extraction) in response to treatments with organic and inorganic fertilizers at Lanna and Petersborg in 2010.
Nickel in Swedish cereal grain
Survey of concentrations in grain
Concentrations of Ni in the grain of winter wheat, spring barley, and oats are shown in . The mean concentration was 0.18, 0.07, and 1.20 mg kg−1 dm for winter wheat, spring barley, and oats, respectively, and concentrations ranged from <0.05 mg kg−1 dm (detection limit) to 10.31 mg kg−1 dm (one sample with a very high Ni concentration was excluded from the analysis). The data were not normally distributed and a few samples showed very high values. Approximately 99% of all samples had concentrations below 5 mg Ni kg−1 dm. There were large differences in Ni concentrations between crop types (p < 0.01) with barley having the lowest and oats the highest concentrations.
Influence of soil parameters
Analysis of the monitoring data showed a positive correlation between total amounts of Ni in soil (HNO3) and Ni concentrations in wheat grain (; log-transformed data; R2 = 0.13; p < 0.001). Similar correlations were found for spring barley and oats (R2 = 0.10 and 0.32, respectively, data not shown). Although these correlations were significant, there was a large variation in Ni uptake by crops at a given soil Ni content. There was a negative correlation between pH in soil and Ni content in wheat grain (; log-transformed data; R2 = 0.14; p < 0.001). A one-unit increase in soil pH on average led to a 33% decrease in Ni content in wheat grain although there was great variation in this correlation too. Moreover, multiple regression analysis with pH and Ni concentration in soil as independent variables resulted in a higher correlation with Ni concentration in wheat grain (; log-transformed data; R2 = 0.34; p < 0.001). The corresponding R2 values for spring barley and oats were 0.24 and 0.55, respectively (data not shown).
Influence of organic and mineral fertilizers
Results from the long-term field trial at Lanna showed that 15 years of different fertilizer application have caused some significant differences in Ni concentrations in crops (, ). Treatments with sewage sludge have resulted in higher grain Ni concentrations than treatments with cattle manure and Ca(NO3)2 (p < 0.05). However, compared with the unfertilized control and (NH4)2SO4 treatment, fertilization with sewage sludge has not led to an increase in grain Ni concentrations. Comparing total amounts taken up by crops (g ha−1), there was no difference between treatments with (NH4)2SO4 and sewage sludge. Application of cattle manure has led to a small but nonsignificant decrease in Ni concentrations in crops compared with the unfertilized and (NH4)2SO4 treatment. Soil pH values differed after 15 years of treatment, with sewage sludge and (NH4)2SO4 having reduced pH by approximately 0.5 unit compared with other treatments (). Thus, differences between treatments can be explained by changes in soil pH rather than Ni input with organic fertilizers.
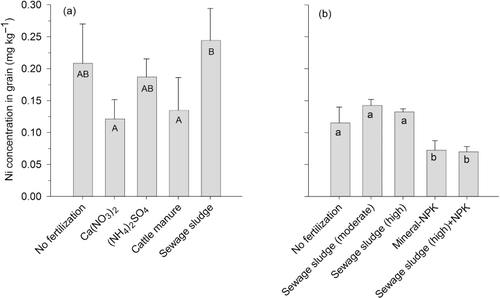
At Petersborg, no significant increase in Ni concentration in wheat grain was observed for the two levels of sewage sludge application compared with the unfertilized treatment (). Furthermore, the treatment with NPK+sewage sludge resulted in the same Ni concentrations in crops as the treatment with only NPK fertilizer, showing that sewage sludge had no effect on Ni concentration in crops. Amounts of Ni taken up were also similar for the two treatments. At this site, the decrease in soil pH after sludge application was less than in the experiment at Lanna (), which supports the results above that a decline in pH value plays a significant role for increased crop uptake of Ni.
The results from the Petersborg trial revealed that fertilization with mineral NPK has led to a significant decline in Ni concentration in crops compared with unfertilized treatments (p < 0.01; ). Crop yield was around 2500 kg for the unfertilized treatment and 6000 kg for the NPK treatment. However, the amount of Ni taken up was higher for the NPK-treated crop than the unfertilized crop (439 and 294 mg ha−1, respectively). A significant negative correlation was found between the N and Ni concentrations in crops (p < 0.05; ). The Ni/N-ratio declined from 0.068 in the unfertilized treatment to 0.029 in the NPK-fertilizer treatment, a decrease of 57%. A similar decline was found in the long-term soil-fertility experiments (; ), where Ni concentrations in the crop were significantly lower after long-term application of NPK compared with unfertilized treatments at 6 of the 10 locations (p < 0.05). Yield levels varied between 1562 and 3408 kg ha−1 in unfertilized treatment and between 4181 and 6851 in the NPK treatment ().
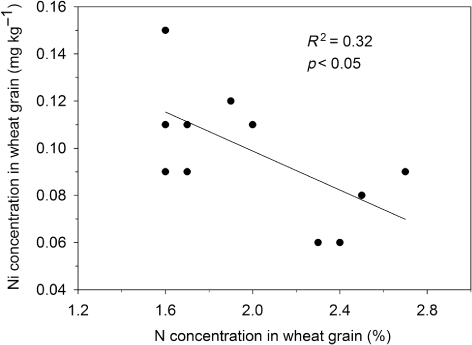
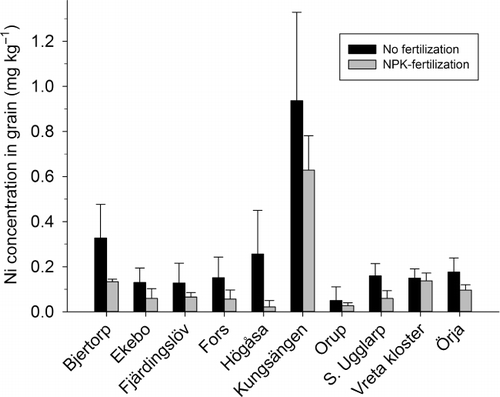
Table 3. Nickel (Ni) concentration in wheat grain (HNO3) and pH in soil (H2O) in unfertilized and NPK-fertilized treatments from the Swedish long-term soil fertility experiments at 10 different locations.
Discussion
Setting Ni concentrations in a practical perspective
The Ni concentrations in Swedish arable soils are generally low due to the formation of soils from mainly granite and gneiss. The strong positive correlation between Ni concentration in soil and clay content observed in this study is in agreement with other studies (e.g. McGrath & Loveland Citation1992; Kabata-Pendias Citation2001; Echevarria et al. Citation2006). The finding that soil pH is an important factor influencing Ni availability is also in accordance with previous studies (Yli-Halla & Palko Citation1987; Andersson & Christensen Citation1988; Echevarria et al. Citation2006; L-Baeckström et al. Citation2006). This implies that a possible Ni deficiency is most likely to occur on sandy soils with a high pH value. Total concentration of Ni in soil (HNO3) was only poorly correlated to Ni concentration in cereal grain. This is not surprising since total Ni concentration in soil is not necessarily a good indicator of plant-available Ni. Combining total Ni concentration in soil with pH values improved the prediction of Ni uptake by crops, but the variation was too large to be a useful tool. There is a need for more accurate determinations, for example extraction with EDTA or diffusion measurements (Yli-Halla & Palko Citation1987; Degryse et al. Citation2009). Ni uptake by crops can also be influenced by atmospheric deposition (Kabata-Pendias Citation2001). Average deposition rates in Sweden have decreased from 8.3 g Ni ha−1 year−1 in 1975 to 2.5 g Ni ha−1 in 2005 (IVL Citation2011). Today, Ni deposition and Ni amounts in crops are of similar magnitude. Although there is uncertainty regarding the utilization of deposited Ni by crops, it can be important for the Ni supply of crops.
There were large differences in Ni concentrations between the different crops analyzed, but the concentrations were generally low, particularly in spring barley. Brown (Citation2006) reported that concentrations below 0.2 mg Ni kg−1 have not been found in field-grown plants. However, results from a large Finnish survey reported similar Ni concentrations in Finnish cereal grain as in the current study (Varo et al. Citation1980) and L-Baeckström et al. (Citation2006) reported Ni concentrations of 0.14 mg kg−1 in wheat grains from a Swedish field trial. Data from the monitoring program revealed that many of the grain samples analyzed had concentrations below the proposed critical limit for optimal germination (0.09 mg Ni kg−1 dm). For spring barley in particular, as much as 77% of the analyzed grain samples were below this limit (). For winter wheat, the corresponding figure was 29%, while hardly any (0.2%) of the oat samples analyzed were below this concentration. Moreover, NPK fertilization resulted in Ni concentrations below the proposed critical concentration also in the Petersborg trial and from six locations in the soil fertility experiments. However, the study by Brown et al. (Citation1987a) was performed on barley grains and the same critical value may not be applicable for other crop species. Moreover, since only one study has identified critical concentrations for germination using other barley varieties, more experimental work is needed to state whether low Ni concentrations in Swedish cereals are a serious problem. Other studies have confirmed that small additions of Ni (5–20 mg L−1) to growth mediums increase germination or growth of early seedlings of sunflower (Ahmad et al. Citation2009), alfalfa (Peralta et al. Citation2001), and mung bean (Srivastava Citation2007).
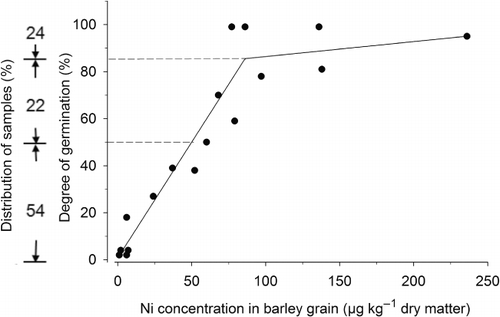
The concentrations of Ni reported to be toxic for plants range from 10 to 50 mg kg−1 dm (Davis et al. Citation1978; Macnicol & Beckett Citation1985; Kabata-Pendias Citation2001; Khan & Khan Citation2010). Since no samples within the monitoring program had Ni concentrations above 10 mg kg−1, it can be concluded that Ni toxicity is not a problem in Swedish cereal production. One should, however, be aware that under some conditions, e.g. in acidified sandy soils or acid sulfate soils, excessive Ni concentrations in crops can occur (Yli-Halla & Palko Citation1987).
Why does Ni application through organic fertilizers not cause an increase in Ni crop uptake?
Application of organic fertilizers to soil involves addition of a number of macro- and micronutrients and is considered to increase the supply to crops. Sewage sludge is therefore regarded as an amendment that can cause increased uptake of heavy metals by crops (e.g. Gonnelli & Renella Citation2013). However, organic amendments are a diverse group of products with varying properties. Sewage sludge can be derived from waste-water treatment plants applying different processes and using different chemicals for P precipitation. Anaerobic digestion may be followed by storage under aerobic conditions that can affect metal solubility. Moreover, the Ni content in sludge can differ. This can lead to contradictory results concerning plant uptake of Ni from sewage sludge. Some studies have indeed shown that Ni uptake by crops is increased after sludge application, probably owing to high Ni concentrations in the sludge used (Tadesse et al. Citation1991; Izewska Citation2009). In the current study, the high application rate of sewage sludge at the Petersborg and Lanna sites corresponded to a Ni addition of about 60 g ha−1 year−1. This is a large amount compared with Ni taken up by cereals, 2–8 g ha−1 year−1, but still small compared to the total Ni amount in soil (about 40 kg Ni ha−1 in topsoil on average). The fact that no or only a small increase in Ni concentration was found in wheat grain after 15–30 years of sewage sludge application at the two sites indicates that Ni in the sewage sludge was not present in forms available to plants. Even though mineralization of the organic matter added to soil over 15–30 years could lead to a higher Ni release than from the unamended soil, Ni concentrations in crops were not increased indicating that Ni was probably re-adsorbed on organic matter or mineral sites by carbonates or Mn- or Fe oxides (Wang et al. Citation1997).
Long-term manure application did not lead to increased Ni concentrations in crops either. However, in this case less Ni was applied with manure than with sewage sludge and the soil pH increased causing Ni to be less available for crops. Our results indicate that applying Swedish sewage sludge or cattle manure at permitted rates of 700 and 3000 kg dm ha−1 year−1, respectively, no increase in Ni concentration in crops can be expected. This is in accordance with another Swedish study (Andersson Citation1976) and results reported in the literature (Singh et al. Citation2010; Pande et al. Citation2012).
The addition of a water-soluble Ni salt (3.1 kg Ni ha−1 year−1) together with sewage sludge caused a 230% increase of the Ni concentration in wheat grain compared with the NH4SO4 treatment (Lanna site, data not shown). This shows that if Ni is present in plant-available form, crop uptake of Ni will increase. Still, much higher Ni concentrations in crops could have been expected through addition of the soluble Ni salt due to the high application rate. Limited uptake by crops indicates that also soluble Ni was retained and bound in soil and/or that Ni uptake by crops was selective.
Is the lower Ni content in N fertilized crops the result of pH change in soil or dilution in crops due to higher yield?
High rates of mineral N fertilization caused a decrease in Ni concentration in wheat grain compared with unfertilized treatments in the long-term field trials. Similar observations have been made in other studies in cereals (Singh et al. Citation1990; L-Baeckström et al. Citation2006) and in other crops (dos Reis et al. Citation2011). The results from the long-term field trials showed that lower Ni concentrations were not caused by a pH change in soil. Moreover, the results from the Petersborg trial showed a significant negative correlation between N and Ni concentrations. The reason for a declining ratio with N fertilization is not obvious. However, if the supply of Ni by the soil is a limiting factor for crop utilization, as indicated by our finding that plant-available Ni was taken up in the Ni-salt treatment, higher yields may lead to a dilution of Ni in crops. In the long term, a larger total uptake of Ni by NPK-fertilized crops may also cause a decrease in the amount of plant-available Ni in soil, contributing to lower concentrations in crops.
Acknowledgments
This project was funded by The Swedish Farmers' Foundation for Agricultural Research (SLF).
References
- Ahmad MSA, Hussain M, Ashraf M, Ahmad R, Ashraf MY. 2009. Effect of nickel on seed germinability of some elite sunflower (Helianthus annus L.) cultivars. Pak J Bot. 41:1871–1882.
- Andersson A. 1976. Influence of organic fertilizers on the solubility and availability to plants of heavy metals in soils. Grundförbättring. 27:159–164.
- Andersson A. 1992. Trace elements in agricultural soils – fluxes, balances and background values. Swedish Environmental Protection Agency, Report no. 4077. Stockholm. Swedish.
- Andersson P-G. 2009. Slamspridning på åkermark – fältförsök med kommunalt avloppsslam från Malmö och Lund under åren 1981-2008 [Addition of sewage sludge to arable land – field trials with municipal sewage sludge from Malmö and Lund 1981-2008]. Stockholm (Sweden): Swedish Rural Economy and Agricultural Societies, Report No. 15. Swedish.
- Andersson PR, Christensen TH. 1988. Distribution coefficients of Cd, Co, Ni and Zn in soils. J Soil Sci. 39:15–22. 10.1111/j.1365-2389.1988.tb01190.x
- Angelone M, Bini C. 1992. Trace elements concentrations in soils and plants of western Europe. In: Adriano DC, editor. Biogeochemistry of trace metals. Boca Raton (FL): CRC Press; p. 19–60.
- Antonious GF, Kochhar TS, Coolong T. 2012. Yield, quality and concentration of seven heavy metals in cabbage and broccoli grown in sewage sludge and chicken manure amended soil. J Environ Sci Heal A. 47:1955–1965. 10.1080/03601234.2012.676509
- Bai C, Reilly CC, Wood BW. 2006. Nickel deficiency disrupts metabolism of ureides, amino acids and organic acids of young pecan foliage. Plant Physiol. 140:433–443. 10.1104/pp.105.072983
- Brown PH. 2006. Nickel. In: Barker AV, Pilbeam DJ, editors. Handbook of plant nutrition. Boca Raton (FL): CRC Press; p. 395–409.
- Brown PH, Welch RM, Cary EE. 1987a. Nickel: a micronutrient essential for higher plants. Plant Physiol. 85:801–803. 10.1104/pp.85.3.801
- Brown PH, Welch RM, Cary EE, Checkai RT. 1987b. Beneficial effects of nickel on plant growth. J Plant Nutr. 10:2125–2135. 10.1080/01904168709363763
- Brown PH, Welch RM, Madison JT. 1990. Effect of nickel deficiency on soluble anion, amino acid and nitrogen levels in barley. Plant Soil. 125:19–27. 10.1007/BF00010740
- Carlgren K, Mattson L. 2001. Swedish soil fertility experiments. Acta Agric Scand Sect B Soil Plant Sci. 51:49–78.
- Davis RD, Beckett PHT, Wollan E. 1978. Critical levels of twenty potentially toxic elements in young spring barley. Plant Soil. 49:395–408. 10.1007/BF02149747
- Degryse F, Smolders E, Zhang H. 2009. Predicting availability of mineral elements to plants with the DGT technique: a review of experimental data and interpretation by modeling. Environ Chem. 6:198–218. 10.1071/EN09010
- dos Reis AR, Favarin JL, Gallo LA, Moraes MF, Tezotto T, Junior JL. 2011. Influence of nitrogen fertilization on nickel accumulation and chemical composition of coffee plants during fruit development. J Plant Nutr. 34:1853–1866. 10.1080/01904167.2011.600412
- Echevarria G, Massoura ST, Sterckeman T, Becquer T, Schwartz C, Morel JL. 2006. Assessment and control of the bioavailability of nickel in soils. Environ Toxicol Chem. 25:643–651. 10.1897/05-051R.1
- Eriksson J. 2001. Halter av 61 spårelement i avloppsslam, stallgödsel, handelsgödsel, nederbörd samt i jord och gröda [Content of 61 trace elements in sewage sludge, farmyard manure, mineral fertilizers, precipitation and in soils and crops]. Stockholm (Sweden): Swedish Environmental Protection Agency, Report No. 5148. Swedish.
- Eriksson J, Mattsson L, Söderström M. 2010. Tillståndet i svensk åkermark och gröda – data från 2001-2007 [Survey of Swedish arable soils and cereal crops - data from 2001-2007]Stockholm (Sweden): Swedish Environmental Protection Agency, Report No. 6349. Swedish.
- Eriksson J, Stenberg B, Andersson A, Andersson R. 2000. Tillståndet i svensk åkermark och spannmålsgröda- jordartens betydelse för markegenskaperna, samband markfaktorer och elementhalter i kärna [Survey of Swedish arable soils and cereals crops – impact of texture for soil properties, correlations of soil factors and elemental contents in crops]. Stockholm (Sweden): Swedish Environmental Protection Agency, Report No. 5062. Stockholm. Swedish.
- Eskew DL, Welch RM, Norvell WA. 1984. Nickel in higher plants – further evidence for an essential role. Plant Physiol. 76:691–693. 10.1104/pp.76.3.691
- Gerendás J, Polacco JC, Freyermuth SK, Sattelmacher B. 1999. Significance of nickel for plant growth and metabolism. J Plant Nutr Soil Sci. 162:241–256. 10.1002/(SICI)1522-2624(199906)162:3%3C241::AID-JPLN241%3E3.0.CO;2-Q
- Gheibi MN, Malakouti MJ, Kholdebarin B, Ghanati F, Teimouri S, Sayadi R. 2009. Significance of nickel supply for growth and chlorophyll content of wheat supplied with urea or ammonium nitrate. J Plant Nutr. 32:1440–1450. 10.1080/01904160903092655
- Gonnelli C, Renella G. 2013. Chromium and nickel. In: Alloway BJ, editor. Heavy metals in soils-trace metals and metalloides in soils and their bioavailability. 3rd ed. Dordrecht: Springer; p. 313–334.
- IVL (Swedish Environmental Research Institute) [Internet]. 2011. Metaller i mossa. IVL, Svenska Miljöinstitutet AB, Stockholm; [ Cited 2012 Dec 4]. Available from: http://www3.ivl.se/db/plsql/dvsmossa$.startup.
- Izewska A. 2009. The impact of manure, municipal sewage sludge and compost prepared from municipal sewage sludge on crop yield and content of Mn, Zn, Cu, Ni, Pb, Cd in spring rape and spring triticale. J Elementol. 14:449–456.
- Kabata-Pendias A. 2001. Trace elements in soils and plants. 3rd ed. Boca Raton (FL): CRC Press LLC.
- Khan MR, Khan MM. 2010. Effect of varying concentration of nickel and cobalt on the plant growth and yield of chickpea. Aust J Basic Appl Sci. 4:1036–1046.
- Krogmeier MJ, McCarty GW, Shogren, DR, Bremner JM. 1991. Effect of nickel deficiency in soybeans on the phytotoxicity of foliar-applied urea. Plant Soil. 135:283–286. 10.1007/BF00010917
- L-Baeckström G, Lundegårdh B, Hanell U. 2006. The interactions between nitrogen dose, year and stage of ripeness on nitrogen and trace element concentrations and seed-borne pathogens in organic and conventional wheat. J Sci Food Agric. 86:2560–2578. 10.1002/jsfa.2646
- Macnicol RD, Beckett PHT. 1985. Critical tissue concentrations of potentially toxic elements. Plant Soil. 85:107–129. 10.1007/BF02197805
- McGrath SP, Loveland PJ. 1992. The soil geochemical atlas of England and Wales. Glascow (Scotland): Blackie Academic & Professional.
- Mishra M, Sahu RK, Sahu SK, Padhy RN. 2009. Growth, yield and elements content of wheat (Triticum aestivum) grown in composted municipal solid wastes amended soil. Environ Dev Sustain. 11:115–126. 10.1007/s10668-007-9100-9
- Pande J, Srivastava PC, Singh SK. 2012. Plant availability of nickel as influenced by farmyard manure and its critical toxic limits in french bean. J Plant Nutr. 35:384–395. 10.1080/01904167.2012.639919
- Peralta JR, Gardea-Torresdey JL, Tiemann KJ, Gomez E, Arteaga S, Rascon E, Parsons JG. 2001. Uptake and effects of five heavy metals on seed germination and plant growth in Alfalfa (Medicago sativa L.). Bull Environ Contam Toxicol. 66:727–734.
- Rahman H, Sabreen S, Alam S, Kawai S. 2005. Effects of nickel on growth and composition of metal micronutrients in barley plants grown in nutrient solution. J Plant Nutr. 28:393–404. 10.1081/PLN-200049149
- Roach WA, Barclay C. 1946. Nickel and multiple trace element deficiencies in agricultural crops. Nature 157:696. 10.1038/157696a0
- Singh A, Agrawal M, Marshall FM. 2010. The role of organic vs. inorganic fertilizers in reducing phytoavailability of heavy metals in a wastewater-irrigated area. Ecol Eng. 36:1733–1740. 10.1016/j.ecoleng.2010.07.021
- Singh B, Dang YP, Mehta SC. 1990. Influence of nitrogen on the behavior of nickel in wheat. Plant Soil. 127:213–218. 10.1007/BF00014428
- Srivastava P. 2007. Effects of pre-soaking of seeds with nickel salt on seedling growth in Vigna radiate L. cultivars. Adv Plant Sci. 20:423–425.
- Tadesse W, Shuford JW, Taylor RW, Adriano DC, Sajwan, S. 1991. Comparative availability to wheat of metals from sewage sludge and inorganic salts. Water Air Soil Pollut. 55:397–408. 10.1007/BF00211202
- Varo P, Nuurtamo M, Saari E, Koivistoinen P. 1980. Mineral element composition of Finnish foods. III. Annual variations in the mineral element composition of cereal grains. In: Koivistoinen P, editor. Mineral element composition of Finnish foods: N, K, Ca, Mg, P, S, Fe, Cu, Mn, Zn, Mo, Co, Ni, Cr, F, Se, Si, Rb, Al, B, Br, Hg, As, Cd, Pb and ash. Almqvist & Wiksell: Stockholm (Sweden); p. 171.
- Walker CD, Graham, RD, Madison, JT, Cary, EE, Welch, RM. 1985. Effects of Ni deficiency on some nitrogen metabolites in cowpeas (Vigna unguiculata L. Walp). Plant Physiol. 79:474–479. 10.1104/pp.79.2.474
- Wang P, Qu E, Li Z, Shuman LM. 1997. Fractions and availability of nickel in loessial soil amended with sewage or sewage sludge. J Environ Qual. 26:795–801.
- Wood BW, Reilly, CC, Nyczepir, AP. 2006. Field deficiencies of nickel in trees: symptoms and causes. Acta Hortic. 721:83–97.
- Yli-Halla M, Palko J. 1987. Mineral element content of oats in an acid sulphate soil area of Tupos village, northern Finland. J Agric Sci Finland. 59:73–78.