ABSTRACT
The association between functional traits and nitrogen use efficiency (NUE) was investigated to assist the breeding of nitrogen (N) use-efficient bread wheat (Triticum aestivum ssp. aestivum) varieties. This study combined results from a climate chamber experiment involving 41 spring wheat varieties and a field experiment involving six winter and six spring wheat varieties grown with and without the application of mineral N fertiliser. The climate chamber experiment was analysed by partial least squares (PLS) regression, with several predictors and NUE as response, to identify traits related to NUE. Specific hypotheses were then tested in the field experiment. The PLS indicated six traits of particular importance for overall NUE: leaf chlorophyll (SPAD value) of the top leaf at stem elongation, grains ear−1, ears pot−1, straw biomass pot−1, days between emergence and anthesis, and days between emergence and completed senescence. In the field experiment, the SPAD value of flag leaves of winter wheat around anthesis was positively correlated with NUE and total grain N, at both N levels. Fast development was positively correlated with high NUE and N uptake efficiency in spring wheat. Early senescence of the flag leaf was positively correlated with grain N concentration and negatively correlated with grain-specific N efficiency in winter wheat at low N fertilisation levels. The results indicate that high SPAD value of the top leaf might be a candidate trait that could be used in wheat breeding for improved NUE, while genetic variation in senescence could possibly be used to tailor varieties for different end-use quality when grown at low N. More studies are needed to validate these findings in other environments and for other genotypes.
Introduction
Wheat is one of the most common arable crops in the world, grown on a larger area than either maize or rice (FAOSTAT Citation2015). It not only contributes carbohydrates to human and livestock diets, but is also estimated to contribute as much protein annually (60 million tonnes) as the global soybean crop (Shewry Citation2009). Nitrogen (N) fertilisation is used to enhance wheat yields and quality, but this practice has its drawbacks, for example, increased emissions of the greenhouse gas nitrous oxide (N2O) from agricultural soils (Bouwman et al. Citation2002) and eutrophication caused by N leaching from fields (Vitousek et al. Citation1997). Much of the N available in the soil is not recovered in the wheat crop or straw, for example, around 35% in the UK (Sylvester-Bradley & Kindred Citation2009). Improving the ability of wheat varieties to take up and utilise N is, therefore, desirable.
Finding and targeting traits associated with higher N use efficiency (NUE) could facilitate breeding of more efficient varieties. Since plants are complex and interact with their environment, it is difficult to predict which combinations of traits are possible and most beneficial. While modelling growth is possible, restrictions regarding certain combinations of traits may not be known in advance. Studying several traits at the same time, in existing varieties, could therefore provide additional knowledge on traits beneficial for N use, while also indirectly considering possible trade-offs between traits.
NUE can be calculated in different ways (see e.g. Fageria et al. Citation2008). For identification of traits important for N use, it is practical to use a method for which the connection between a trait and a NUE component has a plausible functional and mechanistic explanation. The NUE concept presented by Weih et al. (Citation2011) was used in this study (); a corresponding calculation tool is available in Weih (Citation2014). In this NUE concept, the components reflect different periods in plant development, allowing comprehensive links to be made between traits and NUE at different important stages of the crop's life. A discussion of different methodologies for assessing NUE and also quantitative comparisons of NUE measures calculated by different methodologies is found elsewhere (Weih et al. Citation2011; Asplund et al. Citation2014; Pourazari et al. Citation2015).
Table 1. Definitions of NUE components according to Weih et al. (Citation2011, Citation2014).
Vigorous growth early in the season is a trait that could potentially be beneficial for N uptake and, therefore, have a positive impact on N uptake efficiency (UN). In pot experiments performed by Liao et al. (Citation2004, Citation2006), a wheat line with early vigorous shoot growth was also shown to have vigorous root growth and high N (HN) uptake. The same wheat line produced higher shoot and root biomass in unploughed soil than a conventional cultivar (Watt et al. Citation2005). Barley varieties with high seedling root weight (in hydroponics) have higher N uptake at low N (LN) fertilisation levels in the field than varieties with low seedling root weight (Bertholdsson & Kolodinska Brantestam Citation2009).
Another factor that could be important for NUE is the amount of chlorophyll in the leaf, which is correlated with leaf N and represents a functional link between plant N and carbohydrate production. The Minolta SPAD meter (SPAD-502, Konica Minolta Sensing Inc., Japan) is a tool for assessing leaf chlorophyll per unit leaf area in a fast, non-destructive manner. It is generally used for optimising N applications within a certain crop stand (e.g. Peltonen et al. Citation1995), since LN availability can decrease the SPAD value and can also be associated with lower yield. However, the SPAD value is not only affected by the environment, but also differs between varieties. Le Bail et al. (Citation2005) found that different equations were needed to relate SPAD values to the harvested grain N depending on variety. Leaf chlorophyll is thus affected by genetics, not only by environment, indicating the potential for using SPAD values when breeding for higher NUE.
The end of the wheat crop life, encompassing, for example, leaf senescence, is another stage that is considered important for NUE. Delayed senescence of the leaves is believed to be positive for grain biomass production (Gaju et al. Citation2011). On the other hand, connections have been seen between accelerated senescence and efficient retranslocation of N to the grain (Uauy et al. Citation2006). Late maturation can be risky when the growing season is short, as in Sweden. For example, a rare allele of a gene that causes earlier maturation has been found in spring wheat varieties from Sweden and other countries in Fennoscandia (Hagenblad et al. Citation2012; Asplund et al. Citation2013), possibly because early maturation is beneficial in this climate. Therefore, it is particularly interesting to study senescence in this area.
Winter wheat generally achieves higher yield than spring wheat when grown in cool-temperate climate, but the spring wheat often has higher grain N concentration (CN,g). This pattern was seen also when a facultative wheat was grown as winter and spring type in a temperate climate (Neugschwandtner et al. Citation2015). The different management of the two types of wheat makes them somewhat difficult to compare. However, the mean yield per hectare for winter wheat in Sweden was 6170 kg ha−1 between 2008 and 2012, while that of spring wheat was 4190 kg ha−1 (Swedish Board of Agriculture & Statistics Sweden Citation2014). Moreover, the grain protein concentration in wheat varieties tested in the Swedish official variety trials is generally higher in spring wheat than in winter wheat (Larsson et al. Citation2013). Higher yield of winter wheat should be related to, for example, its longer growing season and possibly deeper rooting depth, increasing the availability of nutrients and water (Thorup-Kristensen et al. Citation2009). Due to the likely connection between rooting depth, N supply and grain yield, a comparison of NUE in spring wheat and winter wheat could increase knowledge about the associations between NUE and functional traits.
In a study by Asplund et al. (Citation2014), wheat varieties ranked similarly in the field and in the greenhouse in terms of both NUE and UN calculated according to the method of Weih et al. (Citation2011). Different types of studies have different strengths and limitations, but the link shown by Asplund et al. (Citation2014) demonstrates that combining results from different experimental approaches can be fruitful in crop NUE research.
The aim of this study was to identify traits related to NUE components. Data from two different experiments were used to link functional traits to NUE components. Thus, data from a climate chamber experiment with 41 varieties (previously used to study a specific gene, Asplund et al. Citation2013) were used to identify relationships between NUE and other traits. The most important trait relationships identified in the climate chamber, plus some additional relationships between the NUE components and early vigour, were then tested against another partly published (Asplund et al. Citation2014) data set from a field experiment with two levels of N fertilisation and six varieties each of winter wheat and spring wheat. More specifically, we expected links between some NUE components and early vigour, SPAD value and the dynamics of leaf senescence. Therefore, three specific hypotheses were tested in the field experiment: (1) There is a positive relationship between early vigour (high early aboveground biomass) and UN; (2) there is a positive relationship between leaf chlorophyll (SPAD value) and grain biomass and (3) rapid leaf senescence is accompanied by higher CN,g and lower grain-specific N efficiency (EN,g).
Materials and methods
Partial least squares (PLS) regression was performed on data from the climate chamber experiment. Hypotheses generated from this analysis were tested on data from the field experiment.
Plant material
Climate chamber experiment
Forty-one varieties of spring wheat (Triticum aestivum ssp. aestivum) were used (), of which 38 were donated by NordGen and three by a local farmer. The varieties included three landraces with uncertain background cultivated for 10 years in Uppsala, Sweden, six landraces of Swedish origin preserved in NordGen, 29 Swedish varieties released during the twentieth century and three varieties from other countries which were used in early Swedish breeding.
Table 2. Varieties of wheat used in the climate chamber experiment.
Field experiment
Six spring wheat and six winter wheat varieties were grown. Spring wheat varieties used were a landrace originating in Dalecarlia (Dala), ‘Diskett’, ‘Granary’, ‘Quarna’, ‘Stilett’ and ‘Vinjett’, and winter wheat varieties were a landrace from Germany, ‘Kranich’, ‘Harnesk’, ‘Kosack’, ‘Olivin’ and ‘Loyal’. Two of the varieties were landraces grown by a local farmer for around 10 generations at the experimental site. The varieties represented the span of variation in grain yield, grain protein content, grain size, plant height and maturation time recorded in the 2008 Swedish variety trials (Larsson et al. Citation2008) and recorded by the farmer in the case of the landraces. The intention when selecting varieties was to ensure that the varieties were dissimilar, but still well-adapted to Swedish growth conditions.
Experimental design and management
Climate chamber experiment
The experiment was laid out in a complete randomised block design with four replicates and with pot as the experimental unit. The conditions in the climate chamber was 16 h light, a 9/18°C night/day temperature regime, photosynthetically active radiation (PAR) about 230 µmol m−2 s−1 at the level of the top edge of the pots and 60% relative humidity. The pots were 13 cm × 13 cm × 13 cm in size, and the potting mix was a fertilised blend of peat, perlite, silica clay and gravel. The potting mix initially contained 260 mg N per pot. The planting depth was 2.5 cm and the five plants sown were thinned down to two plants per pot.
The pots were initially placed on nets but were moved to trays (nine pots per tray) after the first harvest to allow nutrient solution to be soaked up when added later on. Nets above the trolleys provided support when the plants grew taller. The plants were moved to lower trolleys on day 69 to allow further vertical growth of the plants. The plants were irrigated on the trays, initially only with deionised water, but from day 27 to 122 the plants were also supplied twice a week with 0.2 L nutrient solution per pot. The nutrient solutions were prepared from a balanced, complete fertiliser, ‘Blomstra’ (Cederroth, Upplands Väsby, Sweden), with NH4+ and NO3− nitrogen in the proportion 2:3 and containing (mg L−1): 102 N, 20 P, 86 K, 8 S, 6 Ca, 8 Mg, 0.34 Fe, 0.4 Mn, 0.2 B, 0.06 Zn, 0.03 Cu and 0.0008 Mo. The dose was halved to 0.1 L nutrient solution applied twice a week from day 123. The plants were watered with deionised water as necessary to keep the soil moist. The beginning of anthesis of the main stem in each pot (growth stage (GS) 61; Tottman Citation1987) was recorded as the day when one of the two plants had reached this stage. More details can be found in Asplund et al. (Citation2013).
Field experiment
The field experiment was designed as a complete block split-split plot with four replications. The main plot factor was type of wheat (winter or spring wheat), with the fertilisation treatments LN and HN randomised on sub-plots within the wheat type. Varieties were randomised in sub-sub plots within the sub-plots.
The field experiment was conducted in the period October 2009–August 2010 at a site near Uppsala, Sweden (59°50′N, 17°47′E). A summary of climate data is presented in . The previous crop was pea and the experimental plots (sub-sub plots) measured 2 m × 16 m. Destructive sampling was limited to the outermost 3 m at each end of the sub-sub plots, while 10 m in the centre was kept intact for grain yield determination. Sowing took place on 2 October 2009 (winter wheat) and 29 April 2010 (spring wheat), with 400 and 550 viable seeds m−2, respectively, which are the standard seed rates for wheat in variety trials in Sweden. On 30 April 2010, the HN treatment received 81 kg N ha−1 as ammonium nitrate mixed with calcium carbonate and sulphur (0.27 g g−1 N) in both spring and winter wheat. The LN treatment did not receive any fertiliser. There were sufficient amounts of P and K in the soil. The herbicides Ariane S and Hormotex were applied once to control weeds. There was no need for any pest or disease control.
Table 3. Monthly climate data for the duration of the field experiment, measured at Ultuna meteorological station about 8 km from the experimental site.
Soil samples were taken from main plots with winter wheat to determine soil mineral N (SMN) on 6–7 November 2009 when no further growth was expected and on 14–15 April 2010 in each of the eight main plots. The reasons for determining SMN were to get an indication of the nitrogen levels in which the seedlings were growing both in the autumn and in the spring and to determine N fertiliser doses. The top 30 cm of the soil was silty clay (British Standards Institution) with 0.056 g g−1 organic matter content. The soil pH (H2O) was 6.4, 6.9 and 7.1 (0–30, 30–60 and 60–90 cm). The mean total amount of ammonium and nitrate N in the soil profile (0–90 cm) was 33 kg ha−1 in the autumn. In the spring, it was 95 kg ha−1 in the spring wheat plots and 80 kg ha−1 in the winter wheat plots before the addition of fertiliser. Thus, the N level in the soil was higher at the sampling occasion in the spring than in the autumn, which indicates net mineralisation during winter. Further details can be found in Asplund et al. (Citation2014).
Measurements
Climate chamber experiment
The SPAD value (SPAD-502, Konica Minolta Sensing Inc., Japan) of the uppermost leaves was recorded on day 27, when the plants were in the stem elongation stage (GS 32–37). The SPAD values were estimated by taking a mean of three measurements from along the middle of the leaves. Leaf senescence was measured by monitoring SPAD values at regular time intervals in the same way. The two main shoots in each pot were individually labelled and flag leaves were measured initially three, and later two, times a week from a few days before anthesis until the leaves were completely yellow. Leaf senescence was thereafter modelled as described in Asplund et al. (Citation2013). The modelled curve consisted of two straight lines, a left horizontal plateau connected with a slope to the right, according to Equation (1)(1)
where the response (g) is the SPAD value and the predictor (x) is the number of days after anthesis. The parameter β0 corresponds to the intercept and level of the left segment/plateau, β2 corresponds to the slope of the right segment which is the senescence rate and τ is the breakpoint between the two segments. Senescence start (at the breakpoint between the lines), rate (slope of the right line) and length (in days from the breakpoint to complete senescence) can be obtained from this model.
The experiment was harvested block-wise on days 138 and 139, when all plants were considered completely mature. In each pot, the number of ears was counted and the height was calculated as a mean of the two main stems, measured from the soil surface to the tip of the ears, excluding awns. The ears were threshed by hand, and the grain and straw were dried for 48 hours at 60°C. Grains were counted by hand and weight per grain was calculated by dividing the dry weight by the number of grains. Grain samples were milled using a knife mill (Grindomix GM 200, Retsch GmbH) and analysed for N concentration using dry combustion (CNS2000, LECO Corporation, Saint Joseph, MI, USA). For occasional ears that were malformed, very late maturing, or where the stem had broken, the grains were not analysed for nutrient content. These unanalysed grains were weighed and the total amount of N was calculated by assuming the same concentration in damaged and undamaged grains within the same pot. The yield of N, that is, the weight, was calculated by multiplying the dry weight of biomass by the concentration of N, adjusted for water content. Harvest Index (HI) was calculated by dividing the grain biomass by the total aboveground biomass, and N Harvest Index was calculated by dividing the grain N yield by the yield of N in the aboveground biomass.
Field experiment
Early vigour
Winter wheat was sampled on 16–17 November 2009 at around GS 11. One sub-sub plot per variety was randomly chosen from each block. Five seedlings were sampled from one corner of the plots; these five plants were considered well representing the sample plots, which were rather homogeneous. The biomass was dried for 24 h at 80°C, followed by 48 hours at 60°C before weighing. All subsequently mentioned biomass samples were dried at 60°C to reach constant weight, which took between 24 and 72 h depending on the type of sample. The sub-sub plots with winter wheat that were not sampled in the autumn were sampled on 27 April, during tillering. Spring wheat in the LN treatment was sampled on 25 May at around GS 13. The number of plants m−2 was assessed on 27 April 2010 for winter wheat and 28 May and 1 June 2010 for spring wheat by counting plants on 4 row-metres per plot.
Major growth period
The mean plant N content during the major growth period is an essential element in the NUE concept by Weih et al. (Citation2011). To calculate the mean N during the major growth period, samples were taken before and after the major growth period. The first sampling took place on 24–31 May, at around GS 13 in spring wheat and the beginning of stem elongation (around GS 31) in winter wheat. The sampling at the end of the major growth period took place on 28 June–1 July for winter wheat and 5–8 July for spring wheat, and the plants were in GS 55–69 at sampling. Five plants per plot were picked randomly from the areas in both ends of the plots (totalling ten plants per plot). The dried plant biomass was ground using a knifemill and thereafter a ball mill. The ball mill grinding and the N analysis were carried out by Waikato Stable Isotope Unit (University of Waikato, Hamilton, New Zealand) using a Dumas elemental analyser (Europa Scientific ANCA-SL) interfaced to an isotope mass spectrometer (Europa Scientific 20–20 Stable Isotope Analyser) (Europa Scientific Ltd, Crewe, UK).
Other measurements
Developmental stage (Tottman Citation1987) was recorded in all sub-sub plots on 24 June. SPAD measurements were made in all sub-sub plots on 2 July (winter varieties) and 9 July (spring varieties), that is, just after the sampling at the end of the major growth period. Measurements of 30 haphazardly chosen flag leaves were taken along the whole plot. The measurement points were distributed evenly, with 15 values on each side of the plot. For each plot, the SPAD value thus represented an average of 30 flag leaves. One measurement was taken in the middle of each leaf. All the sub-sub plots of each type were measured within one day, between 9.00 and 15.00 hours. These days were sunny, with scattered clouds. Visual grading of flag leaf colour was carried out on 22 July (autumn varieties) and 28 July (spring varieties). The average percentage yellow flag leaf area was assessed according to the following scale: 1 = 0% yellow flag leaf area, 2 = 0.1–1.0%, 3 = 1.0–5.0%, 4 = 5–10%, 5 = 10–25%, 6 = 25–50%, 7 = 50–75%, 8 = 75–100%, 9 = dead. Sampling to determine aboveground biomass (B) was carried out on 11 and 20 August for winter and spring wheat, respectively. A total area of 0.5 m2 was sampled from each plot (two 0.5×0.5 m squares were sampled in each plot, one on each end). Thereafter, the samples were dried and weighed. Grain yield was determined from the inner 20 m−2 in each plot at the harvest on 28 August 2010 and the weight was adjusted to 0% H2O. Nitrogen concentration was determined using the near infrared transmittance method (Infratec™ 1241 Grain Analyser, Foss, Denmark) and a conversion factor of 5.7 from protein concentration.
NUE calculations
NUE and its components were calculated in the climate chamber and field study according to the method of Weih et al. (Citation2011) (). In the climate chamber experiment, the N in seed grain (Ns) was estimated by weighing the sown grains and assuming a grain N concentration of 2%. Exact seed grain N concentrations could not be determined because only very limited grain quantities were available from the gene bank from which we obtained the material. In the field experiment, not all grains germinated. The grains may have differed in N content, but it was not possible in this experiment to determine the N content of the particular grains that had germinated in comparison to the ones that did not germinate. Thus, Ns was estimated by assuming that germinated grains had the same grain N concentrations as the whole lot of sown grains of the same variety, that is, for each plot the grain N concentration of each variety was multiplied by the single grain weight and the number of germinated seedlings based on seedling count. Harvest was performed at fixed points in time (not at a certain GS). For the climate chamber experiment, NUE and its components were calculated per pot and for the field experiment per m2. For the field experiment, N translocation to grain was calculated as the ratio between total N amount in grain at harvest and N in the plant at the end of the major growth period.
Statistical analysis
The climate chamber and field experiments were analysed separately, but the results from the climate chamber were also tested using data from the field experiment. Some traits were not measured in exactly the same way in the two experiments and in those cases measurements assumed to represent the same trait were used. The development around anthesis was measured as the day of anthesis in the climate chamber experiment, but in the field all varieties were assessed at one time point. Senescence was measured at regular time intervals with a SPAD meter in the climate chamber, while in the field it was assessed visually at one time point each for spring and winter wheat. The SPAD value of the uppermost leaf at stem elongation, but not at anthesis, was found to be important in the climate chamber experiment. However, in the field experiment only the SPAD value at anthesis was available.
For the climate chamber analysis, the software SAS® (SAS Institute Inc. Citation2011) procedure PLS was used to perform a PLS analysis. This multivariate regression technique strives to explain both predictor and response variation and was used here to identify the traits (predictors) that best explained variety NUE (response). Mean values for each variety were calculated for all variables and used as input in the analysis, so that each observation in the data set analysed corresponded to one variety. There were no missing values in the analysis. The number of factors to extract was chosen using split-sample cross-validation and van der Voet's test. Predictors and response were centred and scaled to a mean of 0 and a standard deviation of 1. Variables (traits) with a small Variable Importance for Projection (under 0.8) and small absolute regression coefficients were considered not to be important for the response (NUE) and were dropped from the model (SAS 9.3 User's Guide).
The field experiment was analysed as a linear mixed model with the SAS procedure Mixed. Block, main plot and sub-plot were treated as random effects. Type (spring/winter), fertilisation, variety within type and their interaction were treated as fixed effects. NUE, UN and N′ were log10-transformed to meet the assumption of homogeneity of variances of the statistical analysis. Degrees of freedom were calculated according to Kenward and Roger (Citation1997). Contrasts were also calculated to distinguish differences between varieties within type, as well as the interaction between fertilisation and variety within type.
Variables that were identified as being important in the PLS analysis of the climate chamber data were further analysed in the field experiment when possible. Some additional traits not measured in the climate chamber experiment were also analysed. ANOVA was performed on the variables to identify significant differences between varieties and treatments, and then correlations were performed (on means for each variety and treatment) to test for associations between traits and NUE components. The p-values for the correlations were calculated only in the cases where the observations could be assumed to be independent, that is, not for varieties grouped into different types and fertilisation treatments. Percentage yellow flag leaf area and GS, even though measured on ordinal scales, were analysed as the other variables with Proc Mixed in SAS. It was not possible to fit generalised linear mixed models. However, the residuals of the chosen model looked good on visual inspection. Spearman correlation coefficients were calculated for the association between yellow leaf area and NUE components, using median values for each variety and treatment combination. Spearman correlations were also used to test for correlations between developmental stage and NUE components.
All statistics were computed with the software SAS version 9.3 (SAS Institute Inc. Citation2011). The figures were produced with the software R version 2.15.2 (R Development Core Team Citation2012) and Adobe Illustrator CS6 version 16.0.0 (Adobe Systems Incorporated © Citation1987–Citation2012).
Results
Identification of important traits (climate chamber study)
The PLS analysis for the 41 varieties grown in the climate chamber identified six traits of particular importance for NUE: number of grains per ear, number of ears per pot, straw biomass per pot, SPAD value of the top leaf at stem elongation, days between emergence and anthesis, and days between emergence and completed senescence. Eight variables were dropped from the analysis because of low importance in the PLS analysis: single kernel weight, plant height, days between anthesis and start of senescence, rate of senescence, SPAD at anthesis (either modelled or measured), HI, days between start and end of senescence and year of release of the variety. The analysis was repeated without these variables and one factor was extracted based on van der Voet's test. This factor explained 38% of the variation in the model effects (traits) and 74% of that in the dependent variable (NUE). Model statistics for the extracted factor are presented in . The six important trait variables were plotted against NUE to visualise the relationships ().
Figure 1. Plot of traits found to be important for nitrogen use efficiency (NUE) in the climate chamber experiment with 41 varieties of spring wheat, based on partial least squares (PLS) analysis. Circles represent the mean of four replicates. These important traits are (a) SPAD of the uppermost leaf at stem elongation (NUE = −307.0 + 12.9 × SPAD, R2 = .40, p < .0001); (b) number of grains per ear (NUE = 112.1 + 4.8 × grains, R2 = .16, p = .01); (c) number of ears per pot (NUE = 2.4 + 28.7 × ears, R2 = .55, p < .0001); (d) straw biomass (Bst) (NUE = 86.2 + 7.6 × Bst, R2 = .43, p < .0001); (e) days from emergence to anthesis (NUE = 705.0–5.7 × days, R2 = .09, p = .052) and (f) days from emergence to senescence of the flag leaf (NUE = 635.5–2.9 × days, R2 = .07, p = .10).
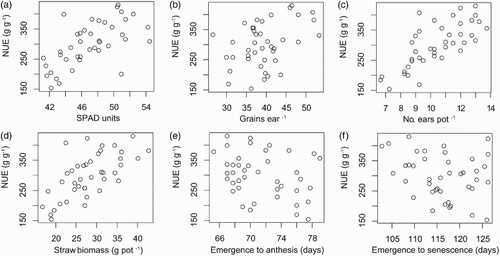
Table 4. Statistics derived from a partial least squares (PLS) analysis with NUE as the dependent variable, with one extracted factor.
Test of important traits in the field experiment
The traits identified as important for NUE in the climate chamber, as well as NUE components and early vigour, were studied in the field experiment.
Wheat type and variety effects
NUE and N uptake efficiency (UN) were higher in winter wheat than in spring wheat, while grain N concentration (CN,g) was lower ( and ). The grain-specific N efficiency (EN,g) was similar between the wheat types. Varieties within the wheat types differed in overall NUE and all NUE components. Grain biomass (Bg) was higher in winter wheat, and there were significant variety effects in both spring and winter wheat. Mean plant N content during the major growth period (N′) was higher in winter wheat, but there was no significant variety effect. Grain N content (Ng) was similar between wheat types, but varied between varieties within both wheat types.
Table 5. Results from ANOVA of several variables collected in a field experiment, showing F and p-values for the fixed effects.
During autumn and in early spring, winter wheat seedlings differed between varieties in terms of aboveground biomass (p < .0001 and p = .005 before and after winter, respectively; ). The seedlings of the different spring wheat varieties did not differ in terms of aboveground biomass. Both winter and spring varieties differed in developmental stage on 24 June. The SPAD values of the flag leaf at anthesis were higher in winter than in spring wheat and there were significant variety effects in both groups. Straw biomass was similar in the different wheat types and varieties. The varieties differed in yellow flag leaf area. Nitrogen translocation to grain, here calculated as the ratio between total N in grain at harvest and N in the plant at the end of the major growth period, was similar between the wheat types, but differed between the spring wheat varieties. The variety ‘Granary’ stood out, with very high translocation (ratio of 1.15, SE 0.08), indicating considerable N uptake after the major growth period and possibly more efficient N translocation than the other varieties. The mean N translocation of all observations was 0.75.
Nitrogen fertilisation effects
HN fertilisation resulted in higher NUE, UN and CN,g, and lower EN,g. Increased NUE with increased N fertilisation is expected using the equation by Weih et al. (Citation2011) since fertilisation increases the N content of the harvested grain (the numerator in the equation) while not affecting the seed grain N content (denominator). The variables N′, Ng and Bg also increased with the use of N fertiliser. The higher number of grains per m2 in the HN treatment was the result of an increase in both number of grains per ear and number of ears per m2. The HN treatment also resulted in higher SPAD values and straw biomass.
The N fertilisation response of leaf senescence (assessed in terms of yellow flag leaf area) was affected by wheat type (e.g. significant type × fertilisation effect, ), here reflecting that senescence in winter wheat grown at LN had progressed further than in all other cases. Many variables showed significant variety × fertilisation interaction effects within the winter wheat type ().
Associations between traits and NUE in the field experiment
In winter wheat, NUE and early aboveground biomass measured in winter and spring were inversely correlated at LN, but not at HN ().
Table 6. Pearson correlations between NUE or UN and aboveground biomass of seedlings of six winter wheat varieties in a field experiment.
Bg was not significantly correlated with SPAD either across or within treatments (, ). In contrast, NUE of winter wheat was positively correlated with SPAD at anthesis when grown in the LN treatment, but not when assessed across both N fertilisation treatments (). The Ng of winter wheat was positively correlated with SPAD at anthesis at LN and HN () and also when assessed across both N fertilisation treatments ((b)). Both N′ vs. Ng and N′ vs. SPAD showed positive, close to significant, associations across treatments (, (a) and 3(c)). The correlation between SPAD at stem elongation and Ng was significant in the climate chamber experiment across the 41 varieties (R2 = .55, p < .0001).
Figure 2. Plot of SPAD against grain biomass (Bg) in a field experiment with spring and winter wheat varieties and two N treatments. SPAD refers to measurements taken on 2 July (winter varieties) and 9 July (spring varieties), that is, just after the major growth period, on 30 flag leaves per plot. Circles represent spring wheat and triangles winter wheat, open symbols represent the LN treatment and filled symbols the HN treatment. The equation for the relationship between the variables is Bg = −340 + 19xSPAD, and R2 = .65.
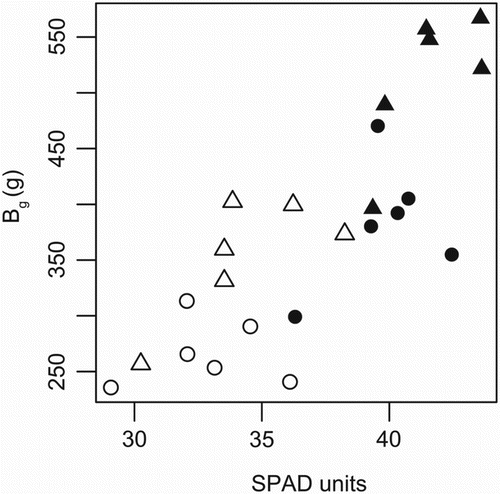
Figure 3. (a) Plot of total grain N (Ng) against mean N during the major growth period (N′) (Ng = 3.44 + 0.51×N′, R2 = .64), (b) Ng against SPAD (Ng = −6.99 + 0.36×SPAD, R2 = .86) and (c) N′ against SPAD (N′ = −11.03 + 0.46×SPAD, R2 = .55), in a field experiment with spring and winter wheat varieties and two N treatments.
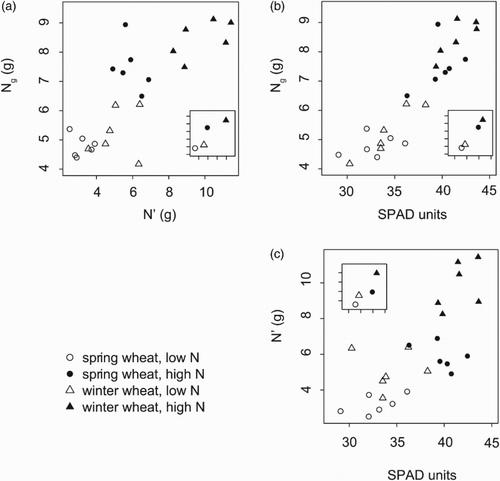
Table 7. Correlations (Pearson and Spearman) between NUE components and functional traits collected in a field experiment with spring and winter wheat at two fertiliser levels.
Straw biomass was uncorrelated with NUE within wheat type and fertilisation combinations, but there was a positive correlation across groups ().
Days to anthesis was identified as an important trait for NUE in the PLS carried out with the data of the climate chamber study (). Since anthesis occurred close to the critical sampling time for UN (i.e. end of the major growth period), the relationship to both NUE and UN was tested in the field experiment. In spring wheat, the UN was positively correlated with the developmental stage on 24 June within both N treatments (, (a) and 4(b)), and NUE was positively correlated with the developmental stage only at LN ().
Figure 4. Relationship between (a) uptake efficiency (UN) and growth stage (GS) on 24 June in spring wheat, LN (UN = −43.19 + 1.30×GS, Spearman correlation coefficient = 0.83, p = .04), (b) UN and GS in spring wheat, HN (UN = −44.59 + 1.46×GS, Spearman correlation coefficient = 0.96, p = .003), (c) grain N concentration (CN,g) and yellow flag leaf area (YFLA) in winter wheat, LN (CN,g = 0.00699 + 0.00117×YFLA, Spearman correlation coefficient = 0.83, p = .04) and (d) grain-specific N efficiency (EN,g) and YFLA in winter wheat, LN (EN,g = 202.4–19.2×YFLA, Spearman correlation coefficient = −0.93, p = .008).
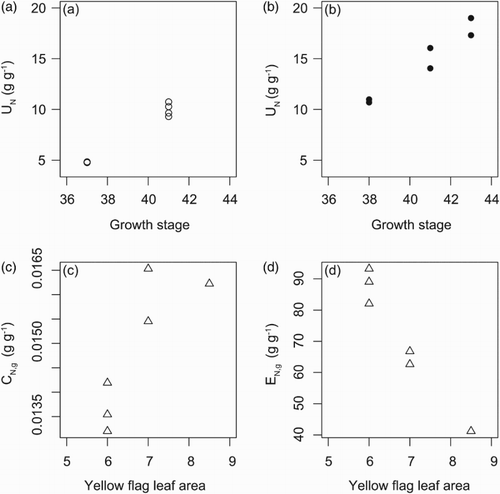
Significant correlations between yellow flag leaf area, indicating senescence, and NUE components were found in winter wheat at LN, including a positive correlation with CN,g (, (c)) and a negative correlation with EN,g (, (d)). Nitrogen translocation to grain was negatively correlated with leaf senescence only at LN in spring wheat ().
Discussion
This paper integrates the data from two independent studies carried out under different experimental conditions, which allows for more robust conclusions, particularly regarding the hypothesised links between some NUE components, early vigour, SPAD value and the dynamics of leaf senescence, than would be expected considering that field data from only one growing season were available. The findings are relevant for wheat, especially when grown under northern-temperate climate, with implications for wheat breeding research.
Association between early vigour and uptake efficiency
The hypothesis that high early biomass, as a consequence of early vigour, is positively connected with UN was not supported in this study (). There was even a negative relationship between early biomass and NUE at LN in winter wheat, mainly caused by the landrace having a much lower NUE in combination with higher early biomass (). Early vigour is believed to be beneficial for both N uptake and yield (Watt et al. Citation2005; Bertholdsson & Kolodinska Brantestam Citation2009), and while our results could not confirm a relationship between early vigour and UN or NUE, the relationship between these variables deserves further research attention.
In the field experiment, we also investigated whether the specific root length of the seedlings was correlated with UN, but we found no significant relationship (data not presented).
Associations between SPAD value and grain biomass
Leaf chlorophyll contents by means of SPAD values recorded at anthesis have previously been found to be highly indicative for biomass production in cereals (e.g. Figueiredo et al. Citation2014). In our field experiment, the relationship between SPAD value and grain biomass (Bg) was positive, but not significant (, ). Semenov et al. (Citation2007) predicted in a modelling study that a higher specific leaf N content (SLN, here assessed by SPAD) in wheat would result in lower NUE sensu yield per unit mineral N supply, rather than higher yield as in our study. However, that model contained assumptions which do not necessarily hold for our study. We found no significant relationship between SPAD and the yield per mean N during the major growth period (EN,g) (), probably because the different varieties allocate different proportions of their total biomass to leaves and grain.
Specific leaf N was not measured in the present study, but a relationship between SPAD value and SLN is commonly reported, especially when compared at the same developmental stage (Chapman & Barreto Citation1997). Plant NUE is affected not only by the N amount in the canopy, but also by its spatial distribution across the canopy. For example, higher flag leaf SPAD and most likely higher SLN in the present study could be connected to a more optimal distribution of N in the canopy, which in turn has been argued to improve the NUE of wheat (reviewed by Foulkes et al. Citation2009).
Associations between SPAD value, grain N content and mean N
A relationship was found between the SPAD value of the uppermost leaves and grain N content (Ng). This pattern was present in the data set of the 41 varieties tested in the climate chamber, in the field experiment as a general trend over all data and within N fertilisation treatments in winter wheat. While the SPAD value at stem elongation, but not at anthesis, was found to be important in the climate chamber (, (a)), the SPAD value at anthesis was correlated with NUE and Ng in the field (, (b)). That inconsistency could be related to extended access to N for the plants in the climate chamber experiment compared with the plants in the field experiment. SPAD values have previously been found to be well correlated to protein yield within varieties of winter wheat and spring barley (Le Bail et al. Citation2005; Spaner et al. Citation2005). In the present study, we found a relationship between SPAD value and Ng across different varieties.
One possible mechanism behind the relationship between SPAD and Ng could be that high SPAD values are indicative of a HN pool, which is then transferred to the grain. These relationships (between SPAD, Ng and N′) were positive, but not significant in the present study (, (a)–(c)). Other mechanisms, for example, related to senescence, probably affect the relationship between SPAD and Ng.
Association between leaf senescence, grain N concentration and grain-specific N efficiency
There was a positive relationship between senesced leaf area and CN,g ((c)), and a negative relationship between senesced leaf area and EN,g ((d)), as hypothesised, but only in winter wheat in the LN treatment (). This effect was not due solely to the effect on grain biomass, which was not significantly correlated with senescence. Gaju et al. (Citation2011) also found associations between onset of senescence and yield and uptake efficiency under low, but not high, N levels in winter wheat. Bogard et al. (Citation2011) studied a doubled haploid winter wheat population and found that the relationship between senescence and CN,g differed depending on the environment and should be used with caution in breeding for CN,g. The relationship may depend on post-anthesis N availability. The relationship between senescence and grain yield was more robust, with delayed senescence resulting in higher grain yield. The present results and those of, for example, Gaju et al. (Citation2011) and Bogard et al. (Citation2011) suggest a trade-off between high grain N concentration and high efficiency of using N for carbohydrate production during grain filling.
In spring wheat grown in the field, early development (higher developmental stage reached at a certain date) was positively associated with NUE and UN (, (a) and 4(b)). In the climate chamber experiment, the association between day of anthesis and NUE was negative, that is, early development was positive for NUE in that case too (, (e)). Gaju et al. (Citation2011) found a positive (i.e. the opposite) correlation between yield and anthesis date, but this difference in anthesis date was not connected to specific differences in responses of cultivars to N supply. Adjusting the length of different developmental phases has previously been suggested for yield improvement. An increase in the duration of the stem elongation could increase the number of grains through reduced floret abortion and increased partitioning of assimilates to the grains (Slafer et al. Citation2001; Reynolds et al. Citation2009). The link between early development and UN that was found in the present field experiment could be related to the specific environmental conditions, since it was rather dry after anthesis, but this was not the case in the climate chamber. Another possible explanation is that growth rate is connected to the GS (Kirby Citation1988) which may cause a correlation between UN and GS. The results highlight the connection between the developmental rate and NUE, and may be related to the processes determining yield described above.
In the spring wheat grown at LN in this study, higher N translocation to grain was associated with lower yellow flag leaf area, that is, greener leaves prior to harvest (). This pattern seems unexpected, because greener leaves prior to harvest would be expected to be connected with poorer N translocation to grain. It is possible that the greener leaves late in the season here indicate enhanced N uptake late in the season and subsequently enhanced N translocation to grain, but we have no data to corroborate this hypothesis. As a comparison, Kichey et al. (Citation2007) studied five winter wheat cultivars and found no relationship between translocation and other agronomic and physiological traits.
Differences between spring and winter wheat
NUE was higher in winter wheat, while harvested grain N (Ng) did not differ between spring and winter wheat (, ). Since sown N (Ns) was higher in spring wheat, winter wheat accumulated a similar amount of N in the harvested grain starting from a lower value of Ns, resulting in higher NUE. The UN was also higher in winter wheat (). However, the measurements of mean N (N′) in the beginning of the major growth period were taken at the same time for both wheat types, which could potentially have contributed to higher values of, for example, UN in winter wheat (cf. discussion by Asplund et al. Citation2014). Nevertheless, winter wheat still had higher UN if calculations were based on the sampling for studying early vigour, a time when winter wheat plants were smaller than the spring wheat plants were in the beginning of the major growth period. Therefore, the difference in sampling time at the beginning of the major growth period was not the cause of the higher UN of winter wheat in this experiment. The higher UN in winter wheat could be explained by a larger root system early in the season when spring wheat has just started growing, which contributes to obtaining N. For example, winter wheat roots have been observed to grow to depths twice as deep as spring wheat roots (Thorup-Kristensen et al. Citation2009).
The grain-specific N efficiency (EN,g) was similar in the spring and winter wheat ( and S1). EN,g can be viewed as a product of the allocation of biomass to the grain, and the total biomass produced from the N′, both of which only differed between varieties and not between types of wheat (data not presented). This indicates an absence of general differences between them in terms of photosynthesis per unit of N. The CN,g was higher in spring wheat ( and S1), as has been observed in many studies (e.g. Larsson et al. Citation2013). However, few previous studies have compared CN,g in spring and winter wheat grown in the same experimental design and at the same N fertilisation levels, as was done in the present study. When the effects of autumn- and spring-sowing were investigated in facultative wheats, higher yields, but lower grain N concentrations were found when sown in winter (Ozturk et al. Citation2006; Neugschwandtner et al. Citation2015). Since Ng did not differ between the types of wheat while the Bg was higher in winter wheat ( and ), our results are consistent with the commonly observed negative relationship between Bg and CN,g (Kibite & Evans Citation1984; Peltonen-Sainio et al. Citation2012).
Implications for breeding
Several functional traits were related to NUE in winter wheat in the LN treatment (). In addition, there were significant interactions between genotype and N fertilisation in many variables in the winter wheat, but we found no such interactions in the spring wheat (). These results suggest that in this study, N resources were depleted earlier in the winter wheat LN treatments. Furthermore, there was genotypic variation due to this limitation and the functional traits studied became a factor which separated the varieties in this situation. The importance of growing conditions was also observed by Asplund et al. (Citation2014) and explains the somewhat differing results between the climate chamber and field experiment in the present study. The genotype × fertilisation effects found in this study indicate the possibilities of using functional traits to achieve more efficient varieties in N-limited conditions.
We found a highly significant relationship between SPAD value around anthesis and Ng across both wheat types and treatments in the field experiment (cf. ) and between SPAD value at stem elongation and Ng across 41 spring wheat varieties grown in a climate chamber. If this relationship can be confirmed in other field situations and varieties, it would be a useful tool in breeding for varieties with higher Ng and thus higher NUE. It would also be interesting to investigate the relationship for SPAD values recorded at earlier GSs in the field.
Acknowledgements
We thank NordGen for providing seeds for the climate chamber experiment, Lennart Karlsson for contributing landraces seeds and SW Seed and Forsbecks AB for providing seeds for the field experiment. We also thank Marie Melander, Wera Kleve and Johan Gottfridsson for assisting with the growth experiments.
Disclosure statement
No potential conflict of interest was reported by the authors.
ORCID
Linnéa Asplund http://orcid.org/0000-0003-0479-5624
Göran Bergkvist http://orcid.org/0000-0001-9287-0510
Martin Weih http://orcid.org/0000-0003-3823-9183
References
- Adobe Systems Incorporated © 1987–2012. Adobe Illustrator CS6 version 16.0.0.
- Asplund L, Bergkvist G, Leino MW, Westerbergh A, Weih M. 2013. Swedish spring wheat varieties with the rare high grain protein allele of NAM-B1 differ in leaf senescence and grain mineral content. PLoS ONE. 8:e59704. doi:10.1371/journal.pone.0059704
- Asplund L, Bergkvist G, Weih M. 2014. Proof of concept: nitrogen use efficiency of contrasting spring wheat varieties grown in greenhouse and field. Plant Soil. 374:829–842. doi: 10.1007/s11104-013-1895-6
- Bertholdsson N-O, Kolodinska Brantestam A. 2009. A century of Nordic barley breeding – Effects on early vigour root and shoot growth, straw length, harvest index and grain weight. Eur J Agron. 30:266–274. doi:10.1007/s11104-013-1895-6
- Bogard M, Jourdan M, Allard V, Martre P, Perretant MR, Ravel C, Heumez E, Orford S, Snape J, Griffiths S, et al. 2011. Anthesis date mainly explained correlations between post-anthesis leaf senescence, grain yield, and grain protein concentration in a winter wheat population segregating for flowering time QTLs. J Exp Bot. 62:3621–3636. doi:10.1093/jxb/err061
- Bouwman AF, Boumans LJM, Batjes NH. 2002. Emissions of N2O and NO from fertilized fields: Summary of available measurement data. Glob Biogeochem Cy. 16:1058.
- Chapman SC, Barreto HJ. 1997. Using a chlorophyll meter to estimate specific leaf nitrogen of tropical maize during vegetative growth. Agron J. 89:557–562. doi:10.2134/agronj1997.00021962008900040004x
- Fageria NK, Baligar VC, Li YC. 2008. The role of nutrient efficient plants in improving crop yields in the twenty first century. J Plant Nutr. 31:1121–1157. doi:10.1080/01904160802116068
- [FAOSTAT] Food and Agriculture Organization of the United Nations. [cited 2015 Mar 30]. Available from: http://faostat3.fao.org/faostat-gateway/go/to/download/Q/QC/E
- Figueiredo N, Carranca C, Trindade H, Pereira J, Goufo P, Coutinho J, Marques P, Maricato R, De Varennes A. 2014. Elevated carbon dioxide and temperature effects on rice yield, leaf greenness, and phenological stages duration. Paddy Water Environ. doi:10.1007/s10333-014-0447-x
- Foulkes MJ, Hawkesford MJ, Barraclough PB, Holdsworth MJ, Kerr S, Kightley S, Shewry PR. 2009. Identifying traits to improve the nitrogen economy of wheat: recent advances and future prospects. Field Crops Res. 114:329–342. doi: 10.1016/j.fcr.2009.09.005
- Gaju O, Allard V, Martre P, Snape JW, Heumez E, LeGouis J, Moreau D, Bogard M, Griffiths S, Orford S, et al. 2011. Identification of traits to improve the nitrogen-use efficiency of wheat genotypes. Field Crops Res. 123:139–152. doi: 10.1016/j.fcr.2011.05.010
- Hagenblad J, Asplund L, Balfourier F, Ravel C, Leino M. 2012. Strong presence of the high grain protein content allele of NAM-B1 in Fennoscandian wheat. Theor Appl Genet. 125:1677–1686. doi: 10.1007/s00122-012-1943-2
- Kenward MG, Roger JH. 1997. Small sample inference for fixed effects from restricted maximum likelihood. Biometrics. 53:983–997. doi: 10.2307/2533558
- Kibite S, Evans LE. 1984. Causes of negative correlations between grain yield and grain protein concentration in common wheat. Euphytica. 33:801–810. doi: 10.1007/BF00021906
- Kichey T, Hirel B, Heumez E, Dubois F, Le Gouis J. 2007. In winter wheat (Triticum aestivum L.), post-anthesis nitrogen uptake and remobilisation to the grain correlates with agronomic traits and nitrogen physiological markers. Field Crops Res. 102:22–32. doi: 10.1016/j.fcr.2007.01.002
- Kirby EJM. 1988. Analysis of leaf, stem and ear growth in wheat from terminal spikelet stage to anthesis. Field Crops Res. 18:127–140. doi: 10.1016/0378-4290(88)90004-4
- Larsson S, Hagman J, Dryler K. 2013. Stråsäd, trindsäd, oljeväxter, potatis: sortval 2013. Uppsala: Institutionen för växtproduktionsekologi, SLU.
- Larsson S, Hagman J, Ericson L. 2008. Stråsäd, trindsäd, oljeväxter, potatis: sortval 2008. Uppsala: Institutionerna för växtproduktionsekologi respektive norrländsk jordbruksvetenskap, SLU.
- Le Bail M, Jeuffroy M-H, Bouchard C, Barbottin A. 2005. Is it possible to forecast the grain quality and yield of different varieties of winter wheat from Minolta SPAD meter measurements? Eur J Agron. 23:379–391. doi: 10.1016/j.eja.2005.02.003
- Liao M, Fillery IRP, Palta JA. 2004. Early vigorous growth is a major factor influencing nitrogen uptake in wheat. Funct Plant Biol. 31:121–129. doi: 10.1071/FP03060
- Liao M, Palta JA, Fillery IRP. 2006. Root characteristics of vigorous wheat improve early nitrogen uptake. Aust J Agric Res. 57:1097–1107. doi: 10.1071/AR05439
- Neugschwandtner RW, Böhm K, Hall RM, Kaul H-P. 2015. Development, growth, and nitrogen use of autumn- and spring-sown facultative wheat. Acta Agric Scand Sect B – Soil Plant Sci. 65:6–13.
- Ozturk A, Caglar O, Bulut S. 2006. Growth and yield response of facultative wheat to winter sowing, freezing sowing and spring sowing at different seeding rates. J Agron Crop Sci. 192:10–16. doi: 10.1111/j.1439-037X.2006.00187.x
- Peltonen J, Virtanen A, Haggrèn E. 1995. Using a chlorophyll meter to optimize nitrogen fertilizer application for intensively-managed small-grain cereals. J Agron Crop Sci. 174:309–318. doi: 10.1111/j.1439-037X.1995.tb01118.x
- Peltonen-Sainio P, Jauhiainen L, Nissilä E. 2012. Improving cereal protein yields for high latitude conditions. Eur J Agron. 39:1–8. doi: 10.1016/j.eja.2012.01.002
- Pourazari F, Vico G, Ehsanzadeh P, Weih M. Forthcoming 2015. Contrasting growth pattern and nitrogen economy in ancient and modern wheat varieties. Can J Plant Sci. doi:10.4141/CJPS-2014-260
- R Development Core Team. 2012. R: A language and environment for statistical computing. Vienna (Austria): R Foundation for Statistical Computing.
- Reynolds M, Foulkes MJ, Slafer GA, Berry P, Parry MAJ, Snape JW, Angus WJ. 2009. Raising yield potential in wheat. J Exp Bot. 60:1899–1918. doi: 10.1093/jxb/erp016
- SAS Institute Inc. 2011. SAS/STAT® 9.3 user's guide. Cary, NC: SAS Institute Inc.
- Semenov MA, Jamieson PD, Martre P. 2007. Deconvoluting nitrogen use efficiency in wheat: a simulation study. Eur J Agron. 26:283–294. doi: 10.1016/j.eja.2006.10.009
- Shewry PR. 2009. Wheat. J Exp Bot. 60:1537–1553. doi: 10.1093/jxb/erp058
- Slafer GA, Abeledo LG, Miralles DJ, Gonzalez FG, Whitechurch EM. 2001. Photoperiod sensitivity during stem elongation as an avenue to raise potential yield in wheat. Euphytica. 119:191–197. doi: 10.1023/A:1017535632171
- Spaner D, Todd AG, Navabi A, McKenzie DB, Goonewardene LA. 2005. Can leaf chlorophyll measures at differing growth stages be used as an indicator of winter wheat and spring barley nitrogen requirements in Eastern Canada? J Agron Crop Sci. 191:393–399. doi: 10.1111/j.1439-037X.2005.00175.x
- Swedish Board of Agriculture, Statistics Sweden. 2014. Production of cereals, dried pulses, oilseed crops, potatoes and temporary grasses in 2013. Final statistics. JO 16 SM 1401.
- Sylvester-Bradley R, Kindred DR. 2009. Analysing nitrogen responses of cereals to prioritize routes to the improvement of nitrogen use efficiency. J Exp Bot. 60:1939–1951. doi: 10.1093/jxb/erp116
- Thorup-Kristensen K, Salmeron Cortasa M, Loges R. 2009. Winter wheat roots grow twice as deep as spring wheat roots, is this important for N uptake and N leaching losses? Plant Soil. 322:101–114. doi: 10.1007/s11104-009-9898-z
- Tottman D. 1987. The decimal code for the growth-stages of cereals, with illustrations. Ann Appl Biol. 110:441–454. doi: 10.1111/j.1744-7348.1987.tb03275.x
- Uauy C, Distelfeld A, Fahima T, Blechl A, Dubcovsky J. 2006. A NAC gene regulating senescence improves grain protein, zinc, and iron content in wheat. Science. 314:1298–1301. doi: 10.1126/science.1133649
- Vitousek PM, Aber JD, Howarth RW, Likens GE, Matson PA, Schindler DW, Schlesinger WH, Tilman DG. 1997. Human alteration of the global nitrogen cycle: sources and consequences. Ecol Appl. 7:737–750.
- Watt M, Kirkegaard JA, Rebetzke GJ. 2005. A wheat genotype developed for rapid leaf growth copes well with the physical and biological constraints of unploughed soil. Funct Plant Biol. 32:695–706. doi: 10.1071/FP05026
- Weih M. 2014. A calculation tool for analyzing nitrogen use efficiency in annual and perennial crops. Agronomy. 4:470–477. doi: 10.3390/agronomy4040470
- Weih M, Asplund L, Bergkvist G. 2011. Assessment of nutrient use in annual and perennial crops: a functional concept for analyzing nitrogen use efficiency. Plant Soil. 339:513–520. doi: 10.1007/s11104-010-0599-4