ABSTRACT
A study was conducted to investigate the sensitivity of physiological traits under different soil water availability in summer maize (Zea mays L.). Plant growth (plant height and stem), water relations (relative water content), gas exchange (net photosynthesis rate, stomatal conductance and transpiration rate) and nutrient levels were measured after 5, 10, 15 and 20 days of drought with different soil water availability. We found that all these physiological traits were inhibited by drought stress, but they showed differing levels of sensitivity with different soil water availability. Our results show that stomatal conductance was the most sensitive in mild drought conditions, that carbon accumulation was more sensitive than the other parameters in moderate drought, and that height and stem showed the largest response ratio in severe drought conditions. Different traits performed differently under drought conditions; thus, choosing an adequate index for evaluating soil water availability is necessary for maize cultivation.
Introduction
Soil water deficits are often the greatest constraint in plant growth (Wu et al. Citation2011a). With global climate change, varying rainfall patterns make droughts more severe and frequent (Easterling et al. Citation2000), and models predict a 25% decrease in soil moisture between 2000 and 2040 (Sabaté et al. Citation2002), which will bring great challenges because almost 66% of total cereal crop production is by rain-fed agriculture (Lobell et al. Citation2014).
Drought affects plant growth, photosynthetic activity and yield (Praba et al. Citation2009). The sensitivity of plants to drought varies based on the degree of the drought stress, accompanying stress, plant species and developmental stage (Demirevska et al. Citation2009). Many studies have reported a reduction in growth that was related to chemical signals resulting from water deficits (Ismail et al. Citation1994; Ismail & Davies Citation1998; Hurley & Rowarth Citation1999). A common adverse effect of drought on plants is the reduction of fresh and dry biomass (Zhao et al. Citation2006); maize height and stem diameter noticeably decreased with increasing drought stress (Zhao et al. Citation2006; Demirevska et al. Citation2009). Another major effect is a reduction in photosynthesis, which is caused by decreased leaf expansion and impaired photosynthetic machinery (Wahid et al. Citation2005). In addition, drought can affect the photosynthetic pigments (Anjum et al. Citation2003) and reduce the relative water content (RWC), which has been noted in a wide variety of plants (Nayyar & Gupta Citation2006).
Carbon (C), nitrogen (N), phosphorus (P) and potassium (K) are considered essential elements for plants and play important roles in plant functions (Marschner Citation2012). Drought can affect the assimilation of C, which, as the structural basis of the plant, constitutes 50% of its dry mass. N is an important constituent of proteins and plays an essential role in all enzymatic activities, and P is involved in energy transfer in cells. Additionally, P and N are important structural elements of nucleic acids (Ågren Citation2008). These three elements are strongly linked in terms of their biochemical functions. K is involved in the plant–water relationship (Babita et al. Citation2010) and functions in plant osmotic control and the improvement of stomatal function (Sangakkara et al. Citation2000; Babita et al. Citation2010; Laus et al. Citation2011). Plants under drought generally reduce their nutrient uptake, which may be diminished because of interference of reduced transpiration (Farooq et al. Citation2009).
Many studies have addressed the effects of soil water availability on plant growth and photosynthesis, which show a strong synergic correlation (Chaves et al. Citation2002; Efeoğlu et al. Citation2009). However, because different physiological traits have different sensitivities (Wu et al. Citation2011b), understanding their different sensitivities could help us judge whether a plant is suffering drought stress. Unfortunately, information about those sensitivities remains scarce (Wu et al. Citation2011a, Citation2015). Maize is the third important food crop in the world (Naveed et al. Citation2014) and is planted in large areas in summer in China; thus, it is necessary to identify an adequate index for evaluating the soil water availability for maize. To more fully understand the response of maize to different soil water availability, the objectives of this study were to investigate the changes in various physiological traits (morphology, water relation, gas exchanges and nutrient absorption) in maize under different soil water availability conditions and to clearly establish the best evaluation index for judging whether the maize is suffering from drought stress, which could guide summer maize production when facing drought stress.
Materials and methods
Experimental site
The study was conducted at the Institute of Soil and Water Conservation, in the Northwest A & F University, in Yangling, Shaanxi. The maize species was cv. Zhengnong 7278. Soil for the experiment was collected from a layer of 0 to 20 cm deep. The field capacity was 0.287 m3 m−3, which was determined using the modified Wilcox method, and the permanent wilting point value was 0.122 m3 m−3, taken from Wu et al. (Citation2011c).
Experimental design
In 2014, a group of pots for maize was placed under an open-sided greenhouse with a glass roof. Each pot was packed with 16 kg of soil. A small tube (2 cm in diameter) at the bottom was used to supply water and oxygen to the soil. One gram of urea and 16 g of NPK fertilizer (18–16–16%) were mixed with the soil before packing, and covered with perlite (60 g) to reduce soil evaporation. Maize seeds were sown on 20 June , and three plants were retained in each pot. Seven treatments (40%, 47%, 55%, 65%, 75%, 85% and 95% soil water availability), with 12 replicates with maize, as well as three replicates without plants to measure the soil evaporation, were established in a randomized block design. At first, all plants were well-watered, and then different water treatments were performed by drying or adding water to reach the intended water content, which was determined by weighing. Once each treatment was established, it was maintained by adding water equal to transpiration through the tube inserted into each pot once or twice daily based on the weather and plant growth, until the experiment was finished.
Measurement
Plants were measured every five days after treatment. The plant height was measured from the soil surface to the highest leaf sheath using a ruler with 1-mm markings, and the stem diameter was measured 5 cm above the soil surface using a Vernier caliper with 0.01-mm accuracy. There were nine measurements per treatment. The third fully expanded leaf from the top was used for measuring leaf gas exchange, chlorophyll fluorescence and chlorophyll content. Leaf gas exchange, including the photosynthetic rate (Pn), stomatal conductance (Gs) and transpiration rate (Tr), was measured with a Li-6400 (Li-Cor model 6400, Lincoln, Neb, USA) on two occasions between 10:00 and 11:00. Environmental conditions in the leaf chamber consisted of a saturating photosynthetic photon flux density of 1500 μmol m–2 s–1 (with 10% blue light), a vapor pressure deficit of 2.0–3.0 kPa, an air temperature of 32°C and an ambient CO2 concentration (Ca) of 400 μmol mol–1 air. The Fv/Fm and ФPSII were calculated from chlorophyll fluorescence data using a Handy PEA (FMS-2.02, Hansatech Instruments, England). The leaves were dark-adapted for 30 min using Handy PEA leaf clips, and the flux density of incident photosynthetically active radiation was 5000 μmol m–2 s–1. ФPSII was determined by measuring steady-state fluorescence (Fs) and maximum fluorescence during a light-saturating pulse of 5000 μmol m–2 s–1 (Fm'), and ФPSII = (Fm'–Fs)/Fm'. The leaf chlorophyll content was measured using a SPAD 502 Plus Chlorophyll Meter (Minolta, Japan); each leaf was measured in at least three different areas, and a total of nine plants per treatment were measured. After gas exchange, chlorophyll fluorescence and chlorophyll content measurements, leaf discs from the same leaves were sampled. Leaf RWC was determined in these leaf discs as follows: RWC (%) = (Fresh weight−Dry weight)/(Fully turgid weight−Dry weight) × 100. Fully turgid weight was determined by placing samples in distilled water and maintaining them at 4°C in darkness until they reached a constant weight (12 h). Dry weight was obtained after placing the samples in an oven at 75°C for 48 h.
Plants were harvested when the physiological measurements were finished, and they were oven-dried at 105°C for 30 min and then at 75°C to a constant mass. All of the samples were ground into uniformly fine powder and sieved through a 1-mm mesh screen. The C, N, P and K contents were assayed according to Bao (Citation2000).
Calculations and statistics
Because of a change in the units used, the sensitivity of the physiological traits to soil water availability was analyzed using linear regression, and the different b-values reflect this change. In the linear-regression analysis, we used the normalized data for the different physiological traits, and the b-values were calculated using the following equation:(1) where y represents the physiological traits and x represents the soil water availability.
The sensitivity of each index was detected by the response ratio (RR), which reflected changes between 95% (XC) and other treatments and was defined as the ratio of the mean value of a given variable in the experimental treatment to that in the control treatment (95%) and calculated using the following equation:(2) where XE is the experimental treatment.
One-way ANOVAs were performed to test for statistical significance, and significant differences were evaluated at the 95% confidence level. We used Pearson's correlation coefficients to test for associations between the soil water availability and the other traits. All the statistical analyses were performed using SPSS software ver. 17.0 (SPSS Inc., Chicago, IL, USA).
Results
Total transpiration, RWC and growth changes of maize under different soil water availability
Low soil water availability induced significant reductions in total transpiration, RWC and growth of maize. As soil water availability increased, these parameters increased significantly (), and they performed the best in the 85% treatment. A stronger reduction was observed after 20 days of water stress, with total transpiration, height and stem increment, respectively, reduced by 85.9%, 77.8% and 69.8% under the 40% condition compared with the 85% condition.
Changes in leaf gas exchange and fluorescence under different soil water availability
The Pn, Gs and Tr significantly increased with increasing soil water availability, showing their highest values in the 85% group and at 20 d ((a)–2(c)), which indicated that leaf gas exchange was strongly inhibited by low soil water availability. The chlorophyll content was only significantly reduced in severely stressed plants ((d)). The Fv/Fm was only reduced in the 40% and 47% conditions at 5 d and 20 d, and no significant difference was observed among the other treatments ((e)); further, the ФPSII was significantly lower in the 40% treatment ((f)), showing that the fluorescence did not significantly change when suffering a moderate shortage in soil water availability.
Figure 2. Leaf gas exchange and fluorescence under different soil water availability. (a) Net photosynthesis (Pn); (b) stomatal conductance (Gs); (c) transpiration rate (Tr); (d) chlorophyll content (Chl); (e) the maximum quantum yield of PSII photochemistry (Fv/Fm); (f) the effective quantum yield of PSII photochemistry (ФPSII). Lowercase letters indicate statistical significance in different soil water availability treatments at P < .05.
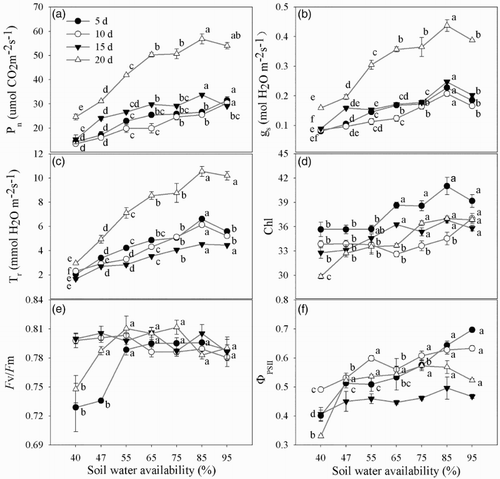
Nutrient accumulation changes of maize under different soil water availability
Increases in growth and in C, N, P and K accumulation were observed with increasing soil water availability in maize (). Compared with the 85% treatment, after 20 days of water stress, the C, N, P and K accumulation of the plants were reduced by 67.6%, 62.7%, 67.2% and 63.5% in 40% water treatments, respectively. However, the C, N, P and K levels decreased with increasing soil water availability.
Sensitivity evaluation and RRs of maize growth, gas exchange and nutrient accumulation under different soil water availability
The growth, gas exchange and nutrient accumulation of maize showed a highly significant correlation with the soil water availability (). The largest b-value was observed for the interaction between the height increment and soil water availability, indicating that height was sensitive to reductions in soil water availability, whereas RWC showed the smallest change.
Table 1. The b-values in the linear-regression analysis between physiological traits and soil water availability.
The RRs of maize RWC, growth, gas exchange and nutrient accumulation were different compared to 95% treatment (). Most indices in the 95% condition were lower than in the 85% treatment, except for RWC; thus, the RR in the 85% treatment was positive, which indicates that 85% water was the best water treatment. Thus, further analyses only consider the RR in the 75–40% water treatments compared with CK. In one column, all indices showed higher RRs in the 40–55% water treatments. As water availability decreased, the absolute value of RR in RWC showed a significant increase in the 47% treatment, and maize growth (Hi and Si) and nutrition accumulation (C, N, P and K) showed significant increases in 55% water treatment. The leaf gas exchange indices Pn and Tr showed significant changes with 65% treatment. Within the 75% and 65% water treatments, the absolute values of RR in Gs were significantly higher than other parameters. As water availability decreased, the RR of C accumulation remained significantly higher than other traits in 55% and 47% treatment. However, in the lowest water treatment, 40% water availability, maize growth index (Hi and Si) showed higher RR values.
Table 2. RRs of plant growth, gas exchange and nutrient accumulation under different water treatments.
Discussion
Drought affects the metabolic and physiological processes of plants, which are largely inhibited during moderate and severe drought stress. In China, water deficit, which is generally associated with high temperature, is the main limiting factor for maize growth. With decreasing soil water availability, the growth, gas exchange and nutrient accumulation were significantly inhibited (–), and the height showed the largest response in the study (). Under low soil water availability, Pn, Gs and Tr significantly decreased, leading to decreased assimilation of C and nutrients, ultimately affecting the crop yield. Thus, effective methods for judging whether maize is suffering drought stress have become very important. However, numerous studies show that all these physiological indicators decreased synergistically with each other, which was also confirmed by our results. RWC, transpiration and leaf temperature are important characteristics under drought stress (Farooq et al. Citation2009), and Sinclair and Ludlow (Citation1985) proposed that RWC was a better indicator than water potential. The results of that study showed that RWC was more sensitive to severe drought (), which was supported by Siddique et al. (Citation2000), who reported that only severe drought significantly decreased RWC to reduce water loss. However, compared with other trials, the RR reported from that study was lower in both mild and severe drought stress, which indicates that although RWC is an important indicator for judging plant–water stress, it is not the most sensitive index.
There was no surprise that Pn significantly decreased under low soil water availability, possibly due to stomatal closure and leaf rolling. To reduce water loss, the first responses of the plant were stomatal closure and decreased Pn and Tr ((a)–2(c)). These results were supported by the observation of the highest RR of Gs in the 75% and 65% water treatments (), which indicates that when maize is subjected to mild drought stress, Gs was generally decreased before other traits. Thus, Gs may be an early index to show whether the maize suffered drought stress. As soil water availability continued to decrease, low soil water availability produced changes in the photosynthetic components and decreased the chlorophyll content (Guerfel et al. Citation2009) ((d)). Chlorophyll content continuously declined with the duration of the stress, which might be caused by chloroplast damage induced by reactive oxygen species (Terzi et al. Citation2010). However, Fv/Fm showed no differences among the other treatments in the present study, except in 40% and 47% soil water availability at 5 d and 20 d ((e)), which indicates that the maximal efficiency of PSII was unaffected by mild water stress, consistent with the work of Shangguan et al. (Citation2000), who reported that mild drought did not influence Fv/Fm. ФPSII only showed a significant decrease in 40% soil water availability ((f)), indicating that severe drought affected the effective quantum yield of PSII photochemistry, consistent with Nar et al. (Citation2009), who found that ФPSII did not decrease until the RWC of the leaf decreased to 79%. In the present study, ФPSII also only decreased in the 40% condition, likely because of a decrease in leaf area caused by leaf rolling, which may protect the plant from photodamage (Nar et al. Citation2009). A major effect of drought was photosynthesis reduction, which arises from a decrease in leaf expansion and from impaired photosynthetic machinery (Farooq et al. Citation2009).
C, N, P and K are considered essential elements for plant growth, and they play important roles in plant functions. Importantly, low soil water availability affected the acquisition of nutrients by the roots by reducing the transpiration rates and impairing active transport (Hu et al. Citation2007). The present study showed that the absorption of N, P and K was significantly affected by low soil water availability ((b)–3(d)); C accumulation was also affected ((a)), primarily due to reduced photosynthesis ((a)). Low soil water availability decreased the Pn, which affected the C assimilation, influenced plant growth and led to decreased accumulation of other basic nutrients (N, P and K). Decreased Pn suppresses the absorption of these nutrients; thus, the RR values for C, N, P and K were higher in moderate drought stress (). C accumulation showed the highest RR values in the 55% and 47% water treatments, indicating that C accumulation may be a good indicator of moderate drought stress. The average RR of N accumulation was higher than those for P and K, indicating that deficiencies in N are more likely in low soil water availability, which may indicate that increased N availability could alleviate the effects of drought (Zhong et al. Citation2014). However, the C, N, P and K contents decreased with increasing soil water availability and plant growth (), suggesting that the slower growth of plants experiencing low soil water availability might prevent nutrients from being diluted and indicating that the reduction in nutrient absorption under drought conditions was lower than the increase in plant biomass. In addition, a decline in soil water availability also resulted in a decrease in the diffusion rate of nutrients from the soil to the root surface (Alam and Pessarakli Citation1999). A reduction in the energy supply to the roots caused by a reduction in photosynthesis would also affect the absorption of nutrients (Aroca Citation2012).
The growth, photosynthesis and nutrient absorption of maize decrease under low soil water availability, but the sensitivity of these traits varied under different soil water availability treatments (). Our results showed the highest RR values for Hi and Si in the lowest soil water availability (40%). Hi and Si are easily detected indices in the field, but they cannot be used as earlier indicators of whether maize suffered drought stress. Further, Gs was the most sensitive indicator of decreased soil water availability and showed the largest RR value in mild and moderate drought stress, and C accumulation is more sensitive than other indicators for moderate drought stress. Different physiological traits are affected differently by decreases in the soil water availability; therefore, it is crucial to choose an adequate index for evaluating soil water availability.
Disclosure statement
No potential conflict of interest was reported by the authors.
Additional information
Funding
References
- Ågren GI. 2008. Stoichiometry and nutrition of plant growth in natural communities. Annu Rev Ecol Evol S. 39:153–170. doi: 10.1146/annurev.ecolsys.39.110707.173515
- Alam SM, Pessarakli, M. 1999. Nutrient uptake by plants under stress conditions. Handbook of plant and crop stress. 2:285–313.
- Anjum F, Yaseen M, Rasul E, Wahid A, Anjum S. 2003. Water stress in barley (Hordeum vulgare L.). Pak J Agr Sci. 40:45–49.
- Aroca R. 2012. Plant responses to drought stress: from morphological to molecular features. Berlin Heidelberg: Springer.
- Babita M, Maheswari M, Rao L, Shanker AK, Rao DG. 2010. Osmotic adjustment, drought tolerance and yield in castor (Ricinus communis L.) hybrids. Environ Exp Bot. 69:243–249. doi: 10.1016/j.envexpbot.2010.05.006
- Bao SD. 2000. Soil and agricultural chemistry analysis. Beijing: Agriculture Press of China.
- Chaves MM, Pereira JS, Maroco J, Rodrigues ML, Ricardo CPP, Osório, ML, Pinheiro C. 2002. How plants cope with water stress in the field? Photosynthesis and growth. Ann Bot-London. 89:907–916. doi: 10.1093/aob/mcf105
- Demirevska K, Zasheva D, Dimitrov R, Simova-Stoilova L, Stamenova M, Feller U. 2009. Drought stress effects on Rubisco in wheat: changes in the Rubisco large subunit. Acta Physiol Plant. 31:1129–1138. doi: 10.1007/s11738-009-0331-2
- Easterling DR, Meehl GA, Parmesan C, Changnon SA, Karl TR, Mearns LO. 2000. Climate extremes: observations, modeling, and impacts. Science. 289:2068–2074. doi: 10.1126/science.289.5487.2068
- Efeoğlu B, Ekmekci Y, Cicek N. 2009. Physiological responses of three maize cultivars to drought stress and recovery. S Afr J Bot. 75:34–42. doi: 10.1016/j.sajb.2008.06.005
- Farooq M, Wahid A, Kobayashi N, Fujita D, Basra SMA. 2009. Plant drought stress: Effects, mechanisms and management. Agron Sustain Dev. 29:185–212. doi: 10.1051/agro:2008021
- Guerfel M, Baccouri O, Boujnah D, Chaïbi W, Zarrouk M. 2009. Impacts of water stress on gas exchange, water relations, chlorophyll content and leaf structure in the two main Tunisian olive (Olea europaea L.) cultivars. Sci Hortic-Amsterdam. 119:257–263. doi: 10.1016/j.scienta.2008.08.006
- Hu Y, Burucs Z, von Tucher S, Schmidhalter U. 2007. Short-term effects of drought and salinity on mineral nutrient distribution along growing leaves of maize seedlings. Environ Exp Bot. 60:268–275. doi: 10.1016/j.envexpbot.2006.11.003
- Hurley MB, Rowart, JS. 1999. Resistance to root growth and changes in the concentrations of ABA within the root and xylem sap during root-restriction stress. J Exp Bot. 50:799–804. doi: 10.1093/jxb/50.335.799
- Ismail MR, Davies W. 1998. Root restriction affects leaf growth and stomatal response: the role of xylem sap ABA. Sci Hortic-Amsterdam. 74:257–268. doi: 10.1016/S0304-4238(98)00090-9
- Ismail A, Hall A, Bray E. 1994. Drought and pot size effects on transpiration efficiency and carbon isotope discrimination of cowpea accessions and hybrids. Funct Plant Biol. 21:23–35.
- Laus MN, Soccio M, Trono D, Liberatore MT, Pastore D. 2011. Activation of the plant mitochondrial potassium channel by free fatty acids and acyl-CoA esters: a possible defence mechanism in the response to hyperosmotic stress. J Exp Bot. 62:141–154. doi: 10.1093/jxb/erq256
- Lobell DB, Roberts MJ, Schlenker W, Braun N, Little BB, Rejesus RM, Hammer GL. 2014. Greater sensitivity to drought accompanies maize yield increase in the US midwest. Science. 344:516–519. doi: 10.1126/science.1251423
- Marschner H. 2012. Marschner's mineral nutrition of higher plants. London: Academic press.
- Nar H, Saglam A, Terzi R, Varkonyi Z, Kadioglu A. 2009. Leaf rolling and photosystem II efficiency in Ctenanthe setosa exposed to drought stress. Photosynthetica. 47:429–436. doi: 10.1007/s11099-009-0066-8
- Naveed M, Mitter B, Reichenauer TG, Wieczorek K, Sessitsch A. 2014. Increased drought stress resilience of maize through endophytic colonization by Burkholderia phytofirmans PsJN and Enterobacter sp. FD17. Environ Exp Bot. 97:30–39. doi: 10.1016/j.envexpbot.2013.09.014
- Nayyar H, Gupta D. 2006. Differential sensitivity of C3 and C4 plants to water deficit stress: association with oxidative stress and antioxidants. Environ Exp Bot. 58:106–113. doi: 10.1016/j.envexpbot.2005.06.021
- Praba ML, Cairns J, Babu R, Lafitte H. 2009. Identification of physiological traits underlying cultivar differences in drought tolerance in rice and wheat. J Agron Crop Sci. 195:30–46. doi: 10.1111/j.1439-037X.2008.00341.x
- Sabaté S, Gracia CA, Sánchez A. 2002. Likely effects of climate change on growth of Quercus ilex, Pinus halepensis, Pinus pinaster, Pinus sylvestris and Fagus sylvatica forests in the Mediterranean region. Forest Ecol Manag. 162:23–37. doi: 10.1016/S0378-1127(02)00048-8
- Sangakkara U, Frehner M, Nösberger J. 2000. Effect of soil moisture and potassium fertilizer on shoot water potential, photosynthesis and partitioning of carbon in mungbean and cowpea. J of Agron Crop Sci. 185:201–207. doi: 10.1046/j.1439-037x.2000.00422.x
- Shangguan Z, Shao M, Dyckmans J. 2000. Effects of nitrogen nutrition and water deficit on net photosynthetic rate and chlorophyll fluorescence in winter wheat. J Plant Physiol. 156:46–51. doi: 10.1016/S0176-1617(00)80271-0
- Siddique M, Hamid A, Islam M. 2000. Drought stress effects on water relations of wheat. Bot Bull Acad Sinic. 41:35–39.
- Sinclair T, Ludlow M. 1985. Who taught plants thermodynamics? The unfulfilled potential of plant water potential. Funct Plant Biol. 12:213–217.
- Terzi R, Saglam A, Kutlu N, Nar H, Kadioglu A. 2010. Impact of soil drought stress on photochemical efficiency of photosystem II and antioxidant enzyme activities of Phaseolus vulgaris cultivars. Turk J Bot. 34:1–10.
- Wahid A, Rasul E, Rao R, Iqbal R. 2005. Photosynthesis in leaf, stem, flower and fruit. Handbook of Photosynthesis. 479–497.
- Wu Y, Huang M, Gallichand J. 2011a. Transpirational response to water availability for winter wheat as affected by soil textures. Agr Water Manage. 98:569–576. doi: 10.1016/j.agwat.2010.10.015
- Wu Y, Huang M, Warrington DN. 2011b. Responses of different physiological indices for maize (Zea mays) to soil water availability. Pedosphere. 21:639–649. doi: 10.1016/S1002-0160(11)60166-5
- Wu Y, Huang M, Warrington DN. 2011c. Growth and transpiration of maize and winter wheat in response to water deficits in pots and plots. Environ Exp Bot. 71:65–71. doi: 10.1016/j.envexpbot.2010.10.015
- Wu Y, Huang M, Warrington DN. 2015. Black locust transpiration responses to soil water availability as affected by meteorological factors and soil texture. Pedosphere. 25:57–71. doi: 10.1016/S1002-0160(14)60076-X
- Zhao TJ, Sun S, Liu Y, Liu JM, Liu Q, Yan YB, Zhou HM. 2006. Regulating the drought-responsive element (DRE)-mediated signaling pathway by synergic functions of trans-active and trans-inactive DRE binding factors in Brassica napus. J Biol Chem. 281:10752–10759. doi: 10.1074/jbc.M510535200
- Zhong Y, Yan W, Chen J, Shangguan Z. 2014. Net ammonium and nitrate fluxes in wheat roots under different environmental conditions as assessed by scanning ion-selective electrode technique. Sci Rep. 4:7223. doi:10.1038/srep07223.