Abstract
To assess emergence time of shoots from roots of the perennial weed Sonchus arvensis as a function of root weight and soil temperature, we performed an experiment to which linear models were fitted. Root parts of three distinct initial weight classes were grown in pots in the dark at constant temperatures of 4, 8 and 18°C, respectively. During five harvest occasions, prior to or at shoot emergence, below-ground shoot length was measured. Root planting depth (3, 10 and 17 cm) did not influence shoot elongation rate. The below-ground shoot elongation rate for a given initial root-weight class was estimated from the observations to be constant with time, but to increase with temperature and initial root weight. By expressing shoot length for a given day as a linear function of the number of days from planting date, and elongation rate as a linear function of temperature, we calculated (1) the accumulated temperature-sum requirement for emergence, (2) emergence time for variable temperature conditions in a clay soil using soil temperature recordings at 5-cm depth for seven seasons in central Sweden and (3) the emergence time at three elevated temperature levels and initial root-weight classes. The accumulated temperature-sum requirements for below-ground shoots of S. arvensis to reach soil surface are independent of temperature regime for roots of a given initial weight but lower for heavier than lighter roots. The temperature limit for below-ground shoot elongation to occur is about 2.0–2.5°C. Between-year variations in temperature under field conditions cause larger variation to emergence time than initial root-weight differences. An average temperature increase of 3°C would cause an earlier emergence time, in the same range (about 2 weeks) as the difference between the earliest and latest year in the current weather conditions.
Introduction
Sonchus arvensis L. is a perennial weed that often occurs in annual crops sown in spring in the northern parts of Europe (Anbari et al. Citation2011). The weed species may cause severe crop yield losses and has become an increasing problem as the use of herbicides is reduced (Bacher et al. Citation1997; Salonen et al. Citation2001; Salonen & Hyvönen Citation2002; Brandsæter et al. Citation2010). To effectively control this weed with other measures, mainly mechanical, the predictions of the behaviour of the weed in relation to climate and the cultivated crop are essential. The competitiveness of the weed is determined by its life cycle as a whole, of which the emergence is a crucial part (Brandsæter et al. Citation2010).
An overall aim of the weather and management-driven modelling of growth and development of S. arvensis is to predict the timing of the life cycle events of the weed in a changing climate and how these can be altered by management options. As the length of future winter seasons will influence on the emergence time of S. arvensis (Verwijst et al. Citation2013), which is sensitive to mechanical control directly after emergence (Håkansson Citation1969), climate-based emergence predictions may enhance the timing of weed control. Parts of this life cycle have been examined in previous studies. Competition for light and nitrogen between S. arvensis and barley has been modelled and tested against observations (Eckersten et al. Citation2010; Citation2011), as well as respiration of the perennial roots during winter and sprouting in spring (Verwijst et al. Citation2013). To link those model approaches, experiments on the effects of root fragmentation on emergence and subsequent performance of S. arvensis have been performed (Anbari et al. Citation2011).
Shoot emergence during spring depends on several processes like root depletion, respiration and shoot elongation, which in turn depend on temperature and root dry matter resources. The question asked in this paper is then to what degree we can predict the emergence time (the time when a below-ground shoot of S. arvensis reaches the soil surface) as a function of observable parameters, like temperature, and factors that we can manipulate by management options, like root size or weight and root depth. As a base for these predictions, we need to develop functional relationships for the most central processes determining when below-ground shoots reach the soil surface (Håkansson Citation1969).
The dynamics of the carbohydrate reserves in the roots interact with the depth from which shoots emerge. Fykse (Citation1974) observed that short roots of S. arvensis produced more shoots per unit root length than long ones and that the emergence occurred earlier from roots at 5-cm depth than at 15 cm. Shoot emergence rates in terms of elongation per time unit, however, were not quantified in that study. With increasing depth the survival of sprouting shoots may decrease (Håkansson & Wallgren Citation1972; Gruzdev & Tulikov Citation1966). This implies that modelling shoot emergence as a part of the lifecycle might need to consider how the shoot emergence frequency over time is influenced by variation in root depth and root weight.
The resources for the underground shoot growth originate from depletion of root biomass. This is not explored explicitly in this paper, since the relation between root depletion and shoot length is likely to be complex. Instead, we test the simplified hypothesis that the soil temperature is the driving force for the processes which determine the elongation rate of below-ground shoots from the roots of S. arvensis. This temperature-dependent elongation rate is then hypothesised to differ among root-weight classes. We assume that the temperature influence on the shoot elongation rate, as derived from the experiment of a number of constant temperature treatments, also is valid for temperature regimes in between those observed in the experiment and for variable temperature conditions. The purpose of the current study is to (1) compare simulated time of emergence with observations of these experiments and (2) use the model to predict the time for when the below-ground shoots of S. arvensis reach the soil surface and start producing above ground shoots in spring under variable climatic conditions.
Materials and methods
Plant material and measurements
Roots of S. arvensis were collected in the autumn of 2005 from organically grown short-term ley fields with cereals in the rotation at Sala (59°40´N, 16°40´E) in central Sweden and planted in buckets filled with soil. This plant material was stored over winters in buckets, constituting a root bank, with a soil volume of 10 litre from October until June in a dark cold store at +2 to +4°C, and grown outdoors in the same buckets during the summers of 2006–2008 from June to October. These buckets were taken from the cold store and roots were replanted during June of each year and fertilised with about 70 kg N ha–1, while the soil was kept moist throughout the growing seasons.
On 29 June 2009, buckets were taken from the cold store and roots were distributed into three thickness categories: fine (<2 mm), medium (2–4 mm) and thick (>4 mm) in diameter. Roots of each category were cut in 5- and 10-cm pieces, respectively, with at least two adventitious buds, but no sprouted buds, on each piece. These root fragments were kept in the cold store until the following day, 30 June 2009, when 15 buckets with 5-cm root parts and 15 buckets with 10-cm root parts were placed in each of three dark chambers, with permanently controlled temperatures of 4, 8 and 18°C (±0.1°C), respectively. The soil in each of the 90 buckets (5 litres per bucket) consisted of 85% moderately decomposed peat, 15% sand and about 1 g N per bucket, given as a compound fertiliser with an NPK proportion of 2:1:2 (Hasselfors Garden, Sweden). For each temperature level and root length, 81 roots were planted, so that three roots (one root for each thickness category) were present in each layer at a depth of 3, 10 or 17 cm below soil surface (all together 27 roots × 3 replicates = 81 roots per temperature level and root length, in total 486 roots), as described in . Each root was tagged with a number and fresh weight was measured. As emergence time is known to be negatively correlated with planting depth (Håkansson & Wallgren Citation1972), this design, which employs a wider depth range, was expected to give a longer time period over which shoots, before reaching the soil surface, could be harvested. All buckets were sealed with plastic and kept moist throughout the experiment.
Table 1. Harvest occasion, planting depths within bucket, number of roots per bucket and harvest day after planting for each of the temperature regimes (4, 8, and 18°C).
To determine the ratio between dry weight and fresh weight of the roots, 10 roots (in addition to those planted) were randomly selected from each of the three thickness categories and of the 5- and 10-cm length categories, in total N = 60. After measuring fresh weight, the roots were dried at 50°C to constant weight (c. 3 days), and individual root dry matter content was determined. As neither length nor thickness had a significant effect on the dry to fresh weight of the roots, the average value (0.178) was used to estimate root dry weight at planting (Verwijst et al. Citation2013).
The 486 roots were ranked according to their initial weights and separated into three, non-overlapping weight classes, each containing 162 roots. This gave three classes with mean root dry weights of 0.044, 0.079 and 0.163 g, respectively.
Harvest was performed per temperature level and replicate (consisting of three buckets) just after the first emergence of the first shoot per temperature level and replicate, and the length of the longest shoot per root was measured. Time (days) from planting until harvest was recorded for each of the buckets. This resulted in five harvest occasions per temperature treatment ().
Model description
Shoots are defined to emerge when the length of the longest shoot per root becomes larger than the depth of the roots from which the shoots originated. Shoot elongation is theoretically expected to depend on root depletion (see further the Discussion section), which is considered in this study by treating each temperature level and root-weight class separately.
The elongation rate of the below-ground shoots, for a given root-weight class is, in agreement with the observations, regarded to be constant (a, mm day–1) with time. Shoot length (mm) for a given day (t) is then an increasing linear function of the number of days since the day (t o) when roots were planted, and b is an offset (mm) related to the fact that the beginning of shoot elongation may be delayed in relation to the planting of the roots.
The elongation rate (a) was found to be higher in the high-temperature treatment, and a linear function was used to express its dependency on temperature (T).
where c (mm day–1 °C–1) is the change of shoot elongation rate per degree Celsius, and d (mm day–1) an offset.
Model applications
The model parameters (a, b, c and d) were determined by calibration of Equations 1–2 to the data from the bucket experiment, i.e. for all combinations of the three root-weight classes and constant temperatures (+4, +8, +18°C, respectively) and roots planted at different depths.
Thereafter, the model (Equation 1) was used to calculate shoot length for variable temperature conditions in a clay soil using soil temperature recordings at 5-cm depth (daily average values of hourly records) from the Ultuna meteorological station (Karlsson & Fagerberg Citation1995) close to Uppsala (58°40´N, 17°39´E) for each growing season during 2004–2010. The emergence time was calculated as the time it takes (t–t 0) before shoot length equals 10 cm, representing emergence time for roots situated at 10-cm depth, and setting t 0 to be 1st March. The soil temperature at 5-cm depth for the 1st of March was on average 0.3°C for 2004–2010 and varied between –0.5 and 1.0°C, and it was assumed that shoot sprouting from root buds had not started. Sensitivity analyses were performed with regard to the effects of temperature variability during the seven seasons, three temperature elevation levels, and initial root-weight classes, on emergence time.
Results
Modelling shoot length
The shoot elongation rate of the first shoot (a in Equation 1), expressed as a mean per root-weight class and temperature treatment, was estimated by performing a regression analysis, using the observed mean length of the first shoot as the dependent variable and time between planting and harvest as the independent variable (Equation 1). The temperature effect on the elongation rate was linear, which in turn was dependent on root-weight class ().
Table 2. Slope (a), intercept (b) and R 2 for the linear regression of shoot length on time since planting (Equation 1) for each root-weight class and temperature.
The delay in the onset of below-ground shoot elongation in relation to the time of planting (t 0) of the root pieces was larger for the low-temperature treatment. However, within temperature treatments, delay did not vary consistently as a function of root-weight class (). The negative values of the intercept (b) are partly due to this delay. The shoot elongation rate (a) increases with root-weight class and temperature. The temperature dependence of these regressions was estimated by calculating the linear regression between the mean elongation rate and temperature ().
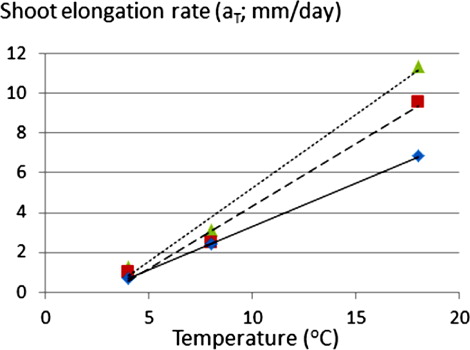
The time required (t–t o in Equation 1) for the first shoot to become 10 cm (assumed to represent emergence time of roots at 10-cm depth) was estimated for different constant temperature regimes above or equal to 4°C. This was done by setting shoot length equal to 100 mm in Equation 1 and replacing a with a T calculated according to Equation 2. At low temperatures, the time to emergence is long (2–3 months at 5°C) and then becomes smaller at higher temperatures when shoot elongation rate is high (3–4 weeks at 10°C). The influence of initial root weight on emergence time is larger at low temperatures, e.g. at 5°C the shoots of the light roots emerge a month later than those of the heavy roots, whereas at 10°C this difference is only a week ().
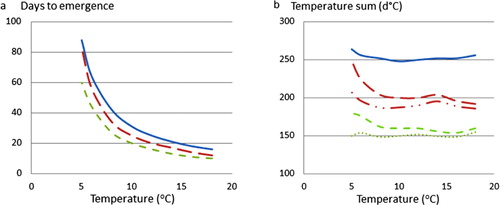
From , we calculated the accumulated temperature-sum requirement for the below-ground shoots to reach the soil surface, by taking the temperature (x values) times the number of days to emergence (y values). The temperature-sum requirement for emergence decreased strongly with increased root weight but was basically independent of temperature, although with different threshold temperatures (). For the light root-weight class a threshold of 2.0°C (T base) gave a constant value of 254 d°C (T sum). For the intermediate and heavy root-weight classes the temperature-sum became almost independent of temperature treatment (T sum is 192 and 151 d°C, respectively) using a threshold of 2.5°C (). The day of emergence (t e) could thus be formulated as the day when the accumulated sum of temperatures above T base (°C) becomes equal to T sum (d°C).
Shoot length predicted for climatic temperature variability
Observed soil temperatures at 5-cm depth at Ultuna climate station during the spring of seven years (2004–2010) varied by approximately 4°C between years for a given date (). This variation in temperature resulted in that the largest roots at depth 10 cm emerged on day number 129 (May 9) in the earliest spring (2007), whereas for the latest springs (2005 and 2006) the shoots were only half way (5 cm) to soil surface and emerged almost 2 weeks later on day number 142 ().
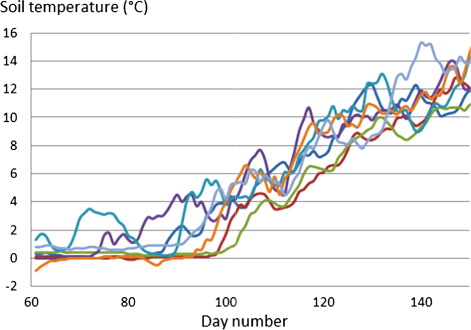
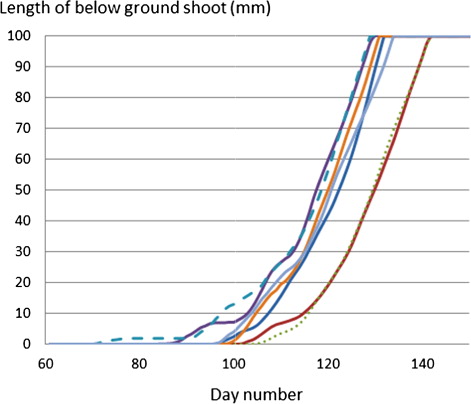
This variation between years was larger than the variation in emergence time due to different initial root weights which for one of the years (2005) was 9 days earlier for the heavy roots compared with the light roots. Increasing the average daily values of the soil temperature by 2, 4 and 6°C, to roughly mimic potential temperature changes corresponding to future projected climate change scenarios, shortened the calculated emergence time by 8, 19 and 30 days, respectively, for the lightest roots, and 1–2 days less for the heavy roots, compared to the year 2008 ().
Table 3. Time of emergence response to changes (Δ) of temperature and/or root weight.
Discussion
The main result of this study concerns the gain in (shorter) emergence time in response to increased temperature () and that this response could be expressed by a temperature sum that is specific for each root-weight category (; Equation 3). The analysis also proposes that the threshold temperature above which shoot elongation starts is 2.0–2.5°C. The rate of shoot elongation at 18°C ranged between 0.7 and 1.2 cm day–1 (), which agrees well with observations of other studies of Cirsium arvense (Sciegienka et al. Citation2011).
It is a common way to predict emergence of shoots from roots by relating the underground shoot length to a temperature sum (Hacault & Van Acker Citation2006), but it has been claimed that this approach has limitations in predictability (Donald Citation2000). Our approach did not assume a priori that the temperature-sum requirement is fixed. Instead we allowed the temperature requirement to vary both with temperature and root weight. The result was then that the temperature-sum requirement for emergence was found to decrease strongly with increased root weight but was basically independent of temperature regime.
The estimated mean emergence day over the years 2004–2010 for large roots was day 135 (May 15; Figure 4), for shoots originating from roots assumed to be planted at 10-cm depth. A 5-cm planting depth might be a more representative depth of weed roots under field conditions (Lemna & Messersmith Citation1990). The shoot elongation rate was derived for roots planted at different depths (); however, no significant relation between shoot elongation rate and planting depth could be detected (data not shown). Hence roots at 5-cm depth would, statistically, have an emerging time half of those at 10-cm depth, in case temperature is the same at the different depths. In spring, though, soil temperature rises in the top layers first, hence the emergence time of the roots at 5 cm would be even shorter. Our results also suggest that no shoot growth will take place before the soil temperature has risen above 2°C.
The ecological significance of these results depends on a variety of circumstances, but a deeper analysis of such responses is beyond the scope of this paper. However, the fact that the heavier roots had a higher below-ground shoot elongation rate ( and ), and an earlier emergence time than lighter roots (, ) might be explained by a larger depletion of heavier roots with relatively larger amount of stored carbohydrates and proteins versus lignified tissues. The higher the content of stored nutrition, the higher the depletion rate (Guncan Citation1973; Anbari et al. Citation2011). It remains to be analysed to what extent the different shoot elongation rate responses to temperature among root-weight categories can be expressed as function of root depletion rate, larger roots having a higher depletion rate than smaller roots. A higher degree of root fragmentation would, according this study, delay the emergence of S. arvensis. A potential effective weed control strategy might be that weed control focuses on fragmenting the weed during the root storing period towards the end of the growing season. In line with this, disturbance strategies have focused on the compensation point, the stage at which the below-ground biomass is at minimum (Håkansson Citation1969; Tavaziva Citation2012). Further mechanical treatments in spring prior to sowing the crop would utterly deplete the root system of S. arvensis.
Conclusions
The main conclusion from this study is that the shoot elongation rate increases with temperature, and that for each root-weight category the temperature-sum requirements for the roots to reach soil surface and emerge are independent of temperature regime, but are lower for heavier than lighter roots. We also conclude that the temperature limit for below-ground shoot growth to occur is about 2.0–2.5°C. Our analysis indicates that between-year variation in temperature under field conditions (in central Sweden) causes larger variation to emergence time than the difference in root weight. It also indicates that an average increase of temperature climate by about three degree Celsius would cause an earlier emergence time, about equal to the difference between the earliest and latest year in the current climate; however, a more comprehensive quantification would need essentially more weather conditions to be examined (cf. Stratonovitch et al. Citation2012).
Disclosure statement
No potential conflict of interest was reported by the authors.
Additional information
Funding
References
- Anbari S, Lundkvist A, Verwijst T. 2011. Sprouting and shoot development of Sonchus arvensis in relation to initial root size. Weed Res. 51:142–150.
- Bacher S, Heizmann A, Nentwig W. 1997. Problemunkräuter in ökologischen Ausgleichsflächen im Ackerbau. Agrarforschung. 4:91–94.
- Brandsæter LO, Fogelfors H, Fykse H, Graglia E, Jensen RK, Melander B, Salonen J, Vanhala P. 2010. Seasonal restrictions of bud growth on roots of Cirsium arvense and Sonchus arvensis and rhizomes of Elymus repens. Weed Res. 50:102–109.
- Donald WW. 2000. A degree-day model of Cirsium arvense shoot emergence from adventitious root buds in spring. Weed Sci. 48:333–341.
- Eckersten H, Lundkvist A, Torssell B. 2010. Comparison of monocultures of perennial sow-thistle and spring barley in estimated shoot radiation-use and nitrogen-uptake efficiencies. Acta Agric Scand, Sect B – Soil & Plant Sci. 60:126–135.
- Eckersten H, Lundkvist A, Torssell B, Verwijst T. 2011. Modelling species competition in mixtures of perennial sow-thistle and spring barley based on shoot radiation use efficiency. Acta Agric Scand, Sect B – Soil & Plant Sci. 61:739–746.
- Fykse H. 1974. Research into Sonchus arvensis L.: II. Distribution in Norway, growth and dormancy in comparison with similar species. Statens plantevern, Ugrasbiologisk avdeling, Særtrykk. 115:389–412.
- Gruzdev G, Tulikov A. 1966. Peculiarities of the vegetative reproduction of yellow sowthistle (Sonchus arvensis) and creeping thistle (Cirsium setosum M.B.). Izvestiya Timiryazevskoi Sel"skokhozyaistvennoi Akademii. 6:83–95.
- Guncan A. 1973. Carbohydrate reserves of Sonchus arvensis rhizomes during a vegetative season and comparison with Convolvulus arvensis L. Mededelingen Fakulteit Landbouwwetenschappen Gent. 38:1011–1017.
- Hacault KM, Van Acker RC. 2006. Emergence timing and control of dandelion (Taraxacum officinale) in spring wheat. Weed Sci. 54:172–181.
- Håkansson S. 1969. Experiments with Sonchus arvensis L. I. Development and growth in response to burial and defoliation in different developmental stages. Ann Agric Coll Sweden. 35:989–1030.
- Håkansson S, Wallgren B. 1972. Experiments with Sonchus arvensis L. 3. The development from reproductive roots cut into different lengths and planted at different depths, with and without competition from barley. Swed J Agric Res. 2:15–26.
- Karlsson S, Fagerberg B. 1995. Climate and bioclimate Station at Ultuna. Uppsala: Swedish University of Agricultural Sciences, Department of Crop Production Science. Sweden.
- Lemna WK, Messersmith CG. 1990. The biology of Canadian weeds. 94. Sonchus arvensis L. Can J Plant Sci. 70:509–532.
- Salonen J, Hyvönen T. 2002. Perennial weeds in conventional and organic cropping of spring cereals in Finland. Zeitschrift Pflanzenkrankheiten Pflanzenschutz. 18:519–525.
- Salonen J, Hyvönen T, Jalli H. 2001. Weed flora in organically grown spring cereals in Finland. Agric Food Sci Finland. 10:231–242.
- Sciegienka J, Keren E, Menalled F. 2011. Impact of root fragment dimension, weight, burial depth, and water regime on Cirsium arvense emergence and growth. Can J Plant Sci. 91:1027–1036.
- Stratonovitch P, Storkey J, Semenov MA. 2012. A process-based approach to modelling impacts of climate change on the damage niche of an agricultural weed. Global Change Biol. 18:2071–2080.
- Tavaziva VJ. 2012. Effects of competition on compensation point and phenological development in Sonchus arvensis L. [Master thesis]. Uppsala: Swedish University of Agricultural Sciences. http://stud.epsilon.slu.se/4572/.
- Verwijst T, Eckersten H, Anbari S, Lundkvist A, Torssell B. 2013. Weight loss in overwintering below-ground parts of Sonchus arvensis under current and temperature elevated climate scenarios in Sweden. Weed Res. 35:21–29.