ABSTRACT
Water shortage is a serious environmental and agricultural problem and saline underground water has been widely used to make up the fresh water shortage in northwestern China. An open-field experiment was conducted to establish a proper irrigation scheme with saline water for cherry tomato in the Minqin oasis, where very severe salinization occurs. The experiment had four treatments including fresh or saline irrigation over the crop season (control, C, T3), fresh/saline-water irrigation change on days after thinning 50 (DAT 50, T1) and saline/fresh irrigation change on DAT 50 (T2). Leaf area index (LAI), photosynthesis rate (Pn), transpiration rate (Tr), leaf dry matter (LDM), stem dry matter (StDM), yield, marketable fruit and total soluble solids (TSS) of tomato were measured. Saline irrigation, irrespective of the timing, significantly decreased maximum LAI, LDM and StDM, Pn, Tr and stomatal conductance but significantly stimulated water use efficiency. The reduction in maximum LAI, LDM and StDM was lower in T2 than in T1 and T3. Harvest index (HI) and TSS were higher in T2 and T3 than in T1 and C. Marketable fruit had no significant change in T2 but significantly declined in T1 and T3. Maximum saturated soil conductivity without yield reduction (the salt tolerance threshold) was 3.69 dS m−1. Total yield of tomato would decrease by 9.85% with one unit increase of soil salinityhigher than the threshold. Final yield significantly reduced by 24.6% and 23.1% in T1 and T3 treatments, respectively. Our results suggest that irrigation with saline water before DAT 50 and fresh water after DAT 50 should be advocated for cherry tomato plantation in water-scarce areas like the Minqin oasis.
Introduction
Ground water shortage is a severe environmental and agricultural problem in arid regions worldwide (Bennett Citation2000). The increasing demand for irrigation water to ensure food security for booming population in arid land necessitates the use of saline water in agricultural activities (Malash et al. Citation2008). Saline irrigation-induced higher osmotic pressure generally results in the decrease in growth rate, yield, dry matter (Ismail & Burrage Citation1994), net CO2 assimilation rate, transpiration and stomatal conductance (Romero-Aranda et al. Citation2001), but led to fruit quality improvement (del Amor et al. Citation2001; Huang et al., Citation2012; Maggio et al. Citation2004) and fruit preservability (Tedeschi et al. Citation2011).
Cherry tomato (Lycopersiconesculentum), a variety of tomato that has been cultivated since at least the early 1800s, is planted in China, United States as well as Southern Europe as a cash crop to raise famers' revenue (Mori et al. Citation2008). Plantation of cherry tomato started from1996 and boomed in 2005 in the Minqin oasis. In addition to the low, irregular annual rainfall (110.7 mm) and very high potential evaporation (2593 mm, Xue et al. Citation2015), establishment of a reservoir in the upper part of the oasis in 1950s–1960s and misuse of ground water had led to the disappearance of lake and severe water shortage in the downstream part of the oasis (Feng et al. Citation2005). Thus, underground water use is indispensable to maintain agricultural activities and to ensure the subsistence of local farmers. However, long-term overuse of underground water has resulted in serious environmental and ecological problems, such as intense mineralization of water quality, lowering underground water table and serious salinization (Figure S1). For example, salt concentration of underground water increases from 0.8 g L−1 in the upper part to 5 g L−1 in the downstream part of the oasis (Feng et al. Citation2005). In local agricultural practice, fresh water is used to leach the soil in the beginning of growing season and fresh water and saline groundwater are alternatively used in the remaining period of the growing season in the oasis (You et al. Citation2011). Several studies found the timing of saline irrigation plays an important role in partially alleviating the negative effect of saline irrigation on production and keeping the positive effect on product quality (Heenan et al. Citation1988; Mass & Poss Citation1989; del Amor et al. Citation2001; Läuchli & Grattan Citation2007; Moradi & Ismail Citation2007; Wan et al. Citation2007; Bao & Li Citation2010). Salt tolerance of tomato plants increased when the application of saline irrigation was delayed with 4, 6 and 8 dS m−1 saline irrigation (del Amor et al. Citation2001), and reproductive growth stage is less sensitive than vegetative growth stage (Läuchli & Grattan Citation2007). Flowering and first fruit cluster bearing period was considered as the most sensitive stage for tomato yield (Bao & Li Citation2010). However, saline irrigation (1.4–4.9 dS m−1) had little effect on tomato yield when saline water used from 30 days after transplanting (Wan et al. Citation2007). We therefore hypothesized a low yield reduction but improvement in fruit quality when saline irrigation (7.03 dS m−1) applied in the reproductive growth stage in the oasis.
Materials and methods
Location and environmental background
The experiment was conducted at the Gansu Desert Control Station (38o35′N, 103o03′E), located in the upper part of the Minqin oasis in 2010. Sand and silt account for 90% of the soil, bulk density of soil in the study site was 1.55 g cm−3and pH was 8.3 in the 0–60 cm layer (Huang et al. Citation2011, Citation2012). Mean annual temperature is 7.6°C and mean rainfall was 110.7 mm (1990–2010 average). Sowing generally started in April and harvesting ended in October in the oasis. Rainfall in the crop season was about 90% of the annual amount. Annual average reference evapotranspiration (ETc) was 995 mm (1998–2008) based on the Penman-Monteith method with a crop reference coefficient of 0.408.
Irrigation practices
Flood irrigation with fresh water (ECw = 0.7 dS m−1) was conducted in spring 2010 before laying the polyethylene filmmulch and drip irrigation facilities. Tomato cultivar zhongzhiNO.1, a determinate type, was widely used by local famers as a cash crop. The seeds from a local seed store were cultivated in a greenhouse in May 2010. Seedlings were transplanted to the field when they were about 15 cm in height in a double row bed. The distance between twin rows was 0.5 m and the distance across the double row beds was 0.7 m. In each row, holes about 9 cm in diameter and 15 cm in depth, spaced 0.4 m were drilled and leached with 300 ml fresh water one day before transplantation. Two seedlings were placed to each hole on 5 June 2010, and were thinned to 4.16 plants per m2 land area on 22 June 2010. The row beds and part of the ridge was covered by low-densitywhite polyethylene film. Experimental area was treated with drip irrigation to facilitate the distribution of the saline solution. Drippers at 3 L h−1 was laid at a high density per m2 (0.4 × 0.5 m) in the twin rows. This design ensured a supply of 15 L h−1 m−2 and completely wet of the soil surface. Water amount for each irrigation was adjusted according to the daily ETc by water meters.
The first irrigation was conducted on 6 June 2010 (0 day after thinning, DAT). The crop phenology of cherry tomato and irrigation arrangement was shown in .
Table 1. Phenology and irrigation calendar for the cherry tomato.
Experimental design
A random experimental of four treatments with three replicates in each treatment was used in this study. The four treatments included fresh (C) or saline irrigation (T3) all over the crop season, fresh/saline water changing on DAT 50 (T1) and saline/fresh water changing on DAT 50 (T2). Salt concentration of the saline water was 5 gL−1 to reproduce the salt concentration of groundwater in the oasis, which was made up from chemical compounds and fresh water (). Each plot was 9 m × 6 m and there were 225 plants in each plot.
Table 2. Chemical composition of fresh and saline irrigation water for the experiment.
Sample collection and measurement protocol
Soil and plant samples were collected in the central part of each plot (inner 3 m × 2 m area). Soil samples were taken on DAT 1, 8, 26, 41, 55, 70, 87 and 102 at the depth of 0–0.2 m and 0.2–0.4 m. One sample was collected in each plot on every sampling day. Soil salinity of the saturated paste (ECe) was determined using the method described by Rhoades (Citation1996). ECe of 20 randomly selected soil samples for each layer were determined using EC1:2.5 and a linear regression proposed by Huang et al. (Citation2011). Soil chemical characteristics in the study site could be seen in Huang et al. (Citation2012).
Leaf area, fresh weight of leaves and stems of three plants in each plot were measured on DAT 6, 20, 34, 47, 59, 74 and 88. Leaf area was monitored using an area meter (LI-3100C, Li-COR, Lincoln, NE, USA). The fresh leaves and stems were oven-dried at 75oC for 72 h to obtain the leaf and stem dry matter (LDM and StDM). The data collected to determine the leaf area duration (LAD) was in agreement with Hunt (Citation1982). Leaf weight (LWR) and stem weight ratio (StWR) was defined as the ratio of LDM and StDM to whole plant dry matter. Fruits were harvested on DAT 39, 57, 74, 93 at marketable maturity. The final yield was the sum of the four harvests.
Nine randomly selected fruits in each plot were cut into slices and flesh total soluble solids (TSS) were determined as the oBrix index using a portable refractometer. Average TSS of the nine fruits was used for statistical analysis.
Net photosynthesis rate (Pn), stomatal conductance (gs) and transpiration rate (Tr) were measured at 11 am on DAT 25 and DAT 53 by using Li-Cor 6400 × t (LI-COR, Lincoln, NE, USA). Daily water flux by Tr was calculated by multiplying Tr, time and leaf area. The fifth leaves from the top-down were used for the measurement. The measurement was conducted on three plants in each plot and was repeated for three times for each seedling. Water use efficiency (WUE) was the ratio of Pn to Tr (Fischer & Turner Citation1978).
Statistical analysis
One-way ANOVA was used to evaluate the effect of treatments on soil salinity on each sampling day. Two-way ANOVA was employed to test the interaction effect of treatment and sampling time on soil salinity at the depth of 0–0.2 m and 0.2–0.4 m, Pn, gs, Tr, WUE, LAD and yield. Tukey analysis was used to do the multiple-comparison.
Results
Soil salinity
During the crop season, average ECe in 0–0.4 m was generally higher in T3 and T2 than in T1 and C ((a)). ECe showed different seasonal variation among treatments. Fresh irrigation tended to decrease ECe (lower ECe in C and T1 from DAT 0 to DAT 40, and in C and T2 from DAT 50 to DAT 88) and saline irrigation increased ECe (higher ECe in T2 and T3 from DAT 0 to DAT 40, and in T1 and T3 from DAT 50 to DAT 88, (a)). ECe increased from DAT 40 to DAT 50 in all treatments ((a)). Average ECe in 0–0.4 m significantly increased by 1.93 and 2.91 dS m−1 in the end of growing season (DAT 102) in T1 and T3 treatments, respectively ((b)). Vertical distribution of ECe was different among treatments, with higher ECe in 0–0.2 m in T2 but lower ECe in 0–0.2 m in C and T1 ((c)).
Figure 1. Seasonal variation in soil salinity at the depth of 0–0.4 m ((a), electrical conductivity in saturated paste, ECe, dS m−1), changes in ECe between the beginning of crop season and the end of harvest at 0–0.4 m depth (b), seasonal average ECe at the 0–0.2 and 0.2–0.4 m depth (c) in fresh water irrigation (C), saline water irrigation after DAT 50 (T1), saline water irrigation before DAT 50 (T2) and saline water irrigation (T3). Values followed by the different small letters indicate the significant difference of ECe at p < .05. DAT means days after thinning.
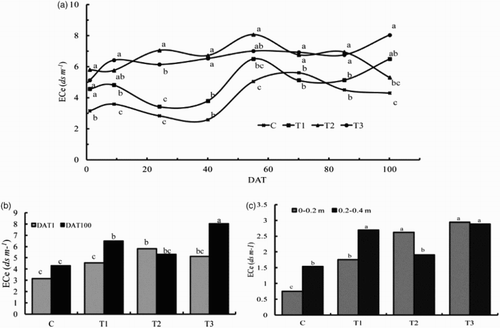
Net photosynthesis, stomatal conductance, transpiration and WUE
Interaction between treatments and measuring time had no significant effect on photosynthesis (Pn), stomatal conductance (gs), transpiration (Tr) and WUE. Pn, gs and Tr were higher on DAT 25 than on DAT 53 but WUE was lower on DAT 25 than DAT 53 in all treatments (). Daily Tr was 6.4 mm in C on DAT 25 and decreased to 3.1 mm on DAT 50, and had no significant difference among treatments on both measuring dates. Saline irrigation decreased Pn by about 11% in T2 and T3 on DAT 25 and the negative effect of saline irrigation on Pn in T2 sustained on DAT 53 ((a)). Effects of saline irrigation on gs and Tr were similar to Pn on DAT 25 but reduced gs on DAT 53 ((b) and (c)). WUE was significantly increased by 17% in T3 on DAT 25 ((d)).
Figure 2. Effects of measuring timing and treatments on net photosynthesis (a), stomatal conductance (b), transpiration rate (c) and water use efficiency (WUE, d) in C, T1, T2 and T3 treatments. Bar values indicated by different small letters are significantly different at the p < .05. For abbreviations for treatments see . DAT means days after thinning.
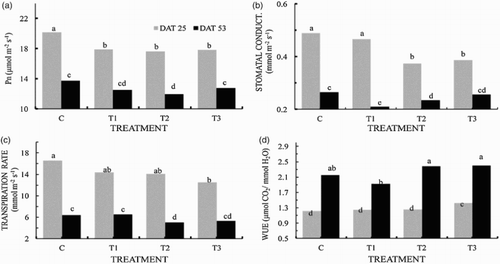
Plant growth
The maximum LAI, LDM and StDM were observed on DAT 59. Maximum LAI, LDM and StDM significantly decreased regardless the timing of saline irrigation applied () and the reduction of LAI, LDM and StDM in T1, T2 and T3 had no significant difference (). LWR and StWR declined in T2 and T3 on DAT 59 (). LAD significantly reduced in T2 and T3 treatments from DAT 40 to the last measurement ().
Figure 3. Interaction (treatments × timing) effects on accumulated leaf area duration (LAD, days) in C, T1, T2 and T3 treatments. Values indicated with different letters are significantly different at p < .05. For abbreviations for treatments see . DAT means days after thinning.
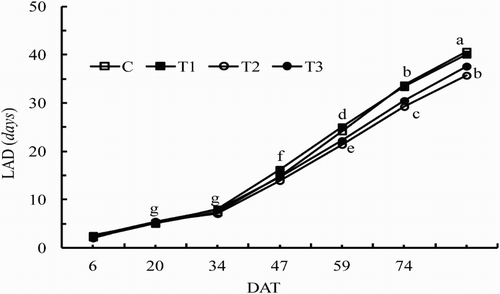
Table 3. Effects of different irrigation treatments on growth, yield and fruit quality parameters.
Yield and product quality
Saline irrigation significantly decreased the yield of marketable fruits by 24.6% and 23.1% in T1 and T3, respectively, compared to the C treatment but had no effect in T2 (). Harvests after DAT 50 accounted for 88–95% of the total yield. Saline irrigation had no significant effect on yield in the first harvest (before DAT 50 in T2 and T3 treatments) but significantly declined yield after DAT 50 in T1 and T3 treatments (). Saline irrigation significantly increased the harvest index (HI) and TSS in T2 and T3 treatments but had no significant effect in T1 ().
Salt tolerance
Yield response to salinity was evaluated as a linear model with a threshold () according to the linear Mass–Hoffman model (1977).
Figure 5. Relative yield responses to average soil salinity (0–0.4 m). The areas indicated as S, MS, MT and T correspond to sensitive, moderately sensitive, moderately tolerant and tolerant according to the Mass–Hoffman model.
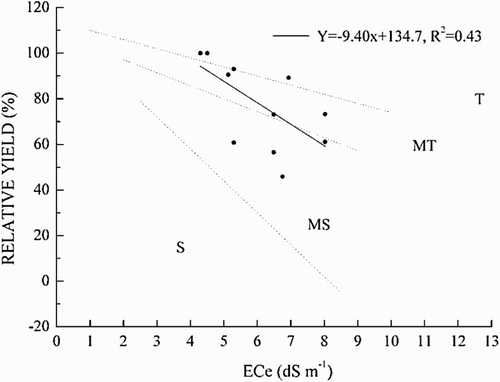
where Y is the relative yield (%); b is the slope (yield reduction per unit increase in salinity); ECe is the average (0–0.4 m) of soil electrical conductivity of soil sample and a is the salinity threshold. The relationship expresses how the plant growth rate reduces linearly as soil salinity increases above a threshold a.
The relative yield was calculated by dividing the yield in T1–T3 treatments by the yield of C in each harvest. Saline irrigation had no significant effect on the first harvest; therefore, we used nine pairs of relative yield and soil salinity to do the salt-tolerance analysis.
The ECe threshold beyond which the crop yield decreased was 3.69 dS m−1. Beyond the threshold the yield decreased at the rate of 9.85% per unit increase in ECe. Yield reduction rate would rate the cultivar as moderately sensitive to salinity ().
Discussion
The seasonal variation in soil salinity was the result of soil water balance between irrigation and evapotranspiration, and the associated movement of soil salt with soil water. Higher daily Tr than the sum of irrigation and rainfall from DAT 40 to DAT 50 could induce the upward movement of soil water and soil salt. Lower ECe from DAT 0 to DAT 40 in C and T1 and from DAT 50 to DAT 88 in C and T2 ((a)) could be induced by leaching of salt to deep soil by fresh irrigation because of lower Tr (Huang et al. Citation2012). The leaching effect could be partly proved by the soil water content increase from DAT 0 to DAT 40. The significant increase in soil ECe between DAT 102 and DAT 1 in T1 and T3, but no change in T2 ((b)) indicates the build-up of soil salt primarily occurred in the later period of the growing season. Observed lower soil salinity in 0–0.2 m than in 0.2–0.4 m in C and T1 treatments but no difference between the two layers in T3 ((c)) was a different result from a furrow saline irrigation study in which soil salt aggregated in surface layer (Kaman et al. Citation2006). Suppression on soil evaporation and consequent depressed salt accumulation toward the top layer due to plastic mulch (Brent et al. Citation1991; Li et al. Citation2004) could provide support for a different result in soil salt between the current study and the furrow saline irrigation study.
Several studies showed that soil salinity slows growth, decreases yield and plant photosynthesis and transpiration, but compensates the yield loss by promoting fruit quality through increase in TSS (Ismail and Burrage Citation1994; Schwarz and Klaring Citation2002; Botia et al. Citation2005; Bao and Li Citation2010; Zong et al. Citation2011). Decreased maximum LAI, maximum LDM, StDM and accumulated LAD () in T1–T3 supported the effect of saline irrigation on plant growth, and indicated the sensitiveness of tomato plant growth to salt stress irrespective of the timing saline irrigation used. Higher relative decrease in maximum LAI, maximum LDM and StDM in T1 and T3 than in T2 treatment confirmed the finding that salt sensitivity at reproductive growth stage is greater than at vegetative growth stage (Läuchli Citation1990; Läuchli & Grattan Citation2007). Reduction in Pn () but no change in accumulated LAD () on DAT 25 suggests leaf morphology change through increasing leaf thickness as evidenced in kochia (Masoume et al. Citation2009). Decrease in LDM and StDM after DAT 50 in T2 indicates the persistence of saline irrigation effects on plant growth even though fresh water irrigation has been used.
Tomato yield is quite sensitive to salinity increase above 3.0 dS m−1 (Cuartero & Fernandez-Munoz Citation1998; del Amor et al. Citation2001; Malash et al. Citation2012). Significant decrease of final marketable yield in T1 and T3 but no significant change () suggests the stage-dependent sensitivity of yield to saline irrigation. The highest yield loss of tomato was observed when plants were irrigated with saline water at the flowering and fruit-bearing stage (Bao & Li Citation2010). Yield loss after DAT 50 contributed 88–94% to the total reduction, which seems different with the results that flowering and fruit bearing stage was the most sensitive stage (Bao & Li Citation2010). In their study, flowering and fruit-bearing stage was 40 days after topping and greenhouse could advance plant phenology. Therefore, the flowering and fruit-bearing stage could be equivalent to DAT 50 in this study. The significant decline in T1 and T3 but not in T2 treatments suggests that saline-water irrigation at peak-fruit-development stage has great influence on yield. Our determined threshold value of 3.69 dS m−1and the corresponding yield decrease rate of 9.85% per unit increase of ECe was not in agreement with the one reported by Mass and Hoffman (Citation1977). Cuartero and Fernandez-Munoz (Citation1998) and Mass (Citation1986) found a threshold value of about 3.0 dS m−1 and yield decrease rate of about 9–10% per unit of ECe increase. The higher threshold value of this experiment is due to the inclusion of the relative yield of 100 (Katerji et al. Citation2003). The less sensitivity to salinity in the experiment () could be due to the mixed use of fresh and saline water, as shown by the ECe variation in the crop season.
Cherry tomato yield was highly correlated with LAD (R2 = .614, n = 16, p < .01). The corresponding higher reduction in LDM and yield in T1 and T3 treatments () confirmed the usefulness of LAD as an indicator of crop yield (Huang et al. Citation2012). Though the fruit yield reduced in T2 and T3 treatments, HI increased (, +24% in T2 and T3 treatments) because LDM and StDM reduction was smaller than yield decrease (). HI has no significant change in T1 since marketable fruit decrease (−24.6% T1 vs. C) could be offset by decrease in LDM and StDM (leaf, −28.0% and stem, −23.4% T1 vs. C). The increase in TSS with saline irrigation is widely documented, which could partly compensate yield loss (Bustan et al. Citation2005; Magan et al. Citation2008; Malash et al. Citation2008; Tedeschi et al. Citation2011; Zong et al. Citation2011). Irrigation with saline water before DAT 50 and fresh water after DAT 50 should be advocated for cherry tomato plantation in water-scarce areas like the Minqin oasis.
Acknowledgements
The authors would like to thank the anonymous reviewers and editors for their help and suggestions.
Disclosure statement
No potential conflict of interest was reported by the authors.
Additional information
Funding
References
- del Amor FM, Martinez V, Cerda A. 2001. Salt tolerance of tomato plants at affected by stage of plant development. Hort Sci. 36:1260–1263.
- Bao H, Li YX. 2010. Effect of stage-specific saline irrigation on greenhouse tomato production. Irrig Sci. 28:421–430. doi: 10.1007/s00271-009-0204-x
- Bennett AJ. 2000. Environmental consequences of increasing production: some current perspectives. Agric Ecosyst Environ. 82:89–95. doi: 10.1016/S0167-8809(00)00218-8
- Botia P, Navarro JM, Cerda A, Martinez V. 2005. Yield and fruit quality of two melon cultivars in irrigated with saline water at different stages of development. Eur J Agron. 23:243–253. doi: 10.1016/j.eja.2004.11.003
- Brent C, Sauer T, Scotter D. 1991. Redistribution of water and solute following infiltration from a surface drip source. Water Resour Res. 27:2091–2097. doi: 10.1029/91WR00938
- Bustan A, Cohen S, De Malach Y, Zimmermann P, Golan R, Sagi M, Pasternak D. 2005. Effects of timing and duration of brackish irrigation water on fruit yield and quality of late summer melons. Agric Water Manage. 74:123–134. doi: 10.1016/j.agwat.2004.11.009
- Cuartero J, Fernandez-Munoz R. 1998. Tomato and salinity. Sci Hortic. 78:83–125. doi: 10.1016/S0304-4238(98)00191-5
- Feng Q, Wei L, Su YZ, Zhang YW, Si JH. 2005. Distribution and evolution of water chemistry in Heihe River Basin. Environ Geol. 45:947–956. doi: 10.1007/s00254-003-0950-7
- Fischer RA, Turner NC. 1978. Plant productivity in the arid and semiarid zones. Annu Rev Plant physiol. 29:277–317. doi: 10.1146/annurev.pp.29.060178.001425
- Heenan DP, Lewin LG, McCaffery DW. 1988. Salinity tolerance in rice varieties at different growth stages. Aust J Exp Agric. 28:343–349. doi: 10.1071/EA9880343
- Huang CH, Xue X, Wang T, De Mascellis R, Mele G, You QG, Peng F, Tedeschi A. 2011. Effects of saline irrigation on soil properties in northwest China. Environ Earth Sci. 63:701–708. doi: 10.1007/s12665-010-0738-5
- Huang CH, Zong L, Buonanno M, Xue X, Wang T, Tedeschi A. 2012. Impact of saline water irrigation on yield and quality of melon (Cucumis melo cv. Huanghemi) in northwest China. Eur J Agron. 43:68–76. doi: 10.1016/j.eja.2012.05.008
- Hunt R. 1982. Plant growth curves: an introduction to the functional approach to plant growth analysis. London: Edward Arnold Press.
- Ismail MR, Burrage SW. 1994. Effects of salinity on growth, water relations and photosynthetic rate of tomatoes grown in nutrient film technique. Pertanika J Trop Agric Sci. 17:73–79.
- Kaman H, Kirda C, Cetin M, Topcu S. 2006. Salt accumulation in the root zones of tomato and cotton irrigated with partial root-drying technique. Irrig Drain. 55:533–544. doi: 10.1002/ird.276
- Katerji N, van Hoorn JW, Hamdy A, Mastrorilli M. 2003. Salinity effect on crop development and yield, analysis of salt tolerance according to several classification methods. Agric Water Manage. 62:37–66. doi: 10.1016/S0378-3774(03)00005-2
- Läuchli A. 1990. Calcium, salinity and the plasma membrane. In: Leonard RT and Hepler PK, editors. Calcium in plant growth and development. Rockville, MD: American Society for Plant Physiologists. p. 26–35.
- Läuchli A, Grattan SR. 2007. Plant growth and development under salinity stress. In: Jenks MA, Hasegawa PM, Jain SM, editors. Advances in molecular breeding toward drought and salt tolerant crops. Netherlands: Springer Press. p. 1–29.
- Li Y, Wang WY, Wang JQ, Shao MA. 2004. Experimental study on soil water movement and salt transport characteristics of evaporation from film holes in perforated plastic mulch after trickle infiltration. Chin J Soil Sci. 35:435–440.
- Magan JJ, Gallardo M, Thompson RB, Lorenzo P. 2008. Effects of salinity on fruit yield and quality of tomato grown in soil-less culture in greenhouses in Mediterranean climatic conditions. Agric Water Manage. 95:1041–1055. doi: 10.1016/j.agwat.2008.03.011
- Maggio A, De Pascale S, Angelino G, Ruggiero C, Barbieri B. 2004. Physiological response of tomato to saline irrigation in long-term salinized soils. Eur J Agron. 21:149–159. doi: 10.1016/S1161-0301(03)00092-3
- Malash NM, Ali FA, Fatahalla MA, Tawfic S. 2012. Response of tomato to irrigation with saline water applied by different irrigation methods and water management strategies. Int J Plant Prod. 2:101–116.
- Malash NM, Flowers TJ, Ragab R. 2008. Effect of irrigation methods management and salinity of irrigation water on tomato yield, soil moisture and salinity distribution. Irrig Sci. 26:313–323. doi: 10.1007/s00271-007-0095-7
- Masoume S, Mohammad K, Alireza K. 2009. Growth analysis of kochia (Kochia scoparria (L.) schrad) irrigated with saline water summer cropping. Pak J Bot. 41:1861–1870.
- Maas EV. 1986. Salt tolerance of plants. Appl Agric Res. 1:12–26.
- Mass EV, Hoffman GJ. 1977. Crop salt tolerance: current assessment. J Irrig Drain E-ASCE.103:115–134.
- Mass EV, Poss JA. 1989. Salt sensitivity of wheat at various growth stages. Irrig Sci. 10:29–40.
- Moradi F, Ismail MA. 2007. Responses of photosynthesis, chlorophyll fluorescence and ros-scaverning systems to salt stress during seedling and reproductive stages in rice. Ann Bo. 99:1167–1173.
- Mori M, Amato M, Mola ID, Caputo R, Quaglietta Chiaranda F, Tommaso TD. 2008. Productive behaviour of “cherry”-type tomato irrigated with saline water in relation to nitrogen fertilisation. Eur J Agrono. 29:135–143. doi: 10.1016/j.eja.2008.05.001
- Rhoades JD. 1996. Salinity: electrical conductivity and total dissolved solids. In: Spark DL, editor. Methods of soil analysis. Part 3. Chemical methods. Madison, USA: SSSA book series. p. 417–435.
- Romero-Aranda R, Soria T, Cuartero J. 2001. Tomato plant-water uptake and plant–water relationships under saline growth conditions. Plant Sci. 160:265–272. doi: 10.1016/S0168-9452(00)00388-5
- Schwarz D, Klaring HP. 2002. Growth and photosynthetic response of tomato to nutrient solution concentration at two light levels. J Am Soc Hort Sci. 127:984–900.
- Tedeschi A, Lavini A, Riccardi M, Pulvento C, d'Andria R. 2011. Melon crops (Cucumis melo L.,cv. Tendral) grown in a Mediterranean environment under saline-sodic conditions: part I. Yield quality. Agric Water Manage. 98:1392–1338.
- Wan SQ, Kang YH, Wang D, Liu SP, Feng LP. 2007. Effect of drip irrigation with saline water on tomato (Lycopersicon esculentum Mill) yield and water use in semi-humid area. Agric Water Manage. 90:63–74. doi: 10.1016/j.agwat.2007.02.011
- Xue X, Liao J, Hsing YT, Huang CH, Liu FM. 2015. Policies, land use and water resource management in an arid oasis ecosystem. Environ Manage. 55:1036–1051. doi: 10.1007/s00267-015-0451-y
- You QG, Xue X, Huang CH. 2011. Preliminary study on the effects of saline irrigation on soil salinization in deep groundwater area: a case study of Minqin oasis. J Desert Res. 31:302–308 (in Chinese with English Abstract).
- Zong L, Tedeschi A, Xue X, Wang T, Menenti M, Huang CH. 2011. Effect of different irrigation water salinities on some yield and quality components of tow field-grown Cucurbit species. Turk J Agric For. 35:297–307.