Abstract
Growth scales give a standardized definition of crop development and increase the understanding among researchers and growers. In this research we defined a growth scale for the phasic development of common buckwheat that was mainly based on a sequence of easily recognizable changes occurring on the first and the terminal clusters of inflorescences formed on the main stem. Observations were carried out on plants grown in two years throughout spring. In an attempt to uniform the duration of phasic development across sowing dates, the length of phases and sub-phases was calculated in days and in thermal time using nine combinations of cardinal temperatures. A sequence of stages and various patterns of coordinated development were maintained throughout all sowings and years. Specifically, (1) the first inflorescence became visible after three true leaves had fully expanded on the main stem; (2) flowering reached the terminal inflorescence cluster before full-sized green fruits became visible in the first inflorescence, and (3) fruit ripening in the whole plant ended within two weeks of the end of ripening in the oldest inflorescence. Plant size was increased with the delay of sowing, and the length of the growth cycle was by approximately 400°Cd longer when plants experienced a day length longer than 15 h. This changed the correspondence between flowering and ripening stages, so that full flowering was associated with the development of green fruits in the first inflorescence when the cycle was short, but with their development in the terminal cluster when it was long. Trends in grain yield did not correspond to those in plant size and phase length. We are confident that this growth scale will be a valuable tool for following the progress of buckwheat development and to predict growth patterns and harvest time in response to temperature and photoperiod.
Abbreviations
SD | = | sowing date |
+INF | = | first inflorescence |
TINF | = | terminal cluster of inflorescences at the main stem apex |
GDD | = | growing degree-days |
Introduction
Common buckwheat (Fagopyrum esculentum Moench.), hereafter buckwheat, is a dicotyledonous annual herb of the family Polygonaceae. It is an ancient crop from Asia that was widely cultivated throughout Europe and North America up to the nineteenth century, but was later abandoned, especially in Western Europe (Campbell Citation1997). Today there is a renewed interest in this crop worldwide, due to its nutritional quality for humans and livestock. Buckwheat plants contain proteins with a high biological value, the flavonoid rutin, polysaccharides, dietary fibres, lipids, polyphenols, and micronutrients (minerals and vitamins) (Ahmed et al. Citation2014). In agro ecosystems, this species has an additional value, as it requires very low fertilizer inputs, it helps to suppress weeds, and it also offers a substantial late summer feed source for insects and honeybees (Amelchanka et al. Citation2010).
In most buckwheat ecotypes and cultivars, growth is indeterminate and the main stem consists of a highly variable number of metamers, 5–30, according to Cawoy et al. (Citation2009), each giving one leaf and one axillary bud per node. Plants produce a lot of flowers, but only a low proportion develop into dark-hulled triangular achenes (Halbrecq et al. Citation2005). Flower and fruit development are sequential, both within and between inflorescences, and the duration of corresponding phases is highly variable depending on the number of reproductive structures produced by the plant, which, in turn, is greatly influenced by environmental conditions (Taylor & Obendorf Citation2001; Michiyama et al. Citation2005). The morphology of buckwheat plants and the progress of flower development and achene ripening have been described in detail (Marshall & Pomeranz Citation1982; Funatsuki et al. Citation2000; Quinet et al. Citation2004; Halbrecq et al. Citation2005; Cawoy et al. Citation2009). However, to the best of our knowledge, the definition of a growth scale that describes buckwheat phasic development through a sequence of stabile and easily recognizable growth stages is lacking (Meier Citation2001). A primary reason is the great morphological plasticity in response to growth conditions, which is increased by the high level of genetic heterogeneity in most cultivars (Funatsuki et al. Citation2000; Michiyama et al. Citation2007).
Moreover, the timing of buckwheat phasic development has mainly been described in days or weeks after sowing (Campbell Citation1997; Funatsuki et al. Citation2000; Taylor & Obendorf Citation2001; Quinet et al. Citation2004; Halbrecq et al. Citation2005; Cawoy et al. Citation2009; Baumgertel et al. Citation2010; Gupta et al. Citation2011; Ahmed et al. Citation2014), which does not take into account the well-known influence of temperature and day length on this crop. Only a few attempts have related phasic development with thermal time and none with day length. Seed maturation required approximately 1200°Cd in the USA at 47° north latitude, with a base temperature of 5°C (Edwardson Citation1995), and 700°Cd in Slovenia, with a base temperature of 10°C (Kajfež-Bogataj Citation1988). According to Cha et al. (Citation1989), Korean buckwheat required approximately 1100°Cd to reach optimum seed harvest when planted on July 11, but only 950–1000°Cd when planted 10–20 days later.
The spread of buckwheat cultivation in different climatic regions entails substantial shifts from conventional sowing dates, thus exposing plants to novel combinations of day length and temperature. Therefore, an unambiguous definition of growth stages and an estimate of the duration of development phases, as much as possible independent of local conditions, are of crucial interest.
The aim of this study is to give a standardized definition of the chronological sequence of growth stages that occur in buckwheat independently of sowing date. The influence of sowing date on growth habit was evaluated through changes in plant height, the number of inflorescences and grain yield. In addition, in order to define a general model for the timing of phasic development, the duration of phases was calculated in days and in thermal time, by testing several combinations of cardinal temperatures.
Material and methods
Environmental conditions
The research was carried out in 2013 and 2014 at the Department of Agriculture, Food and Environment of the University of Pisa (Italy, 43°40′N, 10°19′E), at a distance of approximately 4 km from the sea and 6 m above sea level. Photoperiod ranges from 8 h 46 min to 15 h 14 min and the day length exceeds 15 h from 1 June to 13 July. Mean annual maximum and minimum daily air temperatures calculated from 1878 to 2012 are 20.2 and 9.5°C, respectively, and mean rainfall is 989 mm per year. According to Köppen (Citation1936), the climate is classified as Csa, humid temperate with dry and hot summers. Over the research seasons (21 March–21 September) of the two years, the mean maximum and minimum daily air temperatures were approximately 25.5 and 12.5°C, respectively, and were both by 0.5°C lower in 2014 than in 2013. Rainfall over the same period was markedly higher in 2014, 434 mm compared to 316 mm.
Treatments
The European buckwheat variety ‘Lileja’ was used, because of its stabile yield, and its widespread cultivation throughout Europe (Brunori et al. Citation2006; Goeritz et al. Citation2009; Kälber et al. Citation2012). Plants were collected from field trials and, in 2014, also from PVC pots (30 cm diameter, 35 cm height) that were filled with the same soil of the field trial. The pot experiment was established to follow more frequently and precisely the progress of phenological development on distinct tagged plants. Main soil physical and chemical properties were 51.1% sand (2 mm > Ø > 0.05 mm), 38.6% silt (0.05 mm > Ø > 0.002 mm), 10.3% clay (Ø < 0.002 mm), 8.2 pH (H2O), 22.6 g kg−1 organic matter (Walkley and Black method); 14.2 g kg−1 total CaCO3 (Scheibler method), 0.91 g kg−1 total nitrogen (Kjeldhal method), 10.2 mg kg−1 available P (Olsen method), 162.4 mg kg−1 available K (ammonium acetate test method). Before tillage and at pot filling, phosphorous fertilizer was applied at a rate of 44 kg P ha−1 as triple superphosphate, and potassium fertilizer at a rate of 83 kg K ha−1 as K2SO4. Nitrogen fertilizer was applied as urea, at a rate of 30 kg N ha−1, just before seeding. Pots were placed in the open air and, throughout the entire experiment, they were regularly watered and kept free of weeds by hand hoeing.
In the field, 200 viable seeds m−2 were sown at 15-cm row spacing in 3 × 5 m plots in both years. In pots, 20 seeds were sown and then thinned to 10 seedlings at the cotyledon stage, which corresponded to a final density of approximately 140 plants m−2. In 2013 sowing dates (SD) were 8 April (SD IIa) and 27 May (SD IVa), while in 2014 there were five sowing dates, performed at 20-day intervals throughout spring: SD I, 24 March; SD II, 14 April; SD III, 5 May; SD IV, 26 May and SD V, 17 June. For each sowing date five pots and a total of 50 plants were arranged.
Data collection
Weather data, daily minimum and maximum temperatures and rainfall, were collected from a weather station located close to the experimental site.
In the field, the phasic development and the timing of the growth stages were recorded on 10 plants, collected at one-week intervals after the complete emission of the first true leaf. In pots, all plants were observed at one- to three-day intervals starting from emergence. A stage was considered achieved when at least six out of ten plants had reached the specific stage in question. When existing, the terminology previously reported for buckwheat was used for plant descriptions (Funatsuki et al. Citation2000; Quinet et al. Citation2004; Halbrecq et al. Citation2005; Michiyama et al. Citation2007). The main stem nodes were numbered acropetally (N1, N2, etc.), starting from the node producing the first true leaf (). The cotyledon node was indicated as N0. Leaves were numbered according to corresponding nodes, as L1, L2 and so on. The first inflorescence was indicated as +INF. Uppermost racemes, whose subtending leaves were shorter than 1 cm, were considered together as a terminal inflorescence cluster (TINF), and the most basal node of this cluster was taken as the origin.
Figure 1. Schematic drawing of plant architecture. Numbers indicate sequential metamer development. N, node; L, leaf; +INF, first inflorescence; TINF, terminal inflorescence cluster. Crossed ovals represent cotyledons; grey ovals, axillary buds; blank ovals, inflorescences.
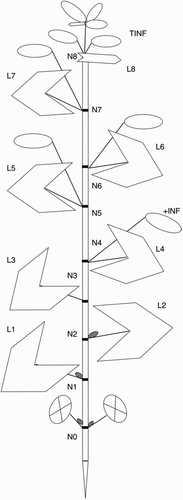
In the scale, we included only the growth stages that were clearly recognizable in all plants and were independent of sowing date. Thus, lateral branching was not reported. Whenever possible, codes assigned to growth stages and their definition followed the general scheme of the subdivision of plant developmental cycles reported in the extended BBCH-scaleFootnote1 (Hess et al. Citation1997; Meier Citation2001).
At maturity, five replicates each consisting of 10 plants were collected both from the field trials and from pots, and the position of the nodes corresponding to +INF and TINF, the length of the main stem, the total number of inflorescences and grain yield were determined.
Calculation and statistics
Thermal time was calculated as the sum of heat units measured in growing degree-days (GDD, °Cd), as GDD = [(Tmax + Tmin)/2] – Tb. In the formula, Tmax and Tmin are the daily maximum and minimum air temperatures, and Tb is the base temperature below which no significant crop development occurs. If Tmin < Tb then Tmin = Tb was also incorporated into the equation. An upper threshold temperature (Tut), above which crop development is negatively affected, was also incorporated, i.e. if Tmax > Tut then Tmax = Tut (McMaster & Wilhelm Citation1997).
Since cardinal threshold temperatures are not well defined for buckwheat, we used several combinations of Tb and Tut, in order to find the one that minimized the variation in the duration of phases over the sowing dates. Tested base temperatures were 0, 5 and 10°C (Kajfež-Bogataj Citation1988; Edwardson Citation1995; Campbell Citation1997), the latter generally indicated as Tb for summer crops in the Mediterranean region. For the upper threshold temperature, we tested 20, 25 and 30°C which are reported to be critical for flower fertilization by several authors (Marshall & Pomeranz Citation1982; Tahir & Farouk Citation1988; Cawoy et al. Citation2009). In summary, nine temperature combinations were tested: 0–20°C, 0–25°C, 0–30°C, 5–20°C, 5–25°C, 5–30°C, 10–20°C, 10–25°C and 10–30°C.
The average growth rate of metamers during the vegetative phase was calculated as the ratio of the thermal time from sowing to the stage 1st flowers open (0–60) and the position of the node corresponding to +INF. The average growth rate of metamers, or inflorescences, during the reproductive phase was calculated as the ratio of the thermal time from the stage 1st flowers open to the end of fruit ripening (60–88) and the difference between the positions of nodes TINF and +INF, or the number of inflorescences, respectively.
The effect of sowing date on biometric parameters and on the duration of phases, calculated both in days and thermal time, was analysed by means of simple ANOVA with five replicates. Means were separated by the least significant difference (LSD) test, when the F test indicated factorial effects on the significance level of p < .05 (Steel et al. Citation1997).
Results
Definition of growth stages
Following the decimal code and the definitions of growth stages of the extended BBCH scale, we elaborated a growth scale for buckwheat, choosing the ordered sequence of growth stages that was observed in plants, independently of their size, year of cultivation and sowing date ().
Table 1. A scale for buckwheat growth stages. Codes and descriptions follow the extended BBCH scale.a In brackets the three-digit code for leaf development. Since flowering and fruiting phases overlap for a long period, distinct codes were reported in two parallel columns. A visual scale is reported in Appendix 1.
In buckwheat, germination is epigeal and, therefore, emergence (stage 09) is closely followed by the unfolding of cotyledon leaflets (stage 10). From our observations seedlings are established with the complete emission of the first true leaf (stage 11), after which the shoot apical meristem produces a sequence of main stem metamers, each consisting of one internode and one node with one leaf and one axillary bud. Since we found that plants produced approximately seven main stem metamers when they were sown between 24 March and 5 May (SD I, II, IIa and III), but approximately 12 when they were sown after 20 May (SD IV, IVa and V) a three-digit code was introduced to describe leaf development. Accordingly, the stage 1st leaf unfolded is indicated both with the two-digit code 11 and the three-digit code 101, while the stages of leaf 10, 11 and 12 correspond to the codes 110, 111 and 112, respectively. The principal growth stage 2, describing the progress of lateral branching, was not detailed in this scale, because branching was highly variable among plants and in response to sowing dates, both in terms of first branch position, which varied from the cotyledon node to that immediately below the first formed inflorescence, and in terms of rate and duration of branch development ().
Figure 2. Different branching of buckwheat plants at the stages third leaf (stage 13) and blossoming (stage 50). a, no branches (SD I); b, four branch primordia (SD IV).
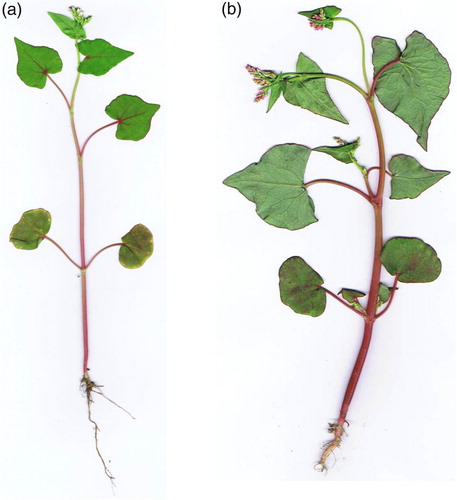
Similar to branching, in this research, the number of inflorescences per plant varied greatly in response to sowing date. However, the first (+INF) and the terminal clusters (TINF) of inflorescences formed on the main stem were easy to detect and to follow in their progress in all plants and, therefore, we based reproductive stages on the changes occurring on them. In addition, because of the long-lasting and widely overlapping flowering and ripening phases, we defined different stages and sub-phases for each, and, after the appearance of the first green fruits at the base of +INF (stage 70), the growth scale proceeds with two parallel sequences of stages, each with distinct codes.
In buckwheat, the shift to reproduction is signalled by the appearance of a blossom bundle through the upper unfolding leaf at the main stem apex (stage 50). In our research this varied from L4 to L5, and the reproductive phase always started with the opening of the most basal flower in the 1st main stem inflorescence +INF (stage 60). Despite this, in the growth scale, we set beginning of flowering (stage 62) when 1–2 flowers were open in the terminal cluster of inflorescences TINF, because this stage is easier to record in field conditions. The percentage of flowers open in the plant is highly variable in buckwheat in response to branching and was not reported. Nevertheless, we observed that at full flowering (stage 65), at least 1–2 flowers were open in each of the main stem inflorescences and in most inflorescences of the lateral branches. Advanced flowering (stage 66) corresponds to the end of flowering in +INF. It is followed by late main stem flowering (stage 67) when most main stem inflorescences have no more open flowers and only 1–2 flowers are open at the same time in TINF. The end points of flowering on the main stem (stage 68) and throughout the whole plant (stage 69) were hard to identify, because blossoms and flowers were still present in senescent plants, especially in later sowing dates [(a)].
Figure 3. Inflorescence TINF (a) showing mature fruits and flowers (SD III). Inflorescence +INF (b) showing all stages of flowering and fruit ripening (SD V).
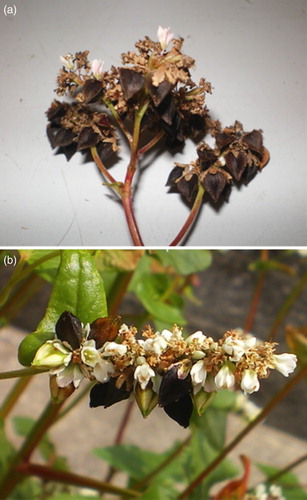
In line with the flowering phase, the first full-developed green achenes appeared at the base of +INF (stage 70), but we set the beginning of fruit development (stage 71) when full-sized green achenes became visible in TINF, and the beginning of fruit ripening (stage 80) when angles of these achenes turned brownish-red. In contrast, ripening stages were defined on the oldest inflorescence, because the sequence of ripening was more ordered in +INF than in TINF. Accordingly, the appearance of the first brown fruits (stage 85) was recorded in +INF, and advanced ripening (stage 86) corresponds to the stage all achenes in this inflorescence are brown. In sequence, late fruit ripening (stage 87) indicates the complete ripening or abortion of all achenes of the main stem inflorescences, and the end of fruit ripening (stage 88) when this occurs on the whole plant.
At last, we indicated the yellowing and withering of leaf margins as the beginning of plant senescence (stage 90), whereas plants were considered dead (stage 97) when the stem was entirely brown and fragile. It is worth noting that the senescence phase was always rather long, ranging approximately from 20 to 30 days.
Observations performed on plants of the pot experiment showed that the correspondence between various flowering and ripening stages was maintained throughout all the sowing dates (). Specifically, (1) the first inflorescence became visible (stage 50) when at least three true leaves had fully expanded on the main stem (stage 13); (2) flowering reached the terminal inflorescence cluster (stage 62) always before full-sized green fruits became visible in +INF (stage 70), and (3) fruit ripening in the whole plant ended within two weeks of the end of ripening in the oldest inflorescence.. Otherwise, the sowing date changed the chronological sequence of some stages, thus affecting their correspondence. In particular, 1st brown fruits visible in +INF (stage 85) occurred when no more flowers were open in this inflorescence (stage 66) in SD I and II, but not in the later sowings, where flowers and fruits at all developmental stages coexisted in the inflorescence [(b)]. Accordingly, (1) stage 85 was associated with the beginning of plant senescence (stage 90) in SD I and II, whereas it followed closely full flowering (stage 65) and the appearance of green fruits in TINF (stage 71) in SD III, IV and V. In turn, (2) stage 65 was associated with the development of 1st green fruits in +INF (stage 70) in SD I and II, but with their development in TINF (stage 71) in SD III, IV and V. Finally, the progress of lateral branching did not match the main stem stages, except that the first lateral branch primordium became visible approximately after three leaves had expanded on the main stem (stage 13) and around blossoming (stage 50).
Table 2. Timing of principal growth stages of buckwheat plants sown at 20-day intervals throughout spring 2014. Reported dates were averaged over five replicate pots.
Growth parameters
Sowing date influenced the growth habit of buckwheat plants. In both years, the first inflorescence developed at the fourth node of the main stem (N4) in plants sown up to beginning of May (SD I, II, IIa and III), and at the fifth node (N5) in later sowings (SD IV, IVa and V), while the terminal cluster developed approximately at N7 in the four earlier SD and at N12 in the later three. Note that the node of the terminal cluster also indicated the total number of main stem metamers formed by the apical meristem.
Measured growth parameters did not differ statistically between pot or field conditions and, therefore, we reported field data from 2013 and the average of field and pot data for 2014. Moreover, also between year differences in the April (SD II and IIa) and end of May (SD IV and IVa) sowings were not significant, probably because temperatures did not differ markedly in 2013 and 2014 and irrigation nullified differences in rainfall. In plants sown from 24 March to 5 May (SD I, II, IIa and III) the main stem height and the number of inflorescences per plant did not differ statistically and were approximately 62 cm and 8.7, respectively (). When sowing was delayed (SD IV, IVa and V), the average stem height increased to 93 cm, while the number of inflorescences per plant was approximately 22 in sowings performed at the end of May (SD IV, IVa), and 38 in that of mid-June (SD V). Grain yield was greatly affected by sowing date: it decreased progressively from 1.9 to 0.1 g plant−1 with its delay from March (SD I) to beginning of May (SD III) and increased again in later sowings, reaching 3.6 g plant−1 with the mid-June one (SD V). Between year differences were approximately 0.5 g plant−1, but were not statistically significant.
Length of growth phases
In buckwheat plants sown in spring, the length of the entire growth cycle, from sowing to the end of fruit ripening (stages 0–88), was not markedly affected by year and sowing date, and was 80 and 87 days in 2013, and ranged from 80 to 91 days in 2014 (). In the second year, the duration of the vegetative phase (stages 0–60) decreased by approximately 21% with the delay of sowing up to SD III, and then remained unchanged, while that of the reproductive phase (stages 60–88) increased by approximately 28% from SD I and II to SD III, and then decreased by 13% in SD IV and V.
Table 3. Duration of the entire growth cycle and of the vegetative (0–60) and reproductive (60–88) phases in days and thermal times (GDD), as affected by sowing date (SD) in 2014. Thermal time is reported for the Tb and Tut intervals 0–20°C and 5–25°C.
With all combinations of cardinal temperatures, the amount of GDD needed to complete both the vegetative and the reproductive phases differed significantly in response to sowing date (). In contrast, between year differences in the amount of accumulated GDD at corresponding sowing dates, II–IIa and IV–IVa, were always lower than 10°Cd and were not reported. In 2014, the thermal time to complete the growth cycle was lower in plants sown in March–April (SD I and II) compared to those sown after mid-May (SD IV and V). In plants sown at the beginning of May (SD III) the thermal time of the vegetative phase equalled that of the earlier SD, and the reproductive phase, that of later SD. Accordingly, two types of growth cycle length were found in response to sowing date: short thermal time in sowings performed in March and April (SD I, II and IIa), and long thermal time in sowings performed in May and June (SD III, IV, IVa and V).
The combination of cardinal temperatures 0–25°C uniformed best the thermal time of the entire growth cycle within each group of SD. Differences were only 13°Cd within early sowings (SD I, II and IIa) and 10°Cd within later ones (SD III, IV, IVa and V), corresponding to a variation of less than 1%. With the interval 5–25°C, differences were slightly higher, approximately 3% ().
The higher amount of GDD accumulated during the vegetative phase in SD IV, IVa and V depended on the higher position of +INF on the main stem, which was N5 compared to N4. We observed, indeed, that up to the stage of first flowers open, the average thermal time needed by buckwheat to produce main stem complete metamers (internode + leaf + lateral branch primordium) was constant across all tested SD and was 117°Cd metamer−1 (±2.4 SE) with the cardinal interval 0–25°C. In contrast, we did not find a constant ratio between the thermal time of the reproductive phase and the number of main stem metamers, or the number of inflorescences produced during that period.
Since reproductive sub-phases overlap widely in buckwheat, in 2014, we calculated the thermal time of each separately, in order to assess whether their duration was affected differently by temperature and sowing date. The thermal time of main stem flowering (60–68) was approximately twice as long in SD III, IV and V compared to earlier sowings, and differences within these two groups of SD were lowest with the cardinal intervals 5–25°C and 5–30°C (). Similar patterns were observed for the amount of GDD required by first flowers to develop into full-sized green achenes (60–70), but differences within groups were lowest with 0–25°C. The additional amount of GDD needed by green achenes to reach full maturity (70–85) was higher than that needed to reach full size (60–70), and was quite uniform throughout sowing dates. Differences were lowest with the interval 5–30°C and with all combinations including a base temperature of 10°C. Finally, late flowering (68–69) and main stem fruits ripening (85–87) lasted significantly more in SD III than in other sowings, and no significant differences were detected between SD I and II and between SD IV and V, irrespectively of the temperature interval tested. However, differences in the length of phase 68–69 were lowest with the intervals 5–25°C, while those of phase 85–87 with 10–25°C.
Table 4. Duration of reproductive sub-phases in days and thermal times (GDD), as affected by sowing date (SD) in 2014. Thermal time is reported for the Tb and Tut intervals that performed best at reducing differences among sowing dates.
Discussion
Despite the well-known heterogeneity of buckwheat plants and the marked influence of sowing date on growth habit and phase length (Funatsuki et al. Citation2000; Michiyama et al. Citation2007), the ordered sequence of development stages reported in the growth scale was recorded in all examined plants. Accordingly, up to the start of flowering, the phasic development of buckwheat can be described as a sequence of metamers produced acropetally, each consisting in one internode, one leaf and one lateral branch primordium. Thereafter, plants grow further by developing acropetally, main stem metamers, consisting in one internode, one leaf and one inflorescence, and, basipetally, lateral branches, in general one or two per node.
The differences in temperature and day length, experienced by buckwheat plants with the delay of sowing from the beginning to the end of spring, increased the number of main stem metamers and the growth of lateral branches, which caused a marked increase in plant height and in the number of inflorescences per plant. This affected the length of phases during the entire cycle, but changes were more pronounced after the start of flowering. Differences in the duration of phases and sub-phases were never reduced, and even increased when they were expressed in thermal time, highlighting that temperature was not the primary factor driving buckwheat development.
Though the thermal time of the vegetative phase was significantly higher in SD IV and V compared with earlier SD, the amount of GDD required for the development of one complete metamer was constant across sowing dates up to the stage first flowers open (stage 60). This confirms that the duration of the vegetative phase is regulated by both temperature and photoperiod and, in specific, that the former determines the growth rate of metamers, and the latter the number of metamers produced below the first inflorescence. The cardinal temperature interval 0–25°C uniformed best the length of the vegetative phase, suggesting that temperatures between 0 and 5°C were not detrimental to early growth, in contrast to findings in the literature (Kajfež-Bogataj Citation1988; Edwardson Citation1995).
Present results were obtained with the European variety Lileja in a the temperate Mediterranean environment, but similar to our findings, Edwardson (Citation1995), with the varieties Mancan and Manor in the USA, and Cha et al. (Citation1989) in Korea with local varieties, reported that the length of the phase from sowing to the beginning of flowering depended strongly on mean temperature, while this did not occur for the reproductive phase. In Japan, Michiyama et al. (Citation2005) found that plants exposed to long days before the start of anthesis prolonged the vegetative phase and elevated the first flowering node, and also indicated that a 14-h day length was critical for the summer ecotype Shinanonatsusoba and 13-h for the autumn one Miyazakizairai. In the variety Lileja, we found that the position of the first inflorescence increased from N4 to N5 when plants reached floral transition, corresponding to the expansion of the first true leaf (Quinet et al. Citation2004), after 1 June (SD IV, IVa and V) and, therefore, only when they experienced a day length longer than 15 h.
In line with findings of Michiyama et al. (Citation2003), in this research, only plants exposed to the long days during the vegetative phase increased main stem height and the number of metamers produced during the reproductive phase, suggesting that both parameters are determined in buckwheat at initial growth stages. In contrast, the number of inflorescences per plant showed a progressive trend with the increase of day length, and was by far higher in plants sown on 17 June compared to those sown on 26 May. Since the number of main stem metamers equalled in the two SD, the different number of inflorescences was probably due to a greater development of lateral branches that are, therefore, regulated differently than the main stem by temperature and photoperiod
Plants sown from May onwards (SD III, IV and V) required a higher thermal time to complete all flowering and ripening sub-phases, except for the ripening of first green achenes (phase 70–85) that was constant across sowing dates. The cardinal intervals that best uniformed the length of this sub-phase were higher than those of other sub-phases, 10–25°C and 5–30°C, suggesting that fruit ripening needs higher temperatures than vegetative growth, flowering and fruit growth. This explains the findings of Funatsuki et al. (Citation2000) that low temperatures during seed ripening prolonged the time of maturation in buckwheat cultivars with both determinate (Kitawase D) and indeterminate (Kitawaseoba) growth habit, and were a primary cause of reduced grain yield in late summer sowings.
The longer duration of the flowering and fruit growth phases could not be attributed to differences in the number of main stem metamers or in the number of inflorescences produced, since both parameters differed greatly between SD III and the later sowings. Thus, other parameters such as the number of flowers produced by each inflorescence and factors affecting seed setting should be taken into account (Taylor & Obendorf Citation2001). Michiyama (Citation2005) reported that day length was a primary cause of differences in flower and grain production, and that grain yield was reduced by long days, especially in the autumn ecotype. In this research, however, variations in grain yield corresponded neither to trends observed in the length of vegetative and reproductive phases and sub-phases, nor in plant height and in the number of inflorescences. Nevertheless, the highest yield was obtained in SD V in correspondence with long vegetative and reproductive phases and with the highest number of inflorescences. Our findings contrast with the hypothesis of Quinet et al. (Citation2004) that for grain yield it would be advantageous to grow buckwheat in short days, and suggest that yield is more stabile in short days, but best performances are obtained with long days.
The response of buckwheat to long days has been investigated by testing constant day lengths in Japanese summer and autumn ecotypes and in the European variety La Harpe (Michiyama et al. Citation2003; and Michiyama et al. Citation2005; Quinet et al. Citation2004), whereas, to the best of our knowledge, increasing or declining day lengths have never been taken into account. One reason for this could be that buckwheat is generally cultivated as a short-day summer–autumn crop throughout the world (Campbell Citation1997; Slawinska & Obendorf Citation2001; Michiyama et al. Citation2007) and, therefore, plants grow mostly in declining day lengths. We found, however, that differences in the thermal time of phases were also associated with trends in day length, and plants prolonged growth phases when they experienced, at least for a period, declining day length. When this occurred, either during the reproductive phase only (SD III) or in both the vegetative and the reproductive phases (SD IV, IVa and V), the entire growth cycle was longer than in plants that grew entirely in increasing day length (SD I, II and IIa). In particular, we found that the number of inflorescences per plant and also grain yield were highest when plants were entirely grown in declining day length (SD V).
Irrespectively of the photoperiodic signal, however, the mechanism controlling plant growth and, consequently, the duration of phases is still unclear. Cawoy et al. (Citation2009) hypothesized that the availability of resources internal to the inflorescence regulates the percentage of fertile flowers, while Taylor and Obendorf (Citation2001) found that the frequency of flower fertilization declined with plant age, but was not related to the amount of developing fruits in the inflorescence. We did not perform a quantitative analysis; however, we suggest that the first formed inflorescence (+INF) plays a key role in determining the length of the entire growth period. Indeed, we observed that the complete ripening, or abortion, of fruits in this inflorescence (stage 86) was closely followed by plant senescence and that, consistently with findings of Michiyama et al. (Citation2005), a long growth cycle was associated with a high number of flowers in that inflorescence. Further investigations are needed to elucidate this point.
To sum up, we found that sowing time changed the growth habit of buckwheat plants and the amount of GDD required to complete the development phases. Accordingly, two types of growth cycle length were highlighted. In plants sown in March and April (SD I, II and IIa), the vegetative and the reproductive phases were both short, and the thermal time of the entire cycle was 900–950°Cd with a cardinal interval 5–25°C. In contrast, in plants sown in May and June either the reproductive phase (SD III) or both (SD IV, IVa and V) were long, and the cycle lasted 1270–1300°Cd. These last values are consistent with those reported for buckwheat sown in May in the north of the USA (Edwardson Citation1995) and can, therefore, be used to estimate the optimal time for fruit harvest when buckwheat is grown in long days.
Changes in growth habit and the different duration of flowering in response to sowing date affected the relation between flowering and ripening stages. This could impair the use of easily recognizable growth stages for the identification of optimal harvest times, in particular for the maximum accumulation of biomass in stem and leaves, which is generally associated with the peak of flowering (Cawoy et al. Citation2009). However, we observed that, with a short cycle, full flowering (stage 65) corresponded to the appearance of first green fruits in +INF (stage 70), while, with a long cycle, it corresponded to the appearance of first green fruits in TINF (stage 71), because of the later flowering on the lateral branches. Since green leaves and flowers retain the highest rutin concentration within the plant (Baumgertel et al. Citation2010; Gupta et al. Citation2011; Ahmed et al. Citation2014), the stages 70 and 71 can be used to identify the best harvest time for both forage and rutin yield, when plants are grown in short or long days, respectively.
Especially in long cycle plants, the optimal time for fruit harvest is difficult to identify, because of sequential ripening and the overlap with seed shattering (Edwardson Citation1995; Funatsuki et al. Citation2000). From our observations, the best time for fruit harvest seems to be when no more green achenes are present in the TINF cluster (stage 87). At this stage, all the main stem inflorescences are ripe, while there may still be green achenes on the lower branches. In line with findings of Taylor and Obendorf (Citation2001), however, these never developed into fruits and, thus, they do not contribute to seed yield. Our calculations suggest that, taking a cardinal temperature interval of 10–25°C, fruit harvest can be planned approximately 160°Cd after the appearance of the first brown achenes in +INF (stage 85), when plants reach full flowering (stage 65) with a day length shorter than 15 h, i.e. before 1 June in this study, but approximately 370°Cd after stage 85 when they flower later.
In conclusion, despite the rather high heterogeneity of plants and the influence of sowing date on growth parameters, the phasic development of buckwheat can be described through an ordered sequence of growth stages that are mainly based on easily recognizable changes occurring in the first and the terminal clusters of inflorescences formed on the main stem. Sowing date did not affect the occurrence of stages, but changed the plant growth habit and the correspondence between flowering and ripening stages. In this study, plants of the European variety Lileja, exposed to day length longer than 15 h at initial growth stages, showed increased size and more profuse flowering, and required a longer thermal time to complete all phases. However, large size and long flowering corresponded to high grain yield only in plants sown in mid-June, so we hypothesize that other factors, such as declining photoperiod, may influence seed setting. Though quantitative analyses are necessary to confirm our statements, we suggest a correspondence of ripening growth stages with the optimal time for forage and fruit harvest. The stage for forage harvest, generally coinciding with full flowering, changes with the length of the growth cycle and, therefore, with sowing date. In specific, it corresponds to the appearance of green achenes in the oldest inflorescence (stage 70) in short growth cycles, and with their appearance in the terminal cluster of inflorescences (stage 71) in long ones. The best time for grain harvest, indeed, corresponds to the complete ripening of achenes on the main stem inflorescences (stage 87) with all sowing dates and cycle length.
Disclosure statement
No potential conflict of interest was reported by the authors.
Notes
1. The abbreviation BBCH indicates the institutions that jointly developed this scale: BBA (Biologische Bundesanstalt fur Land- und Forstwirtschaft – German Federal Biological Research Centre for Agriculture and Forestry); BSA (Bundessortenamt – German Federal Variety Authority); CHemical Industry (Industrieverband Agrar – German Association of Manufacturers of Agrochemical Products).
References
- Ahmed A, Khalid N, Ahmad A, Abbasi NA, Latif MSZ, Randhawa MA. 2014. Phytochemicals and biofunctional properties of buckwheat: a review. J Agr Sci (Camb.). 152:349–369. doi: 10.1017/S0021859613000166
- Amelchanka SL, Kreuzer M, Leiber F. 2010. Utility of buckwheat (Fagopyrum esculentum Moench) as feed: effects of forage and grain on in vitro ruminal fermentation and performance of dairy cows. Anim Feed Sci Technol. 155:111–121. doi: 10.1016/j.anifeedsci.2009.10.007
- Baumgertel A, Loebers A, Kreis W. 2010. Buckwheat as a source for the herbal drug Fagopiriherba: rutin content and activity of flavonoid-degrading enzymes during plant development. Eur J Plant Sci Biotechnol. 4( Special Issue I):82–86.
- Brunori An, Brunori Al, Baviello G, Marconi E, Colonna M, Ricci M, Mandarino P. 2006. Yield assessment of twenty buckwheat (Fagopyrum esculentum Moench and Fagopyrum tataricum Gaertn.) varieties grown in central (Molise) and southern Italy (Basilicata and Calabria). Fagopyrum. 23:83–90.
- Campbell CG. 1997. Buckwheat. Fagopyrum esculentum Moench. Promoting the conservation and use of underutilized and neglected crops. 19. Rome (I): International Plant Genetic Resources Institute.
- Cawoy V, Ledent JF, Kinet JM, Jacquemart AL. 2009. Floral biology of common buckwheat (Fagopyrum esculentum Moench). Eur J Plant Sci Biotechnol. 3 ( Special Issue I):1–9.
- Cha SW, Cha YH, Rho CW, Kim TS, Kwon KC. 1989. Variation of yield related characters on different planting times in local buckwheat Fagopyrum esculentum Moench. Upland Ind Crops. 31:43–49.
- Edwardson SE. 1995. Using growing degree days to estimate optimum windrowing time in buckwheat. In: Matano T, Ujihara A, editors. Current Advances in Buckwheat Research, Proceedings of the Sixth International Symposium on Buckwheat. Shinshu (China): Shinshu University Press; p. 509–514. 24–29 August.
- Funatsuki H, Maruyama-Funatsuki W, Fujino K, Agatsuma M. 2000. Ripening habit of buckwheat. Crop Sci. 40:1103–1108. doi: 10.2135/cropsci2000.4041103x
- Goeritz M, Kawiani R, Loges R, Schwarz K, Kämper M, Ehmsen T, Taube F. 2009. Ertragsleistung und RutingehalteverschiedenerBuchweizensortenunterökologischenAnbaubedingungen [Grain yield and rutin content of different buckwheat varieties in organic farming]. In: Mayer J, Alföldi T, Leiber F, Dubois D, Fried P, Heckendorn F, Hillmann E, Klocke P, Luscher A, Riedel S, Stolze M, Strasser F, van der Heijden M, Willer H, editors. Werte - Wege - Wirkungen: BiolandbauimSpannungsfeldzwischenErnährungssicherung, Markt und Klimawandel, vol. 1. Boden, Pflanzenbau, Agrartechnik, Umwelt- und Naturschutz, Biolandbau international, Wissensmanagement. Proceedings of the 10th Wissenschaftstagung Ökologischer Landbau; 11–13 February 2009; Zurich (CH). Berlin (D): Verlag Dr. Köster; p. 215–218.
- Gupta N, Sharma SK, Rana JC, Chauhan RS. 2011. Expression of flavonoid biosynthesis genes vis-à-vis rutin content variation in different growth stages of Fagopyrum species. J Plant Physiol. 168:2117–2123. doi: 10.1016/j.jplph.2011.06.018
- Halbrecq B, Romedenne P, Ledent JF. 2005. Evolution of flowering, ripening and seed set in buckwheat (Fagopyrum esculentum Moench): quantitative analysis. Eur J Agron. 23:209–224. doi: 10.1016/j.eja.2004.11.006
- Hess M, Barralis G, Bleiholder H, Buhrs L, Eggers Th, Hack H, Stauss R. 1997. Use of the extended BBCH scale – general for the descriptions of the growth stages of mono- and dicotyledonous weed species. Weed Res. 37:433–441. doi: 10.1046/j.1365-3180.1997.d01-70.x
- Kajfež-Bogataj L. 1988. A simple model for allocation of assimilates at different stages of buckwheat plant development. Fagopyrum. 8:23–26.
- Kälber T, Kreuzer M, Leiber F. 2012. Silages containing buckwheat and chicory: quality, digestibility and nitrogen utilisation by lactating cows. Arch Anim Nutr. 66:50–65. doi: 10.1080/1745039X.2011.630213
- Köppen W. 1936. Das geographische System der Klimate [The geographic system of climates]. In: Koppen W, Geiger R, editors. Handbuch der Klimatologie [Handbook of climatology], vol. 1. Part C. Berlin: Gebruder Borntraeger; p. 1–44.
- Marshall HG, Pomeranz Y. 1982. Buckwheat: description, breeding, production and utilization. In: Pomeranz Y, editor. Advances in cereal science and technology, vol. 5. St. Paul, Minnesota: American Association of Cereal Chemists Incorporated; p. 157–210.
- McMaster GS, Wilhelm WW. 1997. Growing degree-days: one equation, two interpretations. Agric For Meteorol. 87:291–300. doi: 10.1016/S0168-1923(97)00027-0
- Meier U. 2001. BBCH monograph. Growth stages of mono- and dicotyledonous plants. 2nd edition. Federal Biological Research Centre for Agriculture and Forestry. Berlin (D): Blackwell.
- Michiyama H, Arikuni M, Hirano T, Hayashi H. 2003. Influence of day length before and after the start of anthesis on the growth, flowering and seed-setting in common buckwheat (Fagopyrum esculentum Moench). Plant Prod Sci. 6:235–242. doi: 10.1626/pps.6.235
- Michiyama H, Tsuchimoto K, Tani KI, Hirano T, Hayashi H, Campbell CG. 2005. Influence of day length on stem growth, flowering, morphology of flower clusters, and seed-set in buckwheat (Fagopyrum esculentum Moench). Plant Prod Sci. 8:44–50. doi: 10.1626/pps.8.44
- Michiyama H, Yoshimura K, Hirano T, Hayashi H. 2007. Influence of air temperature on varietal differences between summer and autumn ecotype in buckwheat. Proceedings of the 10th International Symposium on buckwheat. Yanhling, Shaanxi, China, p. 242–246.
- Quinet M, Cawoy V, Lefèvre I, van Miegroet F, Jacquemart AL, Kinet JM. 2004. Inflorescence structure and control of flowering time and duration by light in buckwheat (Fagopyrum esculentum Moench). J Exp Bot. 55:1509–1517. doi: 10.1093/jxb/erh164
- Slawinska J, Obendorf RL. 2001. Buckwheat seed set in planta and durlimiting seed set in buckwheat.ing in vitro inflorescence culture: evaluation of temperature and water deficit stress. Seed Sci Res. 11:223–233.
- Steel RGD, Torrie JH, Dickey DA. 1997. Principles and procedure of statistics: a biometrical approach. New York, NY: McGraw-Hill.
- Tahir I, Farouk S. 1988. Review article on buckwheat. Fagopyrum. 8:33–53.
- Taylor DP, Obendorf RL. 2001. Quantitative assessment of some factors limiting seed set in buckwheat. Crop Sci. 41:1792–1799. doi: 10.2135/cropsci2001.1792