Abstract
Phosphate-solubilizing microorganisms play an important role in plant nutrition by enhancing phosphorus (P) availability to roots through converting the insoluble phosphates into soluble ions. We isolated phosphate-solubilizing bacteria (PSB) from acidic soil (Ultisols) in the field from the layer of 0–150 mm at a tea garden located at 28°38′26″ N and 116°24′27″ E. The capacity of bacterial isolates to solubilize mineral phosphate was tested on aluminum phosphate (AlPO4) in liquid medium. Among these PSB, isolate B1 (identified as Bacillus thuringiensis) exhibited the maximum P-solubilizing ability and was particularly efficient at solubilizing AlPO4 (up to 321 mg L−1) in vitro. The isolate B1 was inoculated to an acidic soil to study its effect on phosphate solubilization and growth of peanuts (Arachis hypogeae). The Olsen-P in the tested soil increased from 14.7 to 23.4 mg kg−1, with solubilization of 16.4 mg kg−1 soil of Occluded-P after 14-day incubation. The inoculation by B1 significantly increased plant height (from 37.7 to 45.7 cm), number of branches (from 34.0 to 52.7 per plant), hundred-seed weight (from 42.1 to 46.9 g) and crude protein content (from 243.5 to 268.2 g kg−1 dry weight). The phosphate-solubilizing B. thuringiensis strain B1 showed potential as a biological phosphorus fertilizer.
Introduction
Phosphorus (P) is one of the major essential macronutrients for plant growth and development. Most of soil P is in unavailable form and only about 1–2% of it may be taken up and incorporated into the aboveground plant parts (Juhi et al. Citation2011). Similarly, only a small proportion (about 10–20%) of applied P fertilizers can be used by plants, and the rest is rapidly converted into insoluble complexes in soil (Narsian & Patel Citation2000). A large percentage of P fertilizers applied to soils are converted to insoluble forms, thus increasing P fertilizer requirement (Podile & Kishore Citation2006). The frequent application of P fertilizers is not only costly but also depletes the nonrenewable phosphate-rock resources used in fertilizer manufacturing processes. In addition, many synthetic P fertilizers contain acids which tends to increase the acidity of the soil, which reduces the abundance and the diversity of beneficial organisms in soils, and which interferes with plant growth. Therefore, the attempts to improve P fertilizer efficiency and utilization of the plant-unavailable P in soils have long been a very important research topic.
The use of microbial fertilizers in agriculture has received more attention. Many soil microorganisms have the capacity to transform soil phosphate (Rodriguez & Fraga Citation1999). Inoculation of phosphate-solubilizing bacteria (PSB) improved solubilization of sparingly soluble phosphate compounds in soils, resulting in a higher crop yield (Whitelaw et al. Citation1997; Sundara et al. Citation2002). The PSB can solubilize inorganic phosphate (Zhu et al. Citation2011) through the processes of acidification, chelation, exchange reactions, and production of organic acids (Abd-Alla Citation1994; Singh & Reddy Citation2011).
Red clay soil (Ultisols in the American soil nomenclature) is widely distributed in tropical and subtropical climate zones and occupies 11.8% of the total land area in China. Rice production to support 22.5% of the population in the country (Lou et al. Citation2003) and the tea (Camellia sinensis) cultivation are usually on acidic, barren, and clay rich soils (volume-weight ~1.3 g cm−3, clay particle ≥40%, exchangeable aluminum 2–6 cmol(+) kg−1, base saturation percentage ≈40%), which have a low concentration (<15 mg kg−1) of soluble phosphate in soils causing P deficiency in crops (Lu 2000). The improvement of soil P is the most important issue in acidic soils because more than 80% of P in the soil were fixed by Fe and Al oxides and clay minerals (Xiang et al. Citation2003). Recently, the PSB strains have attracted the attention of agriculturists as soil inocula to improve plant growth and yield (Young Citation1994; Young et al. Citation1998; Goldstein et al. Citation1999; Fasim et al. Citation2002). Many PSB strains were reported in literatures, with some of them being able to almost substitute for synthetic P fertilizer in alkaline, calcareous, and acidic soils (Hao et al. Citation2006; Perez et al. Citation2007; Singh & Reddy Citation2011).
Little information is available on the isolation of PSB, increasing plant-available P and its benefit to plant growth in red soils (Ultisols). Hence, the objectives of this study were to (1) isolate bacteria from red soils with P-solubilizing capacity; (2) compare the PSB isolates for the capacity to solubilize aluminum phosphate (AlPO4) in liquid medium; and (3) test the effect of the best PSB isolate on increasing plant-available P and facilitating growth of peanuts in red soils.
Materials and methods
Bacterial isolation
For isolating P-solubilizing bacteria, red clay soils (Ultisols) were sampled in the field from the layer of 0–150 mm at a tea garden located at 28°38′26″ North latitude and 116°24′27″ East longitude. The soils were collected from four non-P-amended plots and mixed into one sample. The sampled soil contains 7.48 g kg−1 organic matter, 0.87 g kg−1 total N, 0.1 g kg−1 total P, 11.76 mg kg−1 Olsen available P, and has a pH value at 5.76, which were assessed using methods according to Lu (Citation2000) and Watanabe and Olsen (Citation1965). The sample was homogenized in sterile MilliQ water and diluted by 10−4, 10−5, and 10−6. Aliquots of each dilution were spread on modified Pikovskaya’s (PVK) (Pikovskaya Citation1948) agar medium with pH adjusted to 7.0 (Nautiyal Citation1999). The modified PVK agar medium contained only AlPO4 instead of Ca3(PO4)2 (Lopez et al. Citation2011). Four isolates were obtained and purified using single colony streak plate method. Each isolate was separately cultured in liquid Luria-Bertani (LB) medium containing 10 g NaCl, 5 g yeast extract, and 10 g tryptone per liter with pH adjusted to 7.0. Bacterial isolates were freeze-stored with 30% (v/v) glycerol at –80°C. All growth media were sterilized prior to use by autoclaving for 30 min at 115°C.
Phosphate-solubilizing capacity in AlPO4 liquid medium
Four replicates of each of four isolates (B1, B2, B3, and B4) were inoculated as 1-mL suspension (108 CFU mL−1) into 40 mL liquid PVK medium supplemented with 0.4 g AlPO4 and were cultured in Erlenmeyer flask at 28°C for 6 days with continuous agitation (150 r min−1). Three replicates of sterile water inoculated at the same dose were treated as blanks. The pH value of the medium was 5.2 before the experiment started. The flasks were sampled aseptically every 24 hours for the determination of acidity and soluble P. The acidity was assayed by reading on a pH meter, and the soil-available P was determined with molybdenum blue method, and the leach liquor was 0.5 mol L−1 NaHCO3 (Watanabe & Olsen Citation1965).
Each liquid medium of 20 mL of bacterial cultures were filtered through 0.2-m membrane filter. The filtrates were measured by High Performance Liquid Chromatography (HPLC) for organic acids, including oxalic acid, malic acid, tartaric acid, citric acid, succinic acid, and lactic acid. A filtrate of 20 μL was injected into HPLC (Model: Agilent HPLC1100, USA), which was equipped with Agilent Zorbax SB-Aq C18 250 × 4.6 mm (5 μm) column and detector DAD-G1315B. The mobile phase was 0.01 mol L−1 (NH4) H2PO4 with pH 2.5, flow rate 1 ml min−1, and temperature 30°C. UV absorption was measured at 214 nm (Yi et al. Citation2008). The organic acids were identified by comparing their retention time with those of the standards which concentrations were described by Zhang et al. (Citation2009).
Phosphate-solubilizing capacity in the soil incubation test
Based on the P-solubilizing capacity of PSBs in AlPO4 liquid medium, strain B1 was selected for examining the effect of its inoculation on P solubilization in the acidic red clay soil (Ultisols). Samples of 100 g soil (Ultisols, pH 5.39, organic matter 6.43 g kg−1, total N 0.87 g kg−1, total P 0.15 g kg−1 (Lu 2000); Olsen available P 14.8 mg kg−1 (Watanabe & Olsen Citation1965); clay proportion ≥40%) were placed in individual Erlenmeyer flasks and were inoculated with strain B1 at 10 ml kg−1 soil (with 108 CFU mL−1). Treated soils were incubated at 28°C and analyzed for soil pH and Olsen-available P after 1, 4, 7, 14, 21, and 28 days of incubation. The assay method was used (Jackson Citation1973). All treatments were repeated four times. Fractionation of inorganic soil P forms (Al-P, Fe-P, Occluded-P, and Ca-P) was measured after 1, 14, and 28 days of incubation (method according to Chang & Jackson Citation1957).
PSB population, soil pH, and peanut growth in pot experiment
To evaluate the P release and growth-promoting efficiency of the P-solubilizing bacterial strain B1, a pot experiment using the red clay soil (Ultisols, pH 5.32, total N 1.16 g kg−1, total P 0.16 g kg−1, organic matter 6.43 g kg−1, Olsen-available P 7.6 mg kg−1, and clay proportion ≥40%) planted with peanut was conducted at Nanjing Agricultural University (32°06′ N, 118°8′ E) in a polyvinyl chloride (PVC) covered greenhouse with 5 mW × 10 mL × 2.4 mH.
Four kilograms of nonsterile and sieved (1651 μm) red clay soil (Ultisols) were placed in a plastic pot (280 mm diameter × 300 mm height). Strain B1 was applied at 10 ml kg−1 soil (with 108 CFU mL−1) for B1 treatment; sterile water was applied at 10 ml kg−1 soil for control treatment.
Two peanut seeds were sown in each pot after the seeds were surface sterilized with sodium hypochlorite (1% v/v). Soil moisture was kept at 40% of field capacity. The pot experiment was arranged in completely randomized block design with four replicates for each of the two treatments, including a control treatment (blank) and a B1 inoculation.
The aboveground biomass of peanuts was harvested after plant height was recorded, and the roots were washed using slow-running water to remove soil particles and organic debris. After washing, the number of branches and nodules per root system and hundred-seed weight were recorded, and the seed crude protein content was measured (Lu Citation2000). The populations of PSB in the rhizosphere soil (Luo et al. Citation2010) were estimated at 15, 30, 60, 90, and 120 days after sowing, using a standard dilution plate counting technique and the modified PVK medium (see above for the composition).
Strain identification
Strain B1 was identified at the species level based on 16S rDNA genes (Yi et al. 2008) and GenBank sequence similarities (http://www.ncbi.nlm.nih.gov/).
Statistical analysis
Data were analyzed by SPSS 11.0 software. One-way analysis of variance (ANOVA) was done at the 0.05 significance level.
Results
Isolation of PSB strains
Four strains (B1, B2, B3, and B4) were found to form clear zones around their colonies on PVK agar plates. Among them, isolate B1 showed the maximum P-solubilizing activity as visualized by the size of the clear zone developed around the colony after 48-hour incubation.
P solubilization, pH change, and organic acid secretion of isolated PSBs with AlPO4 liquid media
The liquid mediums by inoculating four PSBs showed significant increase in the solubilization of AlPO4 (). The levels of soluble P in culture filtrates increased significantly up to the fourth day, but decreased on fifth and sixth day in the B1 treatment. Although the other isolates were significantly different compared with control, the maximum P solubilization was recorded by B1 (321.12 mg L−1). Small amount of phosphate was released during the process of sterilization. The concentration of soluble P in CK treatment increased from 13.14 mg L−1 to 23.98 mg L−1 during medium sterilization.
Note: CK – Sterile water added; B1 – PSB Isolate B1; B2 – PSB Isolate B2; B3 – PSB Isolate B3; B4 – PSB Isolate B4. The error bars are ± standard error, and the different letters denote the statistical difference among treatments on a particular sampling day, according to Duncan’s test (P ≤ 0.05). The tests were based on four replicates.
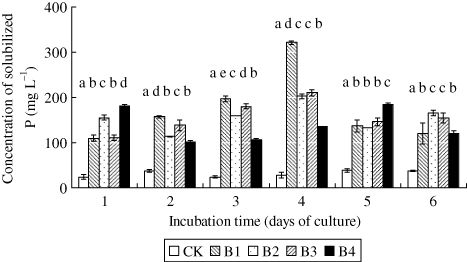
The growth of the isolates caused a significant increase in acidity. In about 6 days, the acidity of B1 treatment increased significantly from pH 5.0 to pH 3.3 (). In contrast, the acidity in the control treatment did not significantly change (slightly increased from pH 5.0 to 4.7).
Note: CK – Sterile water added; B1 – PSB Isolate B1; B2 – PSB Isolate B2; B3 – PSB Isolate B3; B4 – PSB Isolate B4. The tests were based on four replicates. The pH value of the medium was 5.2 for all the treatments before the experiment started.
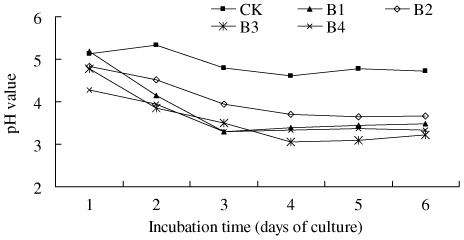
The total quantity of organic acids excreted by the isolates was shown in . The isolates of B1 and B3 yielded higher concentrations of organic acids than that of B2 and B4. The isolate B1 showed a P-solubilizing ability by producing high concentration of organic acid at 516 mg L−1 in the medium supernatant. There was a correlation between pH value and the concentration of organic acid when B1 was inoculated (R2 = 0.55, P > 0.05). The blank control did not produce any organic acid in detectable amounts (), and the phosphate solubilization was the lowest.
Table 1. The quantity of organic acids excreted by the PSB isolates in Pikovskaya’s (PVK) liquid media.
Effect of inoculation with B1 on available P, pH, and P transformation in soil incubation test
The variation trends of soil solubilized-P were in accordance with the liquid medium tested above: The soil soluble P climbed up and then declined after incubating 14 days and the solubilized-P in B1 treatment increased the most. The increment of soil Olsen-available P in B1 treatment rose to 23.4 mg kg−1 after 14 days. The pH of the soil samples was gradually reduced in B1 treatment compared to control (). The PSB B1 was able to solubilize almost 15.4 mg kg−1 soil Occluded-P after incubating 14 days (). B1 could transform soil Occluded-P into the Olsen-available P for plant absorption.
Note: CK – Sterile water added; B1 – PSB Isolate B1. The error bars are ± standard error. The tests were based on four replicates.
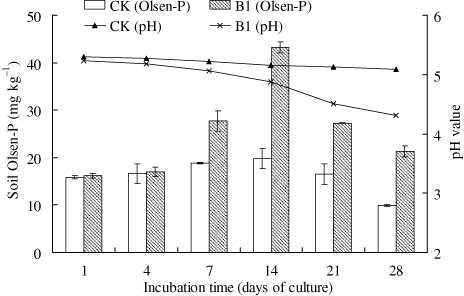
Table 2. Effect of inoculating PSB isolate B1 on forms of inorganic phosphorus in red clay soil (Ultisols) (mg kg−1).
Effect of inoculation with B1 on PSB population, soil pH, and peanut growth in pot experiment
B1-treated soil maintained higher numbers of PSB for at least 90 days, which indicated the presence of a facilitate effect on the growth and multiplication of B1, whereas the control failed to show any significant increase in their population (). The population density of PSB increased in the first 60 days and then decreased throughout the rest of the observation period in the B1 treatment. The pH of B1-treated soil was not very different from the control after 15 days, except for an unexpected temporary drop at 60 days (). Although it was unable to differentiate native PSB from the introduced one, the introduced PSB B1 was a native PSB, but this went through the processes of purification and increment of population via manual cultivation in liquid medium. The soil test serves two purposes: (1) Test the effect of stimulating PSB in native soil during cropping; and (2) test the effect of overall P solubilization and the consequence of crop yields in native soil. Our results suggested that there were good positive impacts in both the cases.
Table 3. Concentration of PSB isolate B1 in peanut rhizosphere (in 107 CFU g−1 soil).
Note: CK – Sterile water added; B1 – PSB Isolate B1. The error bars are ± standard error. The tests were based on four replicates.
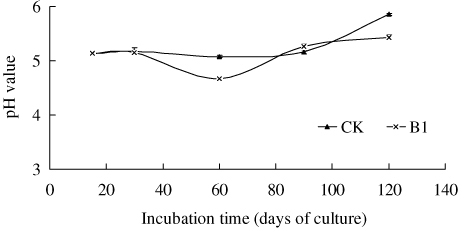
The whole plant total P concentration increased from 9 g kg−1 dry weight in control to 14 g kg−1 dry weight in B1 treatment. Plant height and the number of branches per plant were significantly enhanced by the inoculation of B1 compared to the control. The maximum plant height of 45.7 cm was recorded in B1 treatment. There was a higher number of branches and nodules per plant in the B1 treatment. The plant hundred-seed weight was significantly higher with the inoculation of B1 (46.9 g per plant) than in the control (42.1 g per plant). The crude protein content of peanut seeds significantly increased from 243.5 g kg−1 dry weight in control to 268.2 g kg−1 dry weight in B1 treatment ().
Table 4. Effect of PSB isolate B1 on the growth parameters of peanut.
Strain identification
In this study, only the identity of B1 was revealed, and the closest species of B1 strain was identified as Bacillus thuringiensis, and the similarity between their 16S rDNA genes was 99.99%. Thus, the isolate B1 was named as B. thuringiensis B1. We had applied for China patent for B1, the patent number is 201110095783.2. The NCBI accession number is S000979524.
Discussion
There are some differences among different P-solubilizing microbes in certain environments, which lead to significant difference of solubilizing functions under different conditions. In the present study, the P-solubilizing bacteria were isolated from the red clay soil (Ultisols) in a tea garden. The modified PVK medium was chosen for screening PSBs. Four PSBs had been isolated from the red clay soil in the tea garden. In the recent years, suggestions have been made for isolating PSB from the amended site for increasing efficiency. In this study, it was not targeted to isolate as much PSB as possible, nor targeted for solubilizing specific phosphate source, rather targeted for maximum survival rate of PSB in nonamended environment. The results showed that the solubilization efficiency of B1 was higher than other isolates after incubating it for 4 days; it then presented a decreasing tendency onward (). A plausible reason for such an observation could be attributed to the availability of soluble form of phosphate, which has an inhibitory effect on further phosphate solubilization (Narsian et al. Citation1995). The negative effect of soluble P on microbial acid productivity might also be responsible for final soluble P concentration (Rohr et al. Citation1983). Also, the decrease in the concentration of organic acid was expected due to (1) typical short life cycle (32 to 48 hours) of Bacillus spp.; (2) population growth in constrained environment may decline after 28 to 90 hours of incubation (Renganathan et al. Citation2011; Li et al. Citation2012). Furthermore, organic acid may precipitate and chelate with cation in the soil (Illmer & Schinner Citation1992). All these may contribute to the limitations of biofertilizer application of PSB.
Some studies showed that phosphate-solubilizing microorganisms (PSM), including fungi and bacteria, have only a limited capacity to solubilize FePO4 and AlPO4 (Banik & dey Citation1983; Whitelaw et al. Citation1999). Those studies suggested that where poorly soluble Fe-P and Al-P occurred in high amounts, the microbial-mediated mobilization of P might be less effective. This might be one of the reasons to explain the low fertility of red clay soil. However, B1 was very efficient in solubilizing AlPO4.
In each case, due to bacterial activity, lowering of pH coincided with increasing in the efficiency of phosphate-solubilizing activity. A maximum drop in pH was associated with a higher level of P solubilization, such as in B1, in which a large amount of P released from AlPO4 (321.12 mg L−1) was accompanied by a significant drop in pH. However, there was no significant correlation between pH and soluble P concentration (R 2 = 0.32, P > 0.05). This result agreed with some previous research (Lin et al. Citation2001; Jorquera et al. Citation2008).
The red clay soil is very barren and clay proportion is ≥40%, and the adsorption ability of soil P is very strong. It can hardly provide enough P for the growth of plants. Generally speaking, it is hard to judge which form of inorganic P is effective (for plant growth within) in red clay soil. In calcareous soil, Al-P and Fe-P were often treated as effective P for plant growth; and the soil Occluded-P was acknowledged as useless P (Shen & Jiang Citation1992). If the PSB strains could solubilize the soil Occluded-P, they should secrete organic acids to solubilize the iron and aluminum coating which surrounded the soil Occluded-P (Gong et al. Citation2010). In the present study, B1 could solubilize the soil Occluded-P by secreting organic acid, thus leading to the increase of soil Olsen-available P. This consequence was agreed with by Shi and Wu (Citation1998). Except Occluded-P, the soil Fe-P and Al-P also could be dissolved by organic acids (Jones & Darrah Citation1994; Dinkelaker et al. Citation1995; Iyamuremye et al. Citation1996; Luo et al. Citation1999).
The PSM strains have been studied for their phosphate-solubilizing activity and are often used as biofertilizers (Whitelaw et al. Citation1999; Singh & Reddy Citation2011). The hardly soluble phosphate can be changed by PSM into available phosphates for plant roots. As shown by Malboobi et al. (Citation2009), the competitiveness of PSBs which they screened could increase biomass production and yield of potato in both greenhouse and field trials. In the present study, we found that B1 could increase plant biomass and yield. Researchers observed that coinoculation of PSB and rhizobia could increase both growth and yield of legumes and also improved the absorption of nitrogen and P in plants (Bashan & Holguin Citation1997; Rodriguez & Fraga Citation1999; Rosas et al. Citation2006). El-Komy (Citation2005) demonstrated the beneficial influence of coinoculation of Azospirillum lipoferum and Bacillus megaterium for providing balanced nitrogen and P levels in wheat plants. Peanut (Arachis hypogaea L.) is an important cash and oil crop in low-hilly red soil region of South China and occupies 60–85% dry-farming area in the recent 20 years (Wang & Chen Citation2005). In this study, we found that the number of nodules per plant increased from 44.3 in the control treatment to 76.0 in the B1 treatment. It also revealed that nodule endophytes seemed to be an interesting group since the intimate link between B1 and the plant were thought to greatly improve the efficiency of the delivery of nutrients to the plant (Firáková et al. Citation2007; Hamdali et al. Citation2008).
Funding
This study was supported by the National Natural Science Foundation of China [grant number 41371263].
Additional information
Funding
References
- Abd-Alla MH. 1994. Phosphatases and the utilization of organic phosphorus by Rhizobium leguminosarum biovar viceae. Lett Appl Microbiol. 18:294–296. 10.1111/j.1472-765X.1994.tb00873.x
- Banik S, Dey BK. 1983. Alluvial soil microorganisms capable of utilizing insoluble aluminium phosphate as a sole source of phosphorus. Z Mikrobiol. 138:437–442.
- Bashan Y, Holguin G. 1997. Azospirillum-plant relationships: environmental and physiological advances (1990–1996). Can J Microbiol. 43:103–121. 10.1139/m97-015
- Chang SC, Jackson ML. 1957. Fractionation of soil phosphorus. Soil Sci. 84:133–144. 10.1097/00010694-195708000-00005
- Dinkelaker B, Hengeler C, Marschner H. 1995. Distribution and function of proteoid roots and other root clusters. Bot Acta. 108:183–200.
- El-Komy HM. 2005. Coimmobilization of Azospirillum lipoferum and Bacillus megaterium for successful phosphorus and nitrogen nutrition of wheat plants. Food Technol Biotech. 43:19–27.
- Fasim F, Ahmed N, Parsons R, Gadd GM. 2002. Solubilization of zinc salts by a bacterium isolated from the air environment of a tannery. FEMS Microbiol Lett. 213:1–6.
- Firáková S, Šturdíková M, Múčková M. 2007. Bioactive secondary metabolites produced by microorganisms associated with plants. Biologia. 62:251–257. 10.2478/s11756-007-0044-1
- Goldstein AH, Braverman K, Osorio N. 1999. Evidence for mutualism between a plant growing in a phosphate-limited desert environment and a mineral phosphate solubilizing (MPS) rhizobacterium. FEMS Microbiol Ecol. 30:295–300. 10.1111/j.1574-6941.1999.tb00657.x
- Gong S, Wang X, Zhang T, Li Q, Zhou J. 2010. Release of inorganic phosphorus from red soils induced by low molecular weight organic acids. Acta Pedologica Sinica. 47:692–697.
- Hamdali H, Hafidi M, Virolle MJ, Ouhdouch Y. 2008. Rock phosphate-solubilizing Actinomycetes: screening for plant growth-promoting activities. World J Microbiol Biotechnol. 24:2565–2575. 10.1007/s11274-008-9817-0
- Hao J, Hong J, Liu B, Zhang J. 2006. Isolation, screening and combination of highly-effective phosphorus solubilizing bacterial strains in calcareous soil. Chin J Appl Environ Biol. 12:404–408.
- Illmer P, Schinner F. 1992. Solubilization of inorganic phosphates by microorganisms isolated from forest soils. Soil Biol Biochem. 24:389–395. 10.1016/0038-0717(92)90199-8
- Iyamuremye F, Dick RP, Baham J. 1996. Organic amendments and phosphorus dynamics: Phosphorus speciation. Soil Sci. 161:444–451. 10.1097/00010694-199607000-00004
- Jackson ML. 1973. Soil chemical analysis. New Delhi: Prentice Hall of India. 372 pp.
- Jones DL, Darrah PR. 1994. Role of root derived organic acids in the mobilization of nutrients from the rhizosphere. Plant Soil. 112:19–30.
- Jorquera M, Hernández M, Rengel Z, Marschner P, Mora ML. 2008. Isolation of culturable phosphobacteria with both phytate-mineralization and phosphate-solubilization activity from the rhizosphere of plants grown in a volcanic soil. Biol Fert Soils. 44:1025–1034. 10.1007/s00374-008-0288-0
- Juhi Y, Swati Y, Samuel GS. 2011. Plant growth promotion in wheat crop under environmental condition by PSB as bio-fertilizer. Res J Agric Sci. 2:76–78.
- Li X, Ding X, Xia L, Sun Y, Yuan C, Yin J. 2012. Proteomic analysis of Bacillus thuringiensis strain 4.0718 at different growth phases. Sci World J. 2012:1–10.
- Lin QM, Wang H, Zhao XR, Zhao ZJ. 2001. Capacity of some bacteria and fungi in dissolving phosphate rock. Microbiology. 28:26–30.
- Lopez BR, Bashan Y, Bacilio M. 2011. Endophytic bacteria of Mammillaria fraileana, an endemic rock-colonizing cactus of the southern Sonoran Desert. Arch Microbiol. 193:527–541. 10.1007/s00203-011-0695-8
- Lou YS, Li ZP, Zhang TL. 2003. Carbon dioxide flux in a subtropical agricultural soil of china. Water Air Soil Poll. 1:281–293. 10.1023/A:1025727504841
- Lu RK. 2000. Agricultural chemical analysis of soil. Beijing: China Agricultural Science and Technology Press. pp. 431–433.
- Luo J, Ran W, Hu J, Yang X, Xu Y, Shen Q. 2010. Application of bio-organic fertilizer significantly affected fungal diversity of soils. Soil Sci Soc Am J. 74:2039–2048. 10.2136/sssaj2009.0437
- Luo HM, Watanabe T, Shinano T, Tadano T. 1999. Comparison of aluminum tolerance and phosphate absorption between rape (Brassica napus L.) and tomato (Lycopersicum esculentum Mill.) in relation to organic acid exudation. Soil Sci Plant Nutr. 45:897–907. 10.1080/00380768.1999.10414339
- Malboobi MA, Behbahani M, Madanin H, Owlia P, Deljou A,Yakhchali B, Moradi M, Hassanabadi H. 2009. Performance evaluation of potent phosphate solubilizing bacteria in potato rhizosphere. World J Microbiol Biotech. 25:1479–1484. 10.1007/s11274-009-0038-y
- Narsian V, Patel HH. 2000. Aspergillus aculeatus as a rock phosphate solubilizer. Soil Biol Biochem. 32:559–565. 10.1016/S0038-0717(99)00184-4
- Narsian V, Thakkar J, Patel HH. 1995. Mineral phosphate solubilization by Aspergillus aculeatus. Indian J Exp Microbiol. 33:91–93.
- Nautiyal CS. 1999. An efficient microbiological growth medium for screening phosphate solubilizing microorganisms. FEMS Microbiol Lett. 170:265–270. 10.1111/j.1574-6968.1999.tb13383.x
- Perez E, Sulbaran M, Ball MM, Yarzabal LA. 2007. Isolation and characterization of mineral phosphate-solubilizing bacteria naturally colonizing a limonitic crust in the south-eastern Venezuelan region. Soil Biol Biochem. 39:2905–2914. 10.1016/j.soilbio.2007.06.017
- Pikovskaya RI. 1948. Mobilization of phosphorus in soil in connection with vital activity of some microbial species. Mikrobiologiya. 17:362–370.
- Podile AR, Kishore GK. 2006. Plant growth-promoting rhizobacteria. In: Gnanamanickam S, editor. Plant-associated bacteria. Netherlands: Springer; p. 195–230.
- Renganathan K, Rathinam X, Danial M. 2011. Quick isolation and characterization of novel Bacillus thuringiensis strains from mosquito breeding sites in Malaysia. Emir J Food Agric. 23:17–26.
- Rodriguez H, Fraga R. 1999. Phosphate solubilizing bacteria and their role in plant growth promotion. Biotech Adv. 17:319–339. 10.1016/S0734-9750(99)00014-2
- Rohr M, Kubicek CP, Kominek J. 1983. Gluconic acid. In: Rehm HJ, Reed G, editor. Biotechnology III. Wienheim: Verlag Chemie; p. 456–465.
- Rosas, SB, Andres, JA, Rovera M, Nestor SC. 2006. Phosphate solubilizing Pseudomonas putida can influence the rhizobia legume symbiosis. Soil Biol Biochem. 38:3502–3505. 10.1016/j.soilbio.2006.05.008
- Shen R, Jiang B. 1992. The distribution and effectiveness of inorganic phosphorus classification in calcareous soil. Acta Pedologica Sinica. 29:80–85.
- Shi Y, Wu X. 1998. The influence of available phosphorus effectiveness in the red soil tea garden by phosphorus solubilizing microbial inoculum. Chin Tea. 3:38–39.
- Singh H, Reddy MS. 2011. Effect of inoculation with phosphate solubilizing fungus on growth and nutrient uptake of wheat and maize plants fertilized with rock phosphate in alkaline soils. Eur J Soil Biol. 47:30–34. 10.1016/j.ejsobi.2010.10.005
- Sundara B, Natarajan V, Hari K. 2002. Influence of phosphorus solubilizing bacteria on the changes in soil available phosphorus and sugarcane and sugar yields. Field Crops Res. 77:43–49. 10.1016/S0378-4290(02)00048-5
- Wang M, Chen X. 2005. Obstacle and countermeasure of sustainable high yield for peanut in low-hilly red soil region. J Pean Sci. 34:17–22.
- Watanabe FS, Olsen SR. 1965. Test of an ascorbic acid method for determining phosphorus in water and NaHCO3 extracts from soil. Soil Sci Soc Am J. 29:677–678. 10.2136/sssaj1965.03615995002900060025x
- Whitelaw MA, Harden TJ, Bender GL. 1997. Plant growth promotion of wheat inoculated with Penicillium radicum sp. nov. Aust J Soil Res. 35:291–300. 10.1071/S96040
- Whitelaw MA, Harden TJ, Helyar KR. 1999. Phosphate solubilisation in solution culture by the soil fungus Penicillium radicum. Soil Biol Biochem. 31:655–665. 10.1016/S0038-0717(98)00130-8
- Xiang H, Weng H, Kong X. 2003. Distribution of bound phosphorus in lateritic soils and their sensitivity to acidity. J Agro-Environ Sci. 22:138–141.
- Yi Y, Huang W, Ge Y. 2008. Exopolysaccharide: a novel important factor in the microbial dissolution of tricalcium phosphate. World J Microbiol Biotech. 24:1059–1065. 10.1007/s11274-007-9575-4
- Young CC. 1994. Selection and application of biofertilizers in Taiwan. FFTC. 141:1–9.
- Young CC, Chang CH, Chen LF, Chao CC. 1998. Characterization of the nitrogen fixing and ferric phosphate solubilizing bacteria isolated from Taiwan soil. J Chin Agric Chem. 36:201–210.
- Zhang HJ, Wei ZG, Zhao HY, Yang HX, Li HX, Hu F. 2009. Effects of low-molecular-weight organic acids on gadolinium accumulation and transportation in tomato plants. Biol Trace Elem Res. 127:81–93. 10.1007/s12011-008-8224-6
- Zhu F, Qu L, Hong X, Sun X. 2011. Isolation and characterization of a phosphate solubilizing halophilic bacterium kushneria sp. YCWA18 from Daqiao Saltern on the Coast of Yellow Sea of China. Evid Based Complement Alternat Med. 2011:6.