ABSTRACT
Pyrenochaeta lycopersici is the causal agent of corky root, an important soilborne disease of tomato (Lycopersicon esculentum) and other solanaceous crops. P. lycopersici isolates are classified into Types 1 and 2 on the basis of several physiological and molecular features. In this study, we aimed to establish a loop-mediated isothermal amplification (LAMP) method to identify Types 1 and 2 isolates. Using specific LAMP primer sets for Types 1 and 2, both types were easily identified within 35 min. The LAMP method demonstrated equal sensitivity to the polymerase chain reaction in molecular identification of the pathogen in cultured mycelia, infested plant roots, and their surrounding soil. The LAMP technology will contribute to the detection and identification of the pathogen, crop protection, and thorough understanding about the ecology of corky root disease of tomato.
Introduction
Pyrenochaeta lycopersici is the causal agent of corky root, an important soilborne disease of tomato (Lycopersicon esculentum) and other cultivated crops (Grove & Campbell, Citation1987). The causal agent of corky root was known as the gray sterile fungus until it sporulated and was identified as P. lycopersici (Termohlen Citation1962; Schneider & Gerlach Citation1966). The following symptoms can be observed in infected plants: decrease in size, such as atrophy, chlorosis on the underside of leaves, and characteristic corky roots. The observation of the rapid brown rot caused by the culture filtrates of P. lycopersici suggested that the pathogen secretes phytotoxic substances during its growth (Fiume & Fiume Citation2003). Generally, phytopathogenic microorganisms produce a variety of pectic enzymes capable of attacking plant cell components (Collmer & Keen Citation1986). In the case of P. lycopersici, pectic enzymes are synthesized when the fungus recognizes host and non-host (or tolerant host) cell walls, and the enzymes support the growth of the pathogen; however, the variety of the enzymes produced and supporting growth does not correlate with host susceptibility or tolerance (Collmer & Keen Citation1986). The pathogenesis of corky root rot is affected by small differences in soluble sugar concentrations of susceptible and tolerant tomato cultivars (Collmer & Keen Citation1986).
The common methods for the control of corky root disease are based on soil solarization and grafting on disease-resistant rootstocks. However, the most effective control becomes useless at a high density of the pathogen in the field. Therefore, an accurate diagnosis based on high-throughput and sensitive detection is very important for the success of crop protection.
P. lycopersici isolates have been classified into Types 1 and 2 on the basis of the variations in several physiological and molecular features detected by the mycelial growth rate, ribosomal DNA inter-transcribed spacer (rDNA-ITS) region sequence, and random amplified polymorphic DNA (RAPD) and amplified fragment length polymorphism (AFLP) analyses (Sugiura et al. Citation2003; Infantino & Pucci Citation2005; Pucci et al. Citation2011). The homology of the rDNA-ITS sequences between these two groups is 89–90%, while there is 99–100% similarity within each type (Infantino & Pucci Citation2005).
In a previous study, the variability in pathogenicity among Type 2 strains (ER-854, ER-876, ER-925, and ER-955) was shown to be higher than that between Type 1 (ER-958) and Type 2 (ER-955) strains (Infantino et al. Citation2003). However, there were significant variations in the genetic structure between these two types that were sampled from eight locations in Italy and Israel (Infantino et al. Citation2015). Considering this varied environmental adaptation between these two types, specific detection and diagnosis are required for accurate disease forecasting in each field.
Specific polymerase chain reaction (PCR) primer sets have been designed for the differential diagnosis of each type. These two types of P. lycopersici were identified in Japan, Italy, Israel, and Turkey (Sugiura et al. Citation2003; Infantino & Pucci Citation2005; Bayraktar & Oksal Citation2011; Infantino et al. Citation2015). PCR is widely used to detect plant pathogens in infected plants. Recently, a loop-mediated isothermal amplification (LAMP) method has been established (Notomi et al. Citation2000; Mori et al. Citation2001; Nagamine et al. Citation2002; Mori & Notomi Citation2009), which allows a rapid diagnosis of various plant pathogens in the field (Fukuta et al. Citation2003; Tomlinson et al. Citation2007; Niessen & Vogel Citation2010; Fukuta et al. Citation2013). LAMP has some advantages over PCR, for instance, no requirement of a thermal cycler, data analysis without gel electrophoresis, fast amplification within 1 h, and high tolerance to inhibitors from biological sources (Kaneko et al. Citation2007). In this study, we designed LAMP primer sets for specific and rapid identification of P. lycopersici Types 1 and 2 and compared the detection efficiency between LAMP and PCR methods.
Materials and methods
Isolates and DNA extraction from mycelia
The isolates of P. lycopersici used in this study are listed in . The isolates were grown on half-strength Czapek Dox (Becton, Dickinson and Company, Tokyo, Japan) agar plates for 2 weeks at 25°C. Three mycelial disks cut from the growing margin of a colony were transferred to a 9 cm Petri dish containing 15 mL of half-strength Czapek Dox broth and grown at 25°C for 2 weeks. Fresh mycelia were collected by filtration and ground well in a mortar with liquid nitrogen. Total DNA was extracted using the DNeasy Plant Mini kit (Qiagen K.K., Tokyo, Japan) following the manufacturer's protocol. All samples were stored at −20°C until used.
Table 1. Pyrenochaeta isolates used in this study and results of detection using LAMP and PCR methods.
LAMP primer design and reaction
The LAMP reaction requires a set of four (F3, B3, FIP, and BIP) or six (additional F-loop and B-loop) primers, each recognizing two regions on the template DNA (Notomi et al. Citation2000; Mori et al. Citation2001; Nagamine et al. Citation2002; Mori & Notomi Citation2009). The primer FIP consisted of F1c (the complementary sequence of F1) and F2, and the primer BIP consisted of B1c (the complementary sequence of B1) and B2. The outer primers were F3 and B3, which together functioned as the starter of the LAMP reaction. The type-specific LAMP primer sets were designed based on the rDNA-ITS regions of Type 1 isolate MAFF712039 and Type 2 isolate MAFF712040 (). The sets of LAMP primers were designed using the Primer Explorer V4 software (http://primerexplorer.jp) and further manually redesigned to provide more specificity.
Table 2. Sequences of the LAMP primers used in this study.
The LAMP amplification was conducted in a total volume of 25 μL of a reaction mixture containing 2.5 μL of 1 × LAMP reaction buffer (20 mM Tris–HCl, pH 8.8, 10 mM KCl, 1% Tween 20), 8 mM MgSO4, 10 mM (NH4)2SO4, 0.8 M betaine (Sigma-Aldrich, Tokyo, Japan), 1.4 mM dNTPs (GE Healthcare, Tokyo, Japan), 1.6 μM each of FIP and BIP primer, 0.2 μM each of F3 and B3 primer, 0.8 μM each of F-loop and B-loop primer, 8 U of Bst DNA polymerase (New England Biolabs, Tokyo, Japan), and from 1 pg to 10 ng of extracted DNA. The reaction mix without DNA template was used as a negative control. The reaction temperature was 63°C. The reactions were run using an RT-160C Loopamp real-time turbidimeter (Eiken Chemical Co., Ltd., Tokyo, Japan). The type-specific detection of each isolate ( and ) was performed at least twice with each DNA sample. Detection from a diluted DNA template () was performed three times with each DNA sample. Type-specific detection using DNA extracted from roots and soil () was performed twice with each DNA sample.
Figure 1. Specific detection of Type 1 and Type 2 isolates. Amplification plots of LAMP reactions using the primer sets for P. lycopersici (a) Type 1 and (b) Type 2 are shown. The DNA amounts were approximately 10 ng per reaction. The Type 1 and Type 2 data are shown as triangles and circles, respectively. The threshold time for specific detection is shown as a broken line.
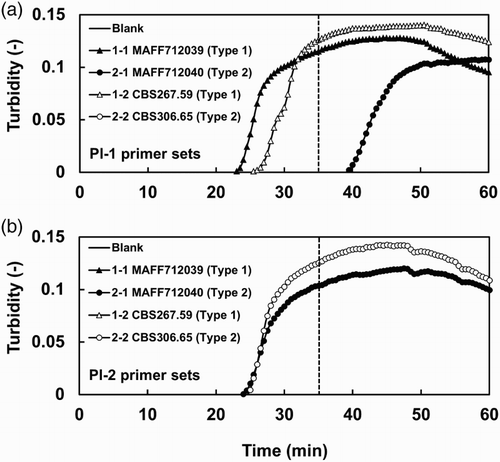
Figure 2. LAMP-based detection with different initial amounts of DNA templates. Representative amplification plots from three replicate experiments are shown. The DNA dilution series were prepared from (a) Type 1 isolate MAFF712039 and (b) Type 2 isolate MAFF712040. The threshold time for specific detection is shown as a broken line.
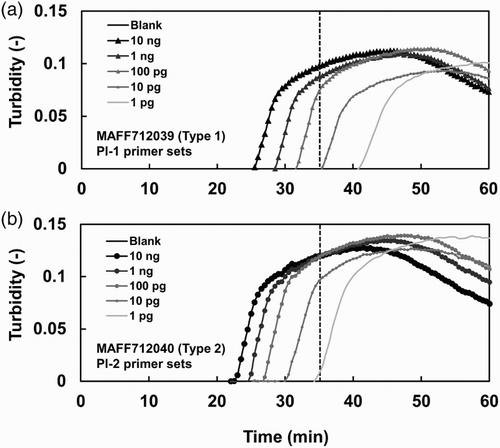
Figure 3. Detection of a Type 2 isolate in infected roots and soil using the LAMP method. Amplification plots of DNA extracted from (a) roots and (b) soil are shown. The amplification plots shown in (a) and (b) were obtained in the same run. The same plots are included in both (a) and (b) for the control samples (Blank, MAFF712039, and MAFF712040). The DNA templates were extracted from roots and their surrounding soil, which were infected with different amounts of BGI inoculum of Type 2 isolate HB-2. The DNA amounts were approximately 10 ng per reaction. Genomic DNA samples extracted from Type 1 isolate MAFF712039 and Type 2 isolate MAFF712040 were used as controls. The threshold time for specific detection is shown as a broken line.
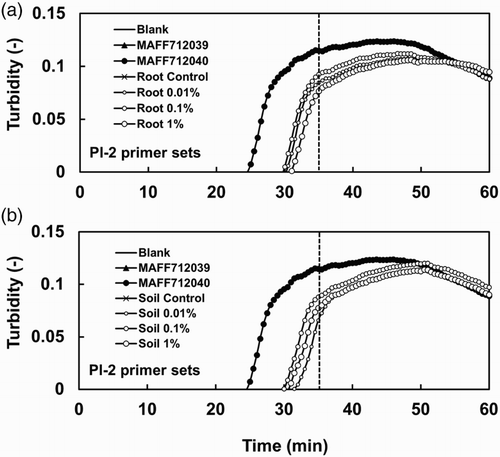
PCR primers and reaction
PCR primers for detection of P. lycopersici isolates were designed by Infantino and Pucci (Citation2005). The primers for Type 1 were Plyc1-F (5′-GTAGGATTGCGTGCTTTGGT-3′) and Plyc1-R (5′-AGTTTTCTGACGCTGATTGC-3′), which produced a 147 bp band. The Type 2 primers were Plyc2-F (5′-CTGTAACATTGGGGGCTGGT-3′) and Plyc2-R (5′-CGATGCCAGAACCAAGAGAT-3′), which produced a 209 bp band. The PCR amplification was carried out in a total volume of 25 μL of a reaction mixture containing 1 × GoTaq® Colorless Master Mix (Promega, Tokyo, Japan), 0.5 μM each primer, and from 100 fg to 10 ng of extracted DNA. For the amplification of soil DNA, bovine serum albumin (New England Biolabs, Tokyo, Japan) was added to a final concentration of 0.16 mg/mL according to the manufacturer's protocol. The PCR thermal cycling conditions were as follows: denaturation for 2 min at 94°C, followed by 35 cycles of 30 s at 94°C, 30 s at 62°C, and 1 min at 72°C, and final extension at 72°C for 7 min. Amplified DNA fragments were analyzed by electrophoresis in a 1.5% agarose gel in Tris–acetate–EDTA buffer (pH 8.0), stained with ethidium bromide, and visualized under ultraviolet light. The PCR experiments using diluted DNA templates and type-specific detection using DNA extracted from roots and soil were performed three times with each DNA sample.
Detection from roots and soil
The detection of P. lycopersici Type 2 in the roots of tomato plants with different disease severity and in their surrounding soil was done using the LAMP primer set for Type 2 (). The samples of tomato plants with different disease severity were prepared as follows: Tomato (L. esculentum cv. Micro-Tom) plants were grown in a cell tray (37 × 37 × 37 mm per cell) filled with a potting medium at 25°C for 23 days under 16/8h (light/dark) conditions. Then, the plants were transferred to a plastic pot (9 cm in diameter) containing soil inoculated with 0.01%, 0.1%, and 1% (w/v) of a ground barley grain inoculum (BGI) of the P. lycopersici HB-2 Type 2 isolate () and grown for 17 days under the same conditions. The disease severity was evaluated based on the root discoloration scale (0–5) as follows: 0 (healthy, no discoloration), 1 (<10% discoloration), 2 (10–25% discoloration), 3 (25–50% discoloration), 4 (50–75% discoloration), and 5 (75–100% discoloration). The roots with different disease severity, 0, 1.0, 4.0, and 4.3 (DSI, disease severity indexes), and their surrounding soil were collected from control (not inoculated) plants and 0.01%, 0.1%, and 1% (w/v) BGI-inoculated plants, respectively. The samples were stored at −20°C until extraction.
DNA was extracted from the roots using the glass microfiber–filter extraction method (Fukuta et al. Citation2010), with a small modification. In brief, Whatman® glass microfiber filters (GF/C, Sigma-Aldrich) were dipped in sodium sulfite buffer (20 mM Tris–HCl, pH 8.0, 2 mM EDTA-Na2, and 10% Na2SO3) and dried. Whole-root samples were ground well and transferred to 1.5 mL Eppendorf tubes. Two hundred microliters of grinding buffer (20 mM Tris–HCl, pH 8.0 and 2 mM EDTA-Na2) was added to the tube and vortexed well. Previously dried Whatman® glass microfiber filters were dipped into the grinding mixture and transferred to new 1.5 mL Eppendorf tubes. Two hundred microliters of fixative buffer (100 mM Tris–HCl, pH 8.0, 10 mM EDTA-Na2, and 5 M guanidine thiocyanate) was added to the tube, which was incubated at room temperature for 5 min and then washed twice with 200 μL of washing buffer (50 mM Tris–HCl, pH 8.0, 5 mM EDTA-Na2, 200 mM NaCl, and 60% ethanol) and once with 70% ethanol. Finally, 100 µL of 1/5 strength TE buffer (2 mM Tris–HCl, pH 8.0, and 0.2 mM EDTA-Na2) was added to the tube. The DNA extracted from roots was stored at −20°C until used.
Total DNA was extracted from 0.5 g of soil using the FastDNA® Spin Kit for Soil (MP Biomedicals, Tokyo, Japan) according to the manufacturer's instructions, with the following modifications. To prevent DNA from binding to soil particles, skim milk was added to the extraction buffer (Kageyama et al. Citation2003). One hundred microliters of a 20% autoclaved skim milk solution (2 mg per tube) was added to the tube, along with 878 µL of sodium phosphate buffer and 122 µL of MT buffer.
The template DNA was amplified using the LAMP and PCR methods described above.
Results
Primer design
For the specific detection of P. lycopersici Types 1 and 2, LAMP primer sets (F3, B3, FIP, BIP, F-loop, and B-loop) were designed for each type (). The FIP primer contained sequence mismatches at the 5′ end in regions important for synthesis of the dumbbell-like starting structure (Parida et al. Citation2008).
Type-specific detection
and show that the amplification of both Types 1 and 2 DNA was consistently detected using the Types 1 and 2 LAMP primer sets, respectively. The threshold time for the reliable detection of each type was 35 min (broken line in ). One of the Type 2 isolates, MAFF712040, was amplified with the Pl-1 primer set after the threshold time (), and this result was confirmed in six out of seven reactions (data not shown). The sensitivity of detection of the Types 1 and 2 isolates with the Types 1 and 2 LAMP primer sets, respectively, was tested using different amounts of DNA of each type. LAMP products were consistently obtained at DNA concentrations higher than 100 pg ().
DNA extracted from 11 Type 1 isolates, 12 Type 2 isolates, and one isolate each of related species, P. globosa and P. gentianicola, was used in LAMP reactions (). The LAMP primer sets for Types 1 and 2 showed specific detection of each type of the P. lycopersici isolates within the threshold time (35 min) but did not detect the isolates of the related species.
Detection from roots and soil
shows LAMP-based detection from the roots and soil, which were infected with the P. lycopersici HB-2 Type 2 isolate (), using the Type 2 LAMP primer set. Both roots and their surrounding soil collected from plants with clear symptoms (disease severities 4.3 and 4.0) and unclear symptoms (disease severity 1.0) showed positive DNA amplification within 35 min. However, no amplification of Type 2 DNA was detected from the roots and soil of the healthy plants (control). Additionally, the Type 1 LAMP primer set could not amplify Type 2 DNA from the roots and soil of the inoculated plants (data not shown).
Discussion
In comparison with PCR, LAMP has many advantages, such as a higher efficiency and tolerance to substances inhibiting DNA amplification (Kaneko et al. Citation2007; Parida et al. Citation2008). In this study, we aimed to establish a LAMP method to identify Types 1 and 2 isolates of P. lycopersici. Two LAMP primer sets able to specifically detect each type were designed based on sequence mismatches in the rDNA-ITS regions of Types 1 and 2 (). The LAMP method using the Types 1 and 2 specific primer sets was able to specifically detect Types 1 and 2 using mycelial DNA of Types 1 and 2, respectively. However, DNA from one of the Type 2 isolates, MAFF712040, was amplified with the Pl-1 primer set after the threshold time. MAFF712040 was cultured many times from a stock and did not contain other microorganisms. The most likely possibility seems to be a cross-reaction produced with the Pl-1 primer set. The cross-reaction was frequently observed in six out of seven reactions (data not shown); however, it was possible to exclude those from consideration because the amplification occurred later than the threshold time. The LAMP products were stably obtained from both Types 1 and 2 DNA samples with concentrations higher than 100 pg (). The detection efficiency of LAMP was equal to that of the PCR method. The LAMP method could easily amplify DNA extracted from infested plant roots and their surrounding soil and also from plants with unclear symptoms (disease severity 1.0). This result indicates that the LAMP method is able to detect the pathogen in plants and soil at an early stage of disease development. The detection efficiency of the LAMP method using the DNA isolated from the infested roots and soil was also equal to that of the PCR method. However, the LAMP method could be used for rapid and high-throughput detection and thus can potentially be used as an alternative to time-consuming diagnostic methods to analyze many samples. Additionally, the LAMP method can be used to easily gather field data, which may contribute to a deep understanding of the ecology of the pathogen, particularly field distribution patterns of Types 1 and 2 strains.
Supplemental figures
Download Zip (2 MB)Acknowledgements
The author would like to acknowledge Dr Shiro Fukuta (Aichi Agricultural Research Center) for technical support.
Disclosure statement
No potential conflict of interest was reported by the authors.
References
- Bayraktar H, Oksal E. 2011. Molecular, physiological and pathogenic variability of Pyrenochaeta lycopersici associated with corky rot disease of tomato plants in Turkey. Phytoparasitica. 39:165–174. doi: 10.1007/s12600-011-0150-z
- Collmer A, Keen NT. 1986. The role of pectic enzymes in plant pathogenesis. Ann Rev Phytopathol. 24:383–409. doi: 10.1146/annurev.py.24.090186.002123
- Fiume F, Fiume G. 2003. Use of culture filtrates of Pyrenochaeta lycopersici in tests for selecting tolerant varieties of tomato. J Plant Pathol. 85:131–133.
- Fukuta S, Kato S, Yoshida K, Mizukami Y, Ishida A, Ueda J, Kanbe M, Ishimoto Y. 2003. Detection of tomato yellow leaf curl virus by loop-mediated isothermal amplification reaction. J Virol Methods. 112:35–40. doi: 10.1016/S0166-0934(03)00187-3
- Fukuta S, Takahashi R, Kuroyanagi S, Miyake N, Nagai H, Suzuki H, Hashizume F, Tsuji T, Taguchi H, Watanabe H, Kageyama K. 2013. Detection of Pythium aphanidermatum in tomato using loop-mediated isothermal amplification (LAMP) with species-specific primers. Eur J Plant Pathol. 136:689–701. doi: 10.1007/s10658-013-0198-3
- Fukuta S, Tsuji T, Ikeda TM, Yoshida T, Fujii K. 2010. Development of loop-mediated isothermal amplification (LAMP) markers for GluB3, GluA3 and GluA1 of hexaploid wheat. Breeding Res. 12:87–95. doi: 10.1270/jsbbr.12.87
- Grove GG, Campbell RN. 1987. Host range and survival in soil of Pyrenochaeta lycopersici. Plant Disease. 71:806–809. doi: 10.1094/PD-71-0806
- Infantino A, Aragona M, Brunetti A, Lahoz E, Oliva A, Porta-Puglia A. 2003. Molecular and physiological characterization of Italian isolates of Pyrenochaeta lycopersici. Mycol Res. 107:707–716. doi: 10.1017/S0953756203007962
- Infantino A, Pucci N. 2005. A PCR-based assay for the detection and identification of Pyrenochaeta lycopersici. Eur J Plant Pathol. 112:337–347. doi: 10.1007/s10658-005-6605-7
- Infantino A, Pucci N, Aragon M, Felice SD, Rau D. 2015. Genetic structure of Italian populations of Pyrenochaeta lycopersici the causal agent of corky root rot of tomato. Plant Pathol. 64:941–950
- Kageyama K, Komatsu T, Suga H. 2003. Refined PCR protocol for detection of plant pathogens in soil. J Gen Plant Pathol. 69:153–160.
- Kaneko H, Kawana T, Fukushima E, Suzutani T. 2007. Tolerance of loop-mediated isothermal amplification to a culture medium and biological substances. J Biochem Bioph Methods. 70:499–501. doi: 10.1016/j.jbbm.2006.08.008
- Mori Y, Nagamine K, Tomita N, Notomi T. 2001. Detection of loop-mediated isothermal amplification reaction by turbidity derived from magnesium pyrophosphate formation. Biochem Bioph Res Co. 289:150–154. doi: 10.1006/bbrc.2001.5921
- Mori Y, Notomi T. 2009. Loop-mediated isothermal amplification (LAMP): a rapid, accurate, and cost-effective diagnostic method for infectious diseases. J Infect Chemother.: Official J Japan Soc Chemother. 15:62–69. doi: 10.1007/s10156-009-0669-9
- Nagamine K, Hase T, Notomi T. 2002. Accelerated reaction by loop-mediated isothermal amplification using loop primers. Mol Cell Probe. 16:223–229. doi: 10.1006/mcpr.2002.0415
- Niessen L, Vogel RF. 2010. Detection of Fusarium graminearum DNA using a loop-mediated isothermal amplification (LAMP) assay. Int J Food Microbiol. 140:183–191. doi: 10.1016/j.ijfoodmicro.2010.03.036
- Notomi T, Okayama H, Masubuchi H, Yonekawa T, Watanabe K, Amino N, Hase T. 2000. Loop-mediated isothermal amplification of DNA. Nucleic Acids Res. 28:e63. doi: 10.1093/nar/28.12.e63
- Parida M, Sannarangaiah S, Dash PK, Rao PV, Morita K. 2008. Loop mediated isothermal amplification (LAMP): a new generation of innovative gene amplification technique; perspectives in clinical diagnosis of infectious diseases. Rev Med Virol. 18:407–421. doi: 10.1002/rmv.593
- Pucci N, Ferrante M, Infantino A. 2011. Study of genetic structure of Italian populations of pyrenochaeta lycopersici by AFLP analysis. Acta Horticulturae. 914:121–124. doi: 10.17660/ActaHortic.2011.914.21
- Schneider R, Gerlach W. 1966. Pyrenochaeta lycopersici nov. spec., der Erreger der Korkwurzelkrankheit der Tomate. J Phytopathol. 56:117–122. doi: 10.1111/j.1439-0434.1966.tb02251.x
- Sugiura T, Horinouchi H, Taguchi Y, Hyakumachi M. 2003. Two types of Pyrenochaeta lycopersici, causal pathogen of corky root of tomato. Proceedings of the 8th International Congress of Plant Pathology, Sydney, Australia: Horticulture Australia. 2:346.
- Termohlen GP. 1962. On corky root of tomato and the corky root fungus. Tijdschr Plantenziekten. 68:295–367.
- Tomlinson JA, Barker I, Boonham N. 2007. Faster, simpler, more-specific methods for improved molecular detection of phytophthora ramorum in the field. Appl Environ Microb. 73:4040–4047. doi: 10.1128/AEM.00161-07