Abstract
Four tillage trials have been performed on moderately well-drained loam soil in southeast Norway for 30–37 years (mean 34), comparing reduced tillage (8–10 cm in spring) with autumn ploughing (25 cm). In some years, additional stubble harrowing in autumn (10–12 cm) was compared with harrowing only in spring. Weeds were controlled with herbicides. Straw residues were retained after around 1990 and no fungicides were used. Grain yields are reported for the last nine years, and compared with earlier years. Results are presented for a number of soil properties measured in recent years. Autumn harrowing gave no consistent yield benefit over harrowing only in spring. There was little difference between ploughed and unploughed treatments in mean grain yields over the whole trial period, and the variability between years was similar in both tillage systems. Relative grain yields, calculated as yields obtained without ploughing in percentage of those obtained with ploughing, appeared to be normally distributed around 100%. Responses were often positive in dry years, and negative in wet years. Reduced tillage gave higher P and K concentrations near the soil surface and slightly lower concentrations in deeper layers. There was little change in their levels, relative to earlier findings. Changes in bulk density and total porosity were mostly attributable to changes in the stratification of organic matter. Reduced tillage increased porosity at 4–8 cm depth and decreased it slightly at 24–28 cm, but there was no change in the intermediate layer. The moisture-holding capacity of the soil was altered little by reduced tillage, and soil aeration properties were satisfactory at all three depths measured. There was no change in the total amount of organic matter stored within the topsoil, despite marked changes in its distribution. Reduced tillage gave significant increases in aggregate stability and an indication of greater earthworm activity.
Introduction
Conservation tillage, comprising non-plough tillage systems with or without some form of shallow tillage, has been investigated in many field trials in Norway since the late 1970s. Besides economies in the use of inputs and labour, motivation for such tillage has been driven mainly by the government support given to farmers who practice it, as a means of reducing the risk of soil erosion and nutrient runoff to waterways. The omission of autumn ploughing has been shown in Norway to reduce erosion by up to 90% on vulnerable silty clay soils (Lundekvam & Skøien Citation1998; Øygarden Citation2000; Lundekvam Citation2007). Over the last two decades, this has led to a halving of the cereal area that is now ploughed annually in autumn. At an international level, the role of conservation tillage in relation to environmental issues and sustainability may be seen from recent reviews in the literature (Olesen Citation2002; Holland Citation2004; Karlen Citation2004; Hobbs Citation2007). The question of whether tillage has a role to play in carbon sequestration has been the subject of particular debate (Baker et al. Citation2007; Angers & Eriksen-Hamel Citation2008; Boddey et al. Citation2009; Powlson et al. Citation2011). Despite the increased area under conservation tillage in Norway, many farmers remain sceptical about the system. One objection to such tillage systems is their dependence upon herbicide use, particularly if the preparations used are found to be environmentally unsafe (Tørresen et al. Citation2003). Ludvigsen et al. (Citation2003) reported traces of glyphosate or its derivative AMPA in drainage or surface runoff in more than 90% of the cases studied in Norway. The average level found was higher than that permitted in drinking water, but well below that considered harmful to freshwater organisms. Another debate is as to whether weeds may become resistant to such herbicides (Bradshaw et al. Citation1997; Powles et al. Citation1998). There is also increasing concern about the effect of reduced tillage on fungal attacks, particularly those of mycotoxin-producing Fusarium spp. (Henriksen Citation1999, Citation2006; Dill-Macky & Jones Citation2000; Elen Citation2002). It is considered that leaving plant residues on the soil surface significantly increases the risk of mycotoxin contamination (Bateman et al. Citation2007). The extent to which such risks differ between soil types is being examined in a current project in Norway (Hofgaard et al. Citation2012).
Yield results from long-term trials are of interest to both farmers and policy-makers. Eight tillage trials have been in existence for several decades in Norway. Yields in four trials on loam soil, representative of about 25% of Norway's grain area were reported by Riley (Citation2006), and yields in four trials on silty clay loam and clay loam soils, representative of 60% of the grain area, were reported by Riley et al. (Citation2009). Results from two medium-term trials on soils in Central Norway, where about 10% of the grain area is found, were reported by Riley et al. (Citation2005).
In Norway, changes in several soil chemical and physical properties have been found to take place relatively rapidly when annual ploughing is omitted (e.g. Riley et al. Citation1985; Riley Citation1988a; Ekeberg Citation1992; Riley & Ekeberg Citation1998; Børresen Citation1999; Riley et al. Citation2005). In particular, plant nutrients become stratified; the lower topsoil horizon becomes denser and properties that are important for aeration, root penetration and drainage may be adversely affected. It is of interest to discover whether such changes remain constant or become more or less pronounced over time.
In this paper, yield results obtained over the last nine years in the four trials on loam soil mentioned above are compared with those reported previously from these trials. In addition, results are presented for a number of soil properties that have been measured in these trials over recent years. These are also compared with results of previous investigations at the same sites. These properties include plant nutrients, organic matter contents, soil bulk density, pore size distribution and air permeability. In addition, measurements of aggregate stability have been performed in three trials and of the presence of earthworms in one trial.
Material and methods
Location and soil
The four trials were situated on the former research station Kise, Nes på Hedmark (60°47′N 10°49′E, 125–135 m a.s.l.) on imperfectly drained brown earth soil derived from ground moraine till. The soil has been classified by the Norwegian Forest and Landscape Institute as Gleyed Melanic Brunisol (Canada Soil Survey Citation1998) and Eutro-Gleyic Cambisol, with patches of Phaeozem (IUSS Working Group WRB Citation2006). The texture () may be classified as clay loam (ISSS), loam (USDA) or sandy silt loam (Soil Survey E&W). In Norway it is known as ‘light clay’, containing 15–20% clay, 30–40% silt and 40–50% sand. The soils are fertile, with medium–high organic matter content (4–8%) in the topsoil. The soil also contains 15–20% gravel and large stones are frequent. The subsoil is naturally compact, due to glaciation, with bulk densities reaching 1.8–1.9 Mg m−3 below ca. 0.8 m depth. The soils are tile-drained at 1 m depth, but with variable drain spacing and age. Of the four trials reported here, trial 1 was previously considered to have the poorest drainage status, and new drains were laid with 16 m spacing in autumn 2001. Trial 3 was re-drained with 10 m spacing in autumn 2011. Trial 2 has not been redrained within the last 35 years. The drainage status of the trials has thus been variable, but is representative of soils in the region.
Table 1. Soil organic matter, gravel content and soil texture measured at 0–20 cm depth in samples taken in 1976.
Tillage treatments and crop management
Trial 1 was started in 1977 and trials 2–4 in 1980. Trials 1–3 ran until autumn 2013, but trial 4 was discontinued after 2009. Full details of the four trials up to 2004, including experimental design, plot sizes, tillage treatments and crop management, were given by Riley (Citation2006). The following account describes tillage treatments and management in the period 2005–2013. As before, the main tillage comparison was between ploughed and unploughed treatments. These were naturally performed on the same plots as those used in previous years, but for practical reasons the split-plots used for stubble tillage comparisons varied slightly between years.
Trials 1–3 had four replications of the main tillage treatments (autumn ploughing and tine harrowing in spring). Trial 1 had 27 × 32 m blocks with 6 m borders between each block. Stubble tillage has since autumn 2007 been performed on half of each block. Trial 2 had 27 × 25 m blocks with a 4 m border between blocks 1–2 and 3–4. Stubble tillage was included since autumn 2009 on half of each block. Trial 3 had 20 × 50 m blocks without borders between blocks, but with surrounding space for turning. A stubble tillage treatment was included from 2007. Trial 4 compared shallow tillage with autumn ploughing on two 70 × 215 m replicate blocks. Stubble cultivations were performed on the whole area in some, but not all years.
The greater plot size allowed a larger-scale comparison of tillage treatments, in order to detect problems that might have been overlooked on smaller plots, such as greater practical difficulties with weed control, crop residues etc.
No straw has been removed from these trials since about 1990. The straw has been cut and spread each year using a chopper mounted at the rear of the combine harvester. In three years after 2004, the straw and stubble was further cut using a Ferraboli stubble chopper. The tillage methods used have remained basically the same throughout the whole trial period. Stubble cultivation, when included, has been done to 10–12 cm depth in early September, by one or occasionally two passes of a Kongskilde Vibroflex harrow. The tines (6 cm width) are spaced at 25 cm, with 10 cm ‘goosefoot’ blades attached horizontally to them. shows typical residue cover in autumn after this treatment.
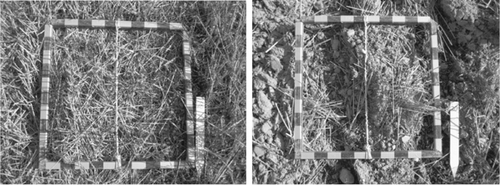
Autumn ploughing was performed to about 25 cm using a 35-cm Kverneland 2– or 3-furrow reversible mouldboard plough, with inversion in opposite directions in alternate years. The plough furrows were levelled in spring prior to harrowing using a locally made over-and-under rigid bar leveller. One extra harrowing of these plots was performed when necessary. Unploughed plots were cultivated in spring to 8–10 cm depth by one pass of the Vibroflex harrow described above. Thereafter, all plots, both ploughed and unploughed, were harrowed to about 5 cm depth by one pass of a Ferraboli horizontally rotating power harrow. In trial 4, an S-tine cultivator was used instead in some years.
Sowing and fertilising was performed in spring using a tractor-mounted Juko combine seed drill with drag-tine coulters spaced at 13 cm, followed by seedbed consolidation with a Cambridge roller. On two occasions, winter cereals were sown in trial 2 using a Tume machine with spring-tine coulters spaced at 16 cm. The tractors used in these trials weighed less than 4.5 tonnes, usually with standard rear tyre inflation pressures (ca. 80–120 kPa). All treatments in individual trials were sown on the same date, as early as practicable each year. Although the soil on unploughed treatments was often somewhat moister than on ploughed treatments, this is not thought to have led to postponement of sowing. The sowing dates have since 2005 ranged from 18 April to 14 May, on average around 1 May, which is one week earlier than the dates reported for the previous seven years (Riley Citation2006). The dates are representative of the normal time of sowing in the district. All treatments were harvested on the same day, usually in late August.
Crops grown in 2005–2013 were mostly spring cereals, except in two cases with winter rye/wheat in trial 2 in 2005 and 2008. In trial 1, barley was grown each year from 2005–2009. The same applied for trial 2 in 2006 and 2007. From 2010 to 2013, two crops per year were grown in these two trials, in consecutive strips across the trials. Oats, wheat and barley were rotated between years, within these years one oat crop, three barley crops and five wheat crops in each trial. In trial 3, only wheat was grown in 2005, followed by wheat and oats in monoculture (without rotation) from 2006 to 2010. In 2011, both wheat and barley were grown after each of these monocultures. Barley and oats were grown in 2012, followed by barley and wheat in 2013. Spring barley was grown in all five years in trial 4.
In spring-sown crops, all fertilisers were applied in the seedbed, row-placed at 25 cm spacing and slightly deeper than the seed, as compound NPK supplying 100–120 kg N ha−1 per year and around 20 kg P and 50 kg K ha−1. Fertiliser to winter-sown crops was spread on the surface in spring. No additional fertiliser was applied later in the season. The level of N was equal to that recommended in Norway for grain yields of around 4–5 Mg ha−1, which is close to the mean non-irrigated yield level in this district. Little crop lodging occurred in these trials.
Annual weeds were controlled in all trials by spraying once per season, with the same dosages on all treatments, using herbicides such as Actril 3D (ioxynil + dichlorprop + MCPA) and Ariane S (fluoxypyr + chlopyralid + MCPA). Occasional spraying with Barnon Plus (flamprop-M-isopropyl) was carried out to control wild oats (Avena fatua). All trials were sprayed each autumn with Roundup (glyphosate) at dosages of 1.5–3.0 l ha−1, 540–1080 g a.i. ha−1, to control perennial weeds such as couch grass (Elymus repens L.) and sow thistle (Sonchus arvensis L.), biennials such as mayweed (Maticaria inodora L.) and winter annuals such as meadow grass (Poa annua L.). The latter weed species was most prevalent in recent years. No fungicides or insecticides have been used in any of these trials, except to control snow mould (Monographella nivalis) with Sportak-EW (prochloraz) in winter cereals in trial 2.
Weather conditions
Growing season values of air temperature, rainfall and evaporation are shown in for the period 2005–2013, compared to the long-term values recorded at Kise weather station. The air temperature was slightly higher than normal throughout the season in all nine years. The moisture conditions in May were close to normal in five years, wetter in three years and much wetter in the last year. In June it was drier than normal in two years, close to normal in three, wetter in two and much wetter in two years. Later in the growing season the weather was wetter than normal in all but three years. On average there was 31% more rainfall than normal in the growing season. Potential evaporation was about 10% lower than normal, due to greater cloudiness. There was thus a considerable moisture surplus towards the end of the growing season in many of these years, whereas there is normally a deficit in this region.
Table 2. Weather conditions at Kise weather station in the growing seasons of 2005–2012, compared to long-term (1961–1990) average values (balance = rainfall minus evaporation).
Crop sampling and measurement of grain quality
Grain yields were recorded on 32 plots in each of trials 1–3, whilst in trial 4 they were recorded on five pairs of plots (ploughed/unploughed) within each block, giving 20 plots in all. A Wintersteiger small-plot combine harvester was used, with a harvested plot size of 18 m2 in trials 1–2 and 15 m2 in trials 3–4. Yields were expressed at 15% moisture content. Grain protein concentration and grain test (bulk) weight were measured in trials 1–3 from 2010 to 2013 by NIR spectrometry using an Infratec™ Grain Analyzer.
Due to the high cost of mycotoxin analyses, only a few samples were analysed for some of the toxins that are commonly found in Fusarium-infected grain, deoxynivalenol (DON) and HT2 + T2. All samples were from the years with monoculture of wheat and oats in trial 3. In 2006–2007, one oat sample per year was used from plots without ploughing. From 2008 until 2011, samples were used from plots both with and without ploughing and stubble cultivation, with in all eight wheat and six oat samples. Samples (200 g) were ground <1 mm in a Retsch mill, and concentrations of DON and HT2 + T2 were analysed as described by Aamot et al. (Citation2013), using ELISA kits: AgraQuant® Deoxynivalenol and AgraQuant® T-2/HT-2 Toxin (Romer Labs, Tulln, Austria), respectively. Absorbance was measured at 450 nm using a Biochrom Asys Expert Plus Microplate Reader (Biochrom Ltd., Cambrigde, UK). The limits of detection (LOD) and quantification (LOQ) with this method were 200 and 250 µg kg−1, respectively, for DON and 120 and 150 for HT2 + T2. Values below LOD are reported here as LOD/2, as practiced in the EU.
Soil sampling and measurement of soil properties
In April 2007, soon after snowmelt, soil was excavated to 20 cm from all ploughed and unploughed plots of trial 3, using 40 × 40 cm quadrats (32 litres). Earthworms were hand-sorted from this soil, counted and weighed live. Most individuals were of endogeic (topsoil-feeding) Apporectodea spp. No anecic (deep-burrowing) spp. emerged when the sample pits were flooded with a mustard powder solution, although channels into the subsoil were visible.
In October 2007, the soil was sampled randomly by hand auger at 0–10 cm and 10–20 cm depth on all ploughed and unploughed plots without stubble cultivation in trials 1 and 3. These samples were analysed for plant-available nutrients (P, K, Ca and Mg), using the ammonium-lactate (AL) extraction method described by Egnér et al. (Citation1960), and their pH was measured in a 1:2.5 soil:water ratio.
Undisturbed 100 ml core samples were taken at three depths (4–8, 14–18 and 24–28 cm) in autumn 2007 in trials 1 and 3 and in autumn 2008 in trials 2 and 4. The sampling was performed before any autumn cultivation, in neighbouring pairs of profiles dug on previously ploughed and unploughed plots that had never been stubble cultivated within the trial period. Nine profile pairs were sampled in trials 1–3 (three blocks per trial) and eight profile pairs were sampled in the large-scale trial 4 (four from each block, spaced ca. 40 m apart). Three cores per depth were taken in 2007 and two per depth in 2008. In the laboratory, the cores were saturated from below and placed in pressure chamber apparatus on ceramic pressure plates (Soil Moisture Inc.). The moisture content at saturation was taken to represent total porosity, as calculating the latter was hampered by the large variations in soil organic matter content, which affected particle density. Soil moisture contents were then measured after equilibration at tensions of −2, −10 and −100 kPa, which are equivalent to pore diameters of 160, 30 and 3 µm. Air permeability was measured at the −10 kPa stage, using the apparatus described by Green and Fordham (Citation1975). Saturated hydraulic conductivity (Ksat) is closely related to air permeability, and was calculated from the latter (expressed as µm2) using a pedotransfer function (PTF1) derived for Norwegian soils by Riley (Citation1996):
In early May 2011, after seedbed preparation, about 3 litres of soil were collected at random, using a spade to ca. 5 cm depth, within each ploughed and unploughed plot in trials 1–3. After air drying at room temperature, these samples were sieved for two minutes in a reciprocal shaker in order to determine aggregate size distribution, using sieves with 2, 6, 10 and 20 mm mesh openings. Larger (> ca. 5 mm) non-embedded gravel was removed by hand, but embedded and smaller (< ca. 5 mm) gravel was retained. The mean weight diameter of aggregates was calculated as described by van Bavel (Citation1949). The stability of aggregate fractions 2–6 mm and 6–10 mm was determined in a reciprocal rainfall simulator similar to that described by Njøs (Citation1967). Two samples (40 g) of each size group were placed within a radius of 15 cm and subjected to rain spraying for 2 minutes (pressure 1 bar, Hardi 4110-20 nozzles, nozzle height 35 cm and ca. 70 passes). Aggregate stability was expressed as the weight percentage of aggregates remaining on the sieves.
Results and discussion
Crop yields
As in previous years of the same trials (Riley Citation2006), tillage regime had little overall influence on mean grain yields in the period 2005–2013. There was however some variation between years, which is of interest in relation to yield stability.
The effect of stubble cultivation in autumn was investigated in 33 combinations of cereal and year, with an almost equal number of cases in each trial. There was no significant effect of stubble cultivation on yield, either on plots that were subsequently ploughed or on plots with reduced tillage (). Trial 1 had slightly higher yields (+3%) on plots with stubble cultivation than on those without, but in the other two trials an opposite trend was seen on plots with reduced tillage. Positive effects of stubble cultivation were in some cases seen in the wet years of 2011–2013, but this was not consistent in all crops. Although stubble cultivation had little effect on yields in these trials, it should be remembered that weeds were combated by herbicide use, without which stubble cultivation might have contributed to weed control, albeit to a lesser extent than ploughing. The potential benefit of stubble cultivation in the present trials lay most likely in enhancing the rapid breakdown of crop residues, which may be important for seedbed preparation and disease control.
Table 3. Grain yields (Mg ha−1 at 15% moisture), with and without stubble cultivation in autumn on soil that had either been unploughed for many years or annually ploughed.
Because stubble cultivation had only small and non-significant effects in these trials, the main effect of reduced tillage versus annual ploughing was assessed, where applicable, using the means of both stubble cultivation treatments. In trials 1–3, in cases where two crops per year were grown, the means of both crops values were used. Analyses of variance was performed for each trial with tillage as the main factor, tested against block × tillage, and with year and tillage × year as split-plot factors tested against the residual variance. The mean yields for the periods after 2004 (up to 2013 for trials 1–3 and up to 2009 for trial 4) are shown in , compared to those obtained in the same trials in previous periods.
Table 4. Mean grain yields (Mg ha−1 at 15% moisture) with annual ploughing and reduced tillage in years up to 2004, years after 2004 and for the whole period of the same trials.
In trial 1 there was no significant difference in average yields between plough tillage and reduced tillage for the period 2005–2013. In trials 2 and 4, yields in this period were on average 3–4% lower with reduced tillage than with plough tillage, whilst in trial 3 there was an opposite trend. Although the tillage interaction with year only approached significance in trial 4, higher yields with reduced tillage than with plough tillage were in 2006 recorded in all four trials. This was the warmest and driest year in the period after 2004. During this period, yield losses of 10–12% were seen with reduced tillage in trial 2 in 2011 and 2012. Both these seasons, especially that of 2011, were wetter than normal. In 2013, reduced tillage gave a yield loss of 28% in trial 2, due to seedling drowning caused by extremely high rainfall shortly after germination. The relative loss in barley was twice as high as that in wheat.
In the period 2006–2010, when wheat and oats were grown in monoculture in trial 3, neither crop responded negatively to reduced tillage, but oats out-yielded wheat by on average 0.6 Mg ha−1 (p < 0.01). In 2011, when the after-effects of such monoculture were investigated in wheat and barley, the wheat yield after oat monoculture was significantly higher than that after wheat monoculture (0.4 Mg ha−1, p < 0.01). There was, however, no interaction with tillage treatments. In the case of barley, low yields were recorded on oat rotation plots in one of the four replicate blocks due to a period of waterlogging. Exclusion of these plots from the dataset led to the same conclusion as that mentioned above for wheat. The beneficial rotation effect of oats as opposed to wheat is probably attributable to a lower incidence of fungal diseases. Our results suggest that the benefit is equally great both with and without ploughing. In previous tillage trials performed elsewhere in Norway (Riley et al. Citation2005), fungicide use was extremely beneficial in both ploughed and unploughed treatments, which also indicated that crop rotation is important for disease control, regardless of tillage system.
Over the whole period since the trials began (in 1977/1980), the mean yields obtained with reduced tillage were in trials 1–3 very similar to those obtained with annual ploughing.
In trial 4, the large-scale trial, reduced tillage gave about 5% lower yields than ploughing. This may have been due to a variety of causes, including greater soil variation, problems with straw residues that hampered sowing in some years and/or somewhat greater weediness. The mean yields were higher in trials 1 and 2 than in trials 3 and 4. This was probably due in part to slightly lower soil moisture–holding capacity in the latter trials.
Annual yield variability
The average yield level was in all trials close to or higher than the regional average (4.1 Mg ha−1 for spring cereals in Hedmark county over the period 2001–2012), but there were large variations in yield level between years (, above). Such variations may be due to weather variations and/or management factors. In a number of years low yields were due to moisture deficits, and in some but not all of these cases, reduced tillage gave better yields than did plough tillage (, below). This was seen particularly in the mid-1990s, as well as in 1987 and 2006. Low yields were sometimes attributable to lodging after heavy rain or grain shedding due to high winds. In 2002 they were partly due to herbicide damage.
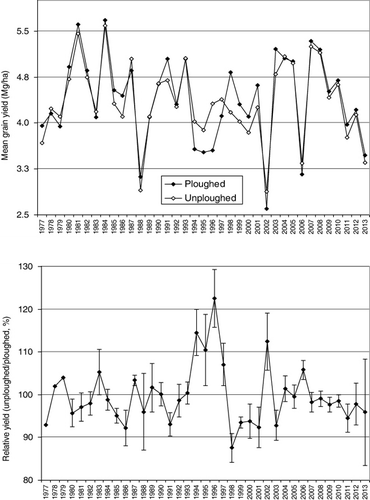
Correlations between yields and weather factors, such as monthly mean temperature and precipitation, were mostly low. The mean yield was negatively correlated with mean June temperature (p < 0.02), probably reflecting an association with moisture limitation. However, relative yields (yield without ploughing as a percentage of that with ploughing) were positively correlated with both the moisture balance in May (p < 0.02) and the mean temperature in August (p < 0.01). These relationships suggest that unploughed treatments yielded less than ploughed treatments when evaporation was higher than precipitation in May and/or when the mean temperature in August was less than 15°C (). The first of these correlations may have been due to poorer germination on unploughed soil in dry seasons, as a consequence of the shallower depth of sowing that is often found with reduced tillage (Riley Citation1988a). The second correlation is more difficult to account for. However, low August temperatures may lead to poor grain-fill, possibly to a greater extent on unploughed soil if crop development is delayed.
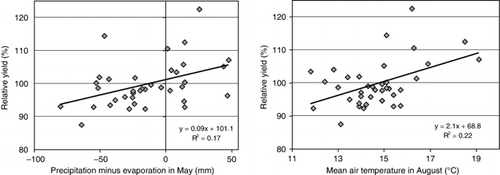
There was also a significant relationship (p < 0.001) between the absolute difference in grain yield (unploughed minus ploughed treatments) and yield level, showing that plough tillage was superior in years with yield levels above 4 Mg ha−1, whilst the opposite was true at lower yield levels (). This suggests that crops grown with ploughing performed best in seasons without yield limitation due to drought and/or in years with excessive rainfall, whilst those grown without ploughing performed best in seasons with yield limitation by drought. Although no clear relationship with moisture balance was found in the present case, previous studies on clay soil in Norway have shown that relative yields without ploughing are often higher in years with low summer rainfall than in wetter years (Riley et al. Citation2009).
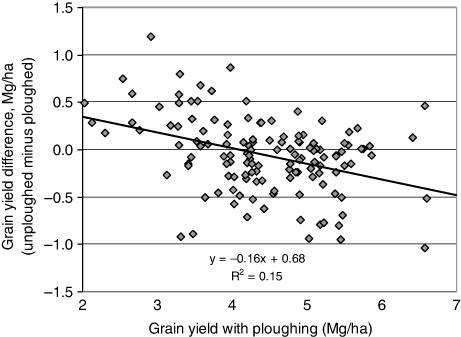
The frequency of years in which unploughed treatments performed better or worse than ploughed treatments showed a normal distribution when data from all four trials and all years were pooled (). Overall, the relative yield without ploughing was lower than that with ploughing in 54% of all cases, and equal or higher in 46%. It was within 5% of that with ploughing in 49% of all cases, within 10% in 73% of cases, and within 15% in 87%. The yield difference was thus greater than 15% in only 13% of all cases, of which less than half were cases where unploughed treatments gave poorer yield than ploughed treatments.
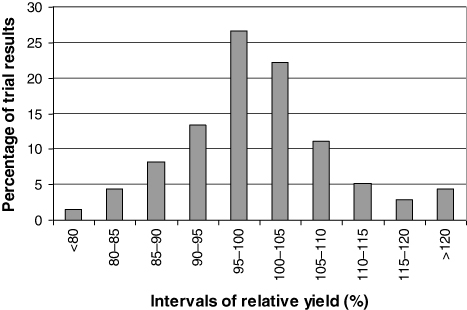
Yield variability between years and within fields is of major concern to farmers for economic stability. The between-year standard deviation for yields with ploughing was slightly greater than that for yields without ploughing in two of the four trials (). However, the variance ratio was < 1.5 in all cases, which is well below the significance level. The within-field variability was assessed from 2005 to 2009 in the large-scale trial, in which paired yields were measured at 10 locations. The coefficients of variation in individual years were in the range 7–20% and 5–19% for ploughed and unploughed treatments. In three years the variation was greatest with ploughing, whilst in two years it was greatest without ploughing. The variance ratio was in all cases below 3.5, which again is below the level of significance. Thus, these results do not suggest that yield variability was systematically greater with one tillage regime than with another, neither between nor within years.
Grain quality
No overall difference in grain moisture at harvest was found between tillage systems in the period 2005–2013, nor in grain test (bulk) weight in the years it was measured (2010–2013). In trial 2, the mean grain protein concentration (2010–2013) was 0.5% units higher (p < 0.05) with plough tillage than with reduced tillage. A similar effect of reduced tillage on grain protein was reported for a trial on clay soil by Riley et al. (Citation2009). However, trials 1 and 3 both showed the opposite in some years (tillage × year interactions p < 0.05). This was most marked in 2013, when protein concentration was 0.7% higher with reduced tillage.
In the two samples of oats grown without ploughing in 2006 and 2007 (the first and second year of monoculture), DON values were below LOD and only small amounts of HT2 + T2 were found (132 and 234 µg kg−1, respectively). The levels of mycotoxin were relatively low in later years also, and no increase over time was detected, despite the fact that the crops were grown without rotation or straw removal, and without the use of fungicides. The values with ploughing were often lower than those without, but the levels of significance were low due to the small number of samples. For DON in wheat, the range was <LOD-516 µg kg−1 with ploughing and <LOD-656 µg kg−1 without ploughing (mean ± SE 243 ± 98 and 358 ± 115, respectively, p = 0.07). In oats, the DON range was <LOD-305 µg kg−1 with ploughing and <LOD-521 µg kg−1 without ploughing (mean ± SE 226 ± 64 and 309 ± 112, respectively, p > 0.1). In the case of HT2 + T2, which was only analysed in oats, the range was <LOD-282 µg kg−1 with ploughing and 132–544 µg kg−1 without ploughing (mean ± SE 159 ± 65 and 342 ± 119, respectively, p = 0.08).
According to European Commission regulation no. 1881/2006, the present limit for DON in unprocessed grain for human consumption is 1250 µg kg−1 in wheat and 1750 µg kg−1 in oats. No limit has yet been set for HT2 + T2 in grain, but a draft Commission regulation suggests that values >1000 µg kg−1 should be further investigated. There are also guidelines that the limits in cereal products should be as low as 100 µg kg−1 for adults and 50 µg kg−1 for infants (http://www.efsa.europa.eu/en/efsajournal/doc/2481.pdf). For animal feed compounds the following HT2 + T2 limits apply in Norway: pigs and horses 200 µg kg−1, poultry (eggs) and young ruminants 600 µg kg−1 and adult ruminants 2000 µg kg−1. The DON levels that we measured in unprocessed grain were thus well below the limits set for grain for human consumption, irrespective of tillage, but the situation for HT2 + T2 is more uncertain.
Soil chemical properties
Soil tillage had no significant effect on soil pH or on AL-extractable calcium, magnesium and sodium, when measured in trials 1 and 3 in 2007. The mean pH (in water) was 6.3 in both trials and the levels of base cations were very high. The concentrations of AL-extractable phosphorus and potassium differed between sampling depths and there were significant interactions between soil depth and tillage treatment in both cases (). The phosphorus concentration was medium to low, and showed a slight accumulation in the upper layer of unploughed soil and a corresponding decline in the lower layer, whereas there was little difference between layers in ploughed soil. The potassium level was medium to high, and this nutrient accumulated in the upper layer of unploughed soil to an even greater extent than was the case for phosphorus. However, when account was taken of differences in bulk density, there was little overall difference between treatments in the total amounts of either nutrient within the topsoil. There appeared to have been little change in the levels or stratification of nutrients, compared to results reported earlier (Riley et al. Citation1985; Ekeberg Citation1992, Riley & Ekeberg Citation1998), but in the longer term non-inversion tillage may lead to deficiencies in the lower topsoil. Very high P concentrations in the surface layer are also undesirable on soils that are prone to erosion, as this may exacerbate eutrophication in waterways (Bechmann et al. Citation2011).
Table 5. Soil concentrations (mg kg−1) of ammonium lactate extractable phosphorus (P-AL) and potassium (K-AL) as affected by ploughing and depth of sampling.
Soil physical properties
Analyses of variance revealed few overall significant effects of tillage regime on the soil physical parameters measured in undisturbed cylinders in autumn 2007 and 2008. There were, however, significant interactions between tillage and sampling depth in many cases. Soil organic matter (SOM) concentration increased and bulk density (BD) decreased in the uppermost topsoil layer of unploughed soil, relative to ploughed soil, whilst the opposite was the case in the lowest layer (). For the upper layer in isolation, the difference in BD between tillage treatments was significant at p < 0.02. The differences in SOM and BD were small in the middle topsoil layer. There was little indication of any great change in BD within the upper 20 cm of soil, relative to values measured previously in the same trials (Riley & Ekeberg Citation1989; Ekeberg Citation1992; Riley Citation1998). BD at 20–30 cm depth has previously only been measured in trial 3 (Riley Citation1998) and in a former trial close to trial 1 (Riley & Ekeberg Citation1998). The present values do not differ greatly from those measured in those two trials. The calculated relative degree of compactness removes the direct effect of organic matter change on bulk density. In the upper layer of unploughed soil, the degree of compactness was at the level considered optimal for spring cereals (ca. 87%, according to Håkansson Citation2005). In all other cases, the soil was somewhat more compact than the optimum level. This was especially the case in the lowest layer of both ploughed and unploughed soil. This indicates that some soil compaction was present in both cases.
Table 6. Selected soil physical parameters, as affected by tillage and depth of sampling.
The total porosity reflected the differences in both BD and SOM, with which it was closely correlated (r = −0.91 and r = ±0.81, respectively, p < 0.001). The highest porosity was seen in the upper layer of unploughed soil, and in general it decreased somewhat with depth. This parameter was also negatively correlated with gravel content (r = −0.52, p < 0.001), which may account in part for the latter decline. The pore size distribution of the soil is shown in . There was a relatively large volume (ca. 10%) of large pores (>160 µm) at all depths, and a medium-high volume (20–25%) of pores able to retain plant-available water (0.2–30 µm). Relative to ploughed soil, there was a non-significant tendency for water retention to increase in the upper layer of unploughed soil, and to decrease in the lower layer, but there was little change in the overall amount of plant-available water.
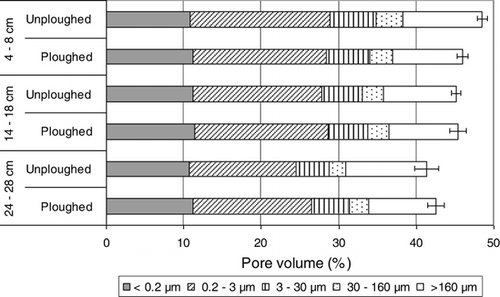
Mean air-filled pore space and air permeability at assumed field capacity (−10 kPa tension) were at all depths higher than the values considered to be limiting for plant growth (Riley Citation1988b), and tillage did not affect these properties significantly (). Mean values mask, however, the wide range that was found in the measured values of both variables. Their distribution was similar in both tillage regimes, and in both cases air permeability increased dramatically at air-filled pore space values above 10% (). There was nevertheless an indication that air permeability was slightly lower in unploughed soil than in ploughed soil at the same air space, possibly reflecting changes in pore geometry. There was also a somewhat higher frequency of very low permeability values in unploughed soil. Hydraulic conductivity (Ksat) calculated from air permeability gave mean values > 6 cm h−1 at all depths, regardless of tillage. The lowest values (around 1–3 cm h−1) were seen in trial 1, where the organic matter content was highest. Provided adequate functioning of drains, such low levels are normally sufficient for adequate infiltration to occur in most situations in southeast Norway, where rainfall intensity seldom exceeds 1 cm h−1 (Mamen et al. Citation2011). However, as was seen in trial 3 in 2013, low infiltration on poorly drained soil may lead to seedling drowning when very high rainfall occurs early in the growing season, especially in the absence of ploughing.
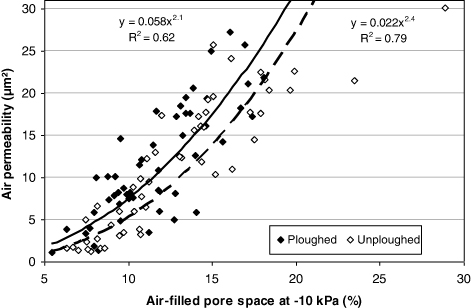
The total amounts of SOM within the upper 30 cm of soil were calculated, taking into account changes in BD. The SOM concentrations in were first adjusted according to the amount of gravel present. The mass of mineral soil particles in each 10 cm horizon was calculated by subtracting the SOM mass from the total soil mass. The mean mineral mass at 0–30 cm depth was almost identical in ploughed and unploughed soil (410 ± 7 and 411 ± 7 kg m−2, respectively), and the mean SOM mass did not differ significantly between tillage treatments (17.1 ± 1.0 and 15.7 ± 0.8 kg m−2, respectively). This confirms that although SOM stratification was altered by reduced tillage, the total amount stored within the topsoil was not. The depth of the boundary between topsoil and subsoil, recorded in each profile at the time of sampling, did not differ significantly between tillage treatments: the mean topsoil depth on ploughed plots was 28.0 ± 0.7 cm whilst on unploughed plots it was 26.4 ± 1.0 cm. It is thus considered unlikely that reduced tillage has had any effect on SOM in subsoil layers below 30 cm. This was confirmed in trial 3, whose main tillage plots were sampled at 10 cm intervals to 60 cm depth in 2011. Below 30 cm, there was no significant effect on ignition-loss of either tillage or sampling depth. Therefore, the present study does not provide any evidence that reduced tillage enhances carbon sequestration in soil. This is in agreement with earlier findings at Kise (Riley & Ekeberg Citation1998) and elsewhere (e.g. Baker et al. Citation2007).
The stratification of SOM within the topsoil is of relevance in relation to surface soil aggregate stability. The aggregate size distribution measured in spring 2011 was very similar in all three trials, and only small differences were found between ploughed and unploughed treatments (). A high proportion (50–60%) of the material passed through the 2 mm sieve, much of which was probably sand (). The relatively small mean weight diameters are probably a result of the rotary harrow that was used. Although the differences were small, unploughed soil had a significantly higher proportion of aggregates > 6 mm than did ploughed soil, and correspondingly less soil < 2 mm. The aggregate stability of both 2–6 mm and 6–10 mm aggregates was significantly higher (p < 0.001) on unploughed plots than on ploughed plots (), increasing on average from 62% to 86% in the smaller size group and from 81% to 93% in the larger. Similar beneficial effects of reduced tillage on the stability of soil aggregates near the surface have been reported previously in Norway by Marti (Citation1984), Børresen (Citation1993), Børresen and Njøs (Citation1993) and Riley et al. (Citation2008).
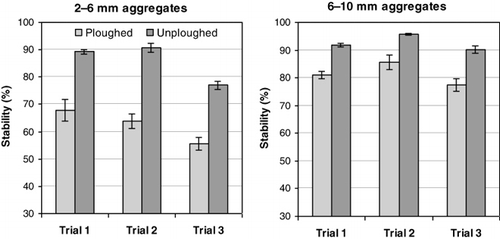
Table 7. Aggregate size distribution (wt.%) and mean aggregate weight diameter (mm) measured in the seedbed (0–5 cm) in trials 1–3 in spring 2011 (means of all trials, n = 12).
There was no interaction in aggregate stability between trials, and the absolute increases were similar in all cases, despite some difference between trials in their SOM content. The ignition loss measured in the 2–6 mm fraction was significantly (p = 0.004) higher on unploughed plots than on ploughed plots (mean increase 1.2%), but significantly (p = 0.007) lower in trial 3 than in trials 1 and 2 (7.7% vs. 8.9% and 9.1%). The stability of these aggregates was positively correlated with ignition loss in both unploughed and ploughed soil (). The stability increased by about 4% units per unit increase in ignition loss, and the correlations were significant at p < 0.01 and p < 0.05. In both cases there was one outlier with low stability, without which the variance accounted for would increase from 53% to 81% on unploughed soil and from 28% to 61% on ploughed soil. Stability would then increase by 5% and 7.5% units per unit increase in ignition loss, respectively. The stability of unploughed soil was generally high, whereas that of ploughed soil fell below 60% at ignition losses <7% (ca. 4.3% SOM).
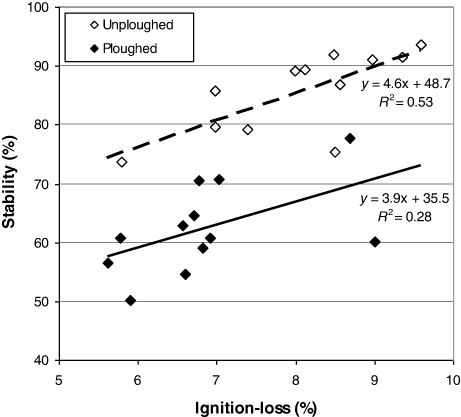
Aggregate stability is probably affected by biological factors as well as by the SOM level. The number of earthworms measured in trial 3 in 2007 was higher on unploughed plots than on ploughed plots in all four replicates, but levels varied considerably between blocks (). The statistical significance was therefore low (p = 0.09). The earthworm liveweight was in three replicates much higher on unploughed plots than on ploughed plots, but the opposite was found in one case, probably as a result of variation in the incidence of Lumbricus species. The overall effect of tillage on earthworm liveweight was therefore not significant. Reduced tillage was found to favour earthworm populations in these trials by Ekeberg (Citation1992) and by Riley et al. (Citation2005) in a trial on clay loam in central Norway. No benefit was seen, however, in another trial on loam soil (Riley et al. Citation2008). Earlier reports from many other countries (e.g. Ehlers Citation1975; Barnes & Ellis Citation1979; Edwards & Lofty Citation1982; Chan Citation2001) support the likelihood of beneficial effects on earthworm activity arising from reduced tillage. A recent study in Ireland showed that straw retention had a marked effect on worm numbers and mass with both minimum and conventional tillage (Kennedy et al. Citation2013).
Summary and concluding remarks
Yields
There was little difference between ploughed and unploughed treatments in the mean grain yields over the whole period from the start of the trials, and the yield variability between years was very similar in both tillage systems. There was no marked time trend in yield level. Relative grain yields, calculated as the yields obtained without ploughing in percentage of those obtained with ploughing, appeared to be normally distributed around 100%. Absolute yield differences between tillage systems were related to yield level, suggesting that reduced tillage performed best when yield levels were low, and vice-versa. This may indicate that reduced tillage is most successful under conditions with limited water availability for plants. Similarly, there was an indication that it is least successful under conditions with excessive rainfall when the degree of water-filled pores may limit gas exchange and aeration.
On these imperfectly to moderately well-drained loam soils, it appears that similar yields may be obtained over many years without ploughing as those obtained with annual autumn ploughing, even when considerable amounts of crop residue are retained and harrowing is performed only in spring. An absolute prerequisite is that weeds are adequately controlled by herbicide use. The main challenge is to combat perennial grasses, biennials and winter annuals such as Poa annua L. The high prevalence of the latter species necessitates spraying every year. Possible future restrictions on herbicide use would thus seriously limit the viability of reduced tillage systems.
Grain quality traits, such as protein and bulk weight, appear to be little affected by reduced tillage. However, it sometimes favours the occurrence of volunteer oats in later crops. The question as to how and when reduced tillage increases mycotoxin contents in grain needs further investigation, particularly on poorly drained soils and in wet regions. The present results indicate a tendency for reduced tillage to increase mycotoxin levels slightly, but the levels found in this study were relatively low, even though fungicides were not used in wheat and oat monocultures with large amounts of straw residue on the soil surface.
Soil properties
Reduced tillage gave higher concentrations of plant nutrients (P and K) close to the soil surface, and somewhat lower concentrations in deeper layers, but little long-term change in their level or stratification was found in these trials, relative to earlier findings. Changes in bulk density and total porosity were mostly attributable to changes in the stratification of organic matter. Reduced tillage led to an increase in the total porosity of the surface layer and slightly decreased it in the lowest layer sampled (at the boundary between top- and subsoil), but there was no change in the intermediate layer. The moisture-holding capacity of the soil was hardly altered by reduced tillage, and soil aeration properties appeared to be satisfactory at all depths.
All in all, therefore, it seems that reduced tillage over many years had remarkably little effect on soil porosity characteristics. Nor was there any change in the total amount of organic matter stored within the topsoil, despite marked changes in its distribution. The increase in organic matter content of the upper layer of unploughed soil resulted, however, in significant increases in the stability of surface soil aggregates. This is of importance for the avoidance of erosion in autumn and winter and of surface capping of the seedbed in spring. Such problems are less common on loam soils than on silt and clay soils elsewhere in Norway, but surface capping was sometimes observed on ploughed plots. Although only investigated in one trial in the present study, there was also an indication that reduced tillage increased earthworm activity, which is likely to have positive long-term effects on soil structure.
Other considerations
In order to perform well in large amounts of residue, more robust and expensive seed drills (with disc coulters) may be required in practice, compared to the type normally used in these trials. On the other hand, overall savings in machinery investment are likely with reduced tillage and its lower time requirement may result in earlier completion of spring work (Riley Citation1988c). This may offset possible delays in crop development due to lower soil temperature with reduced tillage, as reported by Børresen and Njøs (Citation1990), and associated with greater soil moisture in the presence of surface residues. Such delays were considered unlikely to have seriously affected crop yields in the present trials.
Higher soil moisture may also delay the start of spring tillage on unploughed soils, particularly those with poor drainage. Again, this was not considered to have been the case in the present trials. However, it should be remembered that relatively light equipment was used here, and greater soil compaction might be expected with the use of more modern, heavier machinery. The effect of reduced tillage on soil trafficability has been studied relatively little. A more robust soil aggregate system was reported by Wiermann et al. (Citation2000) after 25 years of shallow rotary tillage as compared with annual ploughing, suggesting that reduced tillage may improve conditions for the maintenance of favourable soil structure. Higher organic matter content near the surface may also make unploughed soils friable (i.e. tillable) over a greater range of soil moisture than ploughed soils, as reported for a clay soil by Børresen and Riley (Citation2003). Thus the interactions on soil structure of tillage regime and machinery weight require further study under Scandinavian conditions.
Acknowledgements
Dr. Heidi Udnes Aamot and Dr. Ingerd Skow Hofgaard at Bioforsk Plant Health and Plant Protection Division performed grain mycotoxin analyses and gave helpful advice on their interpretation. Thanks also to Dr. Annbjørg Kristoffersen and Dr. Bernt Hoel for helpful comments on the manuscript.
Funding
The Norwegian Ministry of Food and Agriculture provided financial support for maintaining these trials.
Additional information
Funding
References
- Aamot HU, Hofgaard IS, Brodal G, Elen O, Holen B, Klemsdal S. 2013. Evaluation of rapid test kits for quantification of HT-2 and T-2 toxins in naturally contaminated oats. World Mycotoxin J. 6:31–41. 10.3920/WMJ2012.1496
- Angers DA, Eriksen-Hamel, NS. 2008. Full inversion tillage and organic carbon distribution in soil profiles: a meta-analysis. Soil Sci Soc Am J. 72:1270–1274. 10.2136/sssaj2007.0342
- Baker JM, Ochsner TE, Venterea RT, Griffiths TJ. 2007. Tillage and soil carbon sequestration – what do we really know? Agric Ecosyst Environ. 118:1–5. 10.1016/j.agee.2006.05.014
- Barnes BT, Ellis FB. 1979. The effects of different methods of cultivation and direct drilling and of contrasting methods of straw dispersal on populations of earthworms. J Soil Sci. 30:669–679. 10.1111/j.1365-2389.1979.tb01016.x
- Bateman GL, Gutteridge RJ, Gherbawy Y, Thomsett MA, Nicholson, P. 2007. Infection of stem bases and grains of winter wheat by Fusarium culmorum and F. graminearum and effects of tillage method and maize-stalk residues. Plant Pathol. 56:604–615. 10.1111/j.1365-3059.2007.01577.x
- Bechmann M, Kværnø S, Skøien S, Øygarden L, Riley H, Børresen T, Krogstad T. 2011. [Effects of soil tillage on phosphorus losses: collation of results from Nordic investigations]. Bioforsk Rapport. 6:71. Norwegian.
- Boddey RM, Jantalia CP, Alves BJR, Urquiaga S. 2009. Comments on no-tillage and soil-profile carbon sequestration: an on-farm assessment. Soil Sci Soc Am J. 73:688–689. 10.2136/sssaj2008.0278l
- Børresen T. 1993. The effect on soil physical properties of undersown cover crops in cereal production in southeastern Norway. Norw J Agric Sci. 7:369–379.
- Børresen T. 1999. The effect of straw management and reduced tillage on soil properties and crop yields of spring-sown cereals on two loam soils in Norway. Soil Tillage Res. 51:91–102. Norwegian. 10.1016/S0167-1987(99)00030-6
- Børresen T, Njøs A. 1990. The effects of three tillage systems combined with different compaction and mulching treatments on soil temperature and soil thermal properties. Norw J Agric Sci. 4:363–371
- Børresen T, Njøs A. 1993. Ploughing and rotary cultivation for cereal production in a long-term experiment on a clay soil in southeastern Norway. 1. Soil properties. Soil Tillage Res. 28:97–108. 10.1016/0167-1987(93)90020-P
- Børresen T, Riley H. 2003. The need and potential for conservation tillage in Norway. Proceedings of the 16th Conference International Soil Tillage Res Organization, Brisbane; Vol. 1; p. 190–195.
- Bradshaw LD, Padgette SR, Kimball SL, Wells BH. 1997. Perspectives on glyphosate resistance. Weed Technol. 11:189–198.
- Canada Soil Survey. 1998. Soil classification: working group. The Canadian system of soil classification. 3rd ed. Agriculture and Agri-Food Canada Publication 1646; 187 pp.
- Chan KY. 2001. An overview of some tillage impacts on earthworm population abundance and diversity – implications for functioning in soils. Soil Tillage Res. 57:179–191. 10.1016/S0167-1987(00)00173-2
- Dill-Macky R, Jones RK. 2000. The effect of previous crop residues and tillage on Fusarium head blight of wheat. Plant Dis. 84:71–76. 10.1094/PDIS.2000.84.1.71
- Edwards CA, Lofty JR. 1982. The effects of direct drilling and minimal cultivation on earthworm populations. J Appl Ecol. 19:723–734. 10.2307/2403277
- Egnér H, Riehm H, Domingo WR. 1960. [Investigation of soil chemical analyses as a basis for determining the nutrient status of soil]. Lantbrukshoegsk Ann. 26:199–215. German.
- Ehlers W. 1975. Observations on earthworm channels and infiltration on tilled and untilled loess soil. Soil Sci. 119:242–249. 10.1097/00010694-197503000-00010
- Ekeberg E. 1992. Reduced tillage on loam soil: soil investigations. Norw Agric Res. 6:223–244 ( in Norwegian with English summary).
- Elen O. 2002. Plant protection in spring cereal production with reduced tillage. III. Cereal diseases. Crop Prot. 21:195–201. 10.1016/S0261-2194(01)00082-5
- Green RD, Fordham SJ. 1975. A field method for determining air permeability in soil. MAFF Technical Bulletin 29 “Soil physical conditions and plant growth”. London: HMSO; p. 273–287.
- Håkansson, I. 1990. A method for characterizing the state of compactness of the plough layer. Soil Tillage Res. 16:105–120. 10.1016/0167-1987(90)90024-8
- Håkansson, I. 2005. Machinary-induced compaction of arable soils Incidence – Consequences – Counter-measures. Soil Management Report no. 9. Uppsala: Division of Soil Management, Swedish University of Agriculture Science; 153 pp.
- Henriksen B. 1999. Factors affecting Fusarium infection and mycotoxin content in cereal Grains [ Dr. Scient. thesis]. Ås: Agricultural University of Norway, Vol. 4; 94 pp.
- Henriksen B. 2006. [The importance of cultural practices on the development of Fusarium and mycotoxins in grain]. Bioforsk Fokus. 3:40–41. Norwegian.
- Hobbs PR. 2007. Conservation agriculture: what is it and why is it important for future sustainable food production? J Agric Sci. 145:127–137. 10.1017/S0021859607006892
- Hofgaard IS, Seehusen T, Abrahamsen U, Razzaghian J, Le VH, Elen O, Strand E, Brodal G. 2012. Impact of agricultural practices on mycotoxin contamination of oats and spring wheat in Norway. Poster presentation at: 7th conference of the World mycotoxin forum and XIIIth IUPAC International Symposium, Rotterdam.
- Holland JM. 2004. The environmental consequences of adopting conservation tillage in Europe: reviewing the evidence. Agric Ecosyst Environ. 103:1–25. 10.1016/j.agee.2003.12.018
- IUSS Working Group WRB. 2006. World reference base for soil resources. 2nd ed. World Soil Resources Reports No. 103. Rome: FAO.
- Karlen, DL, editor. 2004. Soil quality as an indicator of sustainable tillage practices. Soil Tillage Res. 78:129–254.
- Kennedy TF, Connery J, Fortune T, Forristal D, Grant J. 2013. A comparison of the effects of minimum-till and conventional-till methods, with and without straw incorporation, on slugs, slug damage, earthworms and carabid beetles in autumn-sown cereals. J Agric Sci. 151:605–629. 10.1017/S0021859612000706
- Ludvigsen GH, Lode O, Skjevdal R. 2003. Retrieval of glyphosate and AMPA in Norwegian Streams, including studies on leaching during heavy rainfall. Poster presented at: XII Symposium on Pesticide Chemistry, Piacenza, Italy.
- Lundekvam H. 2007. Plot studies and modelling of hydrology and erosion in southeast Norway. Catena. 71:200–209. 10.1016/j.catena.2007.03.004
- Lundekvam H, Skøien S. 1998. Soil erosion in Norway. An overview of measurements from soil loss plots. Soil Use Manage. 14:84–89. 10.1111/j.1475-2743.1998.tb00620.x
- Mamen J, Benestad R, Haugen JE. 2011. Analysis of short term precipitation in Norway 1967–2010. Norwegian Meteorological Institute Report15; 34 pp.
- Marti M. 1984. Cereal monoculture without ploughing in South-East Norway – effects on yields and on soil physical and chemical properties [Dr. Scient. thesis]. Agricultural University of Norway; 155 pp. ( in German with English summary).
- Njøs A. 1967. Aggregate stability using artificial rain West-European methods for soil structure determination. Ghent: ISSS Working Group on Soil Structure, ch. VI; p. 53.
- Olesen JE, editor. 2002. Environmental effects of ploughless tillage. Dansk Jordbrugs - Forskning rapport nr. Danish Institute of Agricultural Science. 65; 106 pp. (in Danish with English summary).
- Øygarden L. 2000. Soil erosion in small agricultural catchments, south-eastern Norway [ Dr. Scient. Theses 2000:8]. Agricultural University of Norway; 26 pp.
- Powles SB, Lorraine-Colwill DF, Dellow JJ, Preston C. 1998. Evolved resistance to glyphosate in rigid ryegrass (Lolium rigidum) in Australia. Weed Sci. 46:604–607.
- Powlson DS, Whitmore AP, Goulding, KWT. 2011. Soil carbon sequestration to mitigate climate change: a critical re-examination to identify the true and the false. Eur J Soil Sci. 62:42–55. 10.1111/j.1365-2389.2010.01342.x
- Riley H. 1988a. Effects of reduced tillage on soil physical and chemical properties. Soil Management Report 77. Uppsala: Division of Soil Management, Swedish University of Agricultural Science; p. 45–57.
- Riley H. 1988b. Cereal yields and soil physical properties in relation to the degree of compactness of some Norwegian soils. Proceedings of the 11th Conference of the International Soil Tillage Research Organisation, Edinburgh, Vol. I; p. 109–113.
- Riley H. 1988c. Energy and labour use with various tillage systems. Soil Management Report 77. Uppsala: Swedish University of Agricultural Science, Division of Soil Management; p. 196–206.
- Riley H. 1996. Estimation of physical properties of cultivated soils in southeast Norway from readily available soil information. Norw J Agric Sci. Supplement 25:51 pp.
- Riley H. 1998. Soil mineral-N and N-fertilizer requirements of spring cereals in two long-term tillage trials on loam soil in southeast Norway. Soil Tillage Res. 48:265–274. 10.1016/S0167-1987(98)00165-2
- Riley H. 2006. Recent results and trends over time with conservation tillage on morainic loam soil in southeast Norway. Acta Agric Scand Sect B. 56:117–128.
- Riley H, Bleken M, Abrahamsen S, Bergjord AK, Bakken AK. 2005. Effects of alternative tillage systems on soil quality and yield of spring cereals on silty clay loam and sandy loam soils in the cool, wet climate of central Norway. Soil Tillage Res. 83:79–93. 10.1016/j.still.2004.03.005
- Riley H, Børresen T, Lindemark PO. 2009. Recent yield results and trends over time with conservation tillage on clay loam and silt loam soils in southeast Norway. Acta Agric Scand Sect B. 59:362–372.
- Riley H, Ekeberg E. 1989. Ploughless tillage in large-scale trials. II. Studies of soil chemical and physical properties. Norsk Landbruksforsking. 3:107–115 ( in Norwegian with English summary).
- Riley H, Ekeberg E. 1998. Effects of depth and time of ploughing on yields of spring cereals and potatoes and on soil properties of a morainic loam soil. Acta Agric Scand Sect B. 48:193–200.
- Riley H, Eltun R. 1994. The Apelsvoll cropping system experiment. II. Soil characteristics. Norw J Agric Sci. 8:317–333.
- Riley H, Njøs A, Ekeberg E. 1985. Ploughless tillage to spring cereals. II. Soil investigations. Forskning og forsøk i landbruk. 36:53–59 ( in Norwegian with English summary).
- Riley H, Pommeresche R, Eltun R, Hansen S, Korsaeth A. 2008. Soil structure, organic matter and earthworm activity in a comparison of cropping systems with contrasting tillage, rotations, fertilizer levels and manure use. Agric Ecosyst Environ. 124:275–284. 10.1016/j.agee.2007.11.002
- Tørresen KS, Skuterud R, Tandsæther HJ, Hagemo MB. 2003. Long-term experiments with reduced tillage in spring cereals. I. Effect on weed flora, weed seed bank and grain yield. Crop Prot. 22:185–200. 10.1016/S0261-2194(02)00145-X
- van Bavel CHM. 1949. Mean weight diameter of soil aggregates as a statistical index of aggregation. Soil Sci Soc Am Proc. 13:20–23. 10.2136/sssaj1949.036159950013000C0014x
- Wiermann C, Werner D, Horn R, Rostek J, Werner B. 2000. Stress/strain processes in a structured unsaturated silty loam Luvisol under different tillage treatments in Germany. Soil Tillage Res. 53:117–128. 10.1016/S0167-1987(99)00090-2