ABSTRACT
The contamination of agriculture soils with heavy metals is increasing due to both natural and anthropogenic activities. This has resulted in lowering the yield and quality of agriculture crops. The aim of the present investigation was to determine the effect of Moringa oleifera (M. oleifera) aqueous leaf extract (MALE) on growth and Hg phytoextraction potential of maize cv. Azam under mercuric chloride (HgCl2)-induced stress. Soil was contaminated with different concentrations of HgCl2 (1 and 0.5 mg/kg). The MALE was applied at 5% and 2.5% as seed soaking prior to sowing of seeds in the pots. The M. oleifera leaf was a good source of macronutrients (Na, K, Ca and Mg) and micronutrients (Fe, Zn, Mn, Co and Ni) as well as natural phenolics. The induced HgCl2 stress significantly reduced seed germination (%), shoot dry weight, root dry weight, chlorophyll content and carotenoids content. The HgCl2-induced stress was associated with accumulation of phenolics and Hg in roots. The accumulation of Hg in roots was significantly correlated with phenolics content (r = .8007, p = .000). The exogenous application of MALE significantly ameliorated adverse effects of HgCl2 stress on maize plants. The accumulation of Hg in roots was significantly increased by MALE. It is inferred from findings of the present investigation that MALE served as bioregulator and can be applied to reduce adverse effects of HgCl2 on maize. Moreover, MALE can enhance the Hg phytoremediation potential of maize.
Introduction
Current advancements in the industrial sector have resulted in the contamination of agriculture lands with heavy metals. These heavy metals are highly toxic and inhibit many physiological processes in plants such as photosynthesis, respiration, plant–water relationship, mineral nutrition and thus reduce the yield of agriculture crops (Khan et al. Citation2000; Zornoza et al. Citation2002). Mercury is one of the chief industrial pollutants and is found in various physical and chemical forms (Zahir et al. Citation2005; Palanisamy et al. Citation2012). At room temperature, it is liquid and is present in 1+ or 2+ oxidation states as a cation (Clarkson & Magos Citation2006). Mercury is added to soil in the form of synthetic fertilizers, lime, sludge and manures (Patra & Sharma Citation2000; Nacke et al. Citation2013; Goncalves et al. Citation2014). A greater quantity of mercury is accumulated in the roots of plants, while lesser amounts are accumulated in the shoots (Kumar et al. Citation1995).
Naturally, mercury stress occurs in mercury-rich soils. Mercury destroys plants by inactivation of enzyme system and polynucleotides, causes denaturation of proteins, affects the transportation of essential ions, metallic ions, bursts plasma membranes and destroys cell organelles (Patra et al. Citation2004). It affects the antioxidant defense system of plants (Ortega-Villasante et al. Citation2005), reduces the rates of respiration and photosynthesis, inhibits chlorophyll synthesis, water uptake, potassium, manganese, magnesium and iron accumulation (Boening Citation2000). HgCl2 reduces the primary root elongation in Zea mays (Patra et al. Citation2004).
Phenolics are secondary metabolites that consist of phenolic acid, coumarins, lignans, stilbenes, flavonoids, tannins and lignin. It carries one or more aromatic rings (6-C compounds) with at least one or more than one hydroxyl groups (–OH). High amounts of soluble phenolic compounds create anatomical changes in plants, increase endurance of their cell walls and cause a physiological barrier to protect the plant from harmful effects of heavy metals (Diáz et al. Citation2001). High concentration of heavy metals increases the amount of phenolic compounds in plants. The phenolic compounds, having hydroxyl and carboxyl groups, possess greater tendency to make chelates with heavy metals and hence the antioxidant activities of phenolic compounds are increased (Jung et al. Citation2003). Many plants exude high levels of phenolic compounds when exposed to heavy metals (Winkel-Shirley Citation2002). In menthol extracts, direct chelation of phenols of Nymphaea rhizome with Cr, Pb and Hg was observed (Lavid et al. Citation2001).
Biostimulants are natural compounds that manipulate growth and composition of plants by interacting with endogenous phytohormone groups. Their actions include growth promotion or retardation, flower induction, hastening maturity or senescence, enlarged biomass production, etc. (Narwal Citation2004). Plant phenolic compounds are known for their function as antioxidants due to their free radical-scavenging capabilities (Hassan et al. Citation2015). The same bioactive compounds present in plant extracts may function either as growth stimulator or as inhibitor depending upon the concentration in which it is applied (Popa et al. Citation2002; Ullah et al. Citation2014).
M. oleifera Lam. is a tree species and can tolerate extended periods of drought stress (Martin Citation2000). The Moringa leaf extract has been reported as biostimulant for improving growth of various economically important plant species such as soybean, tea, melon and tomato (Fuglie Citation2000; Yasmeen et al. Citation2014). The present research work was carried out to study the effect of aqueous extracts of M. oleifera on growth and Hg phytoextraction potential of maize plants growing under HgCl2-induced stress.
Materials and methods
The leaves of M. oleifera were collected from wildly growing plants in Pizo region of District Lakki Marwat KP Pakistan. The leaves were shade dried for 2 weeks and ground finely using an electric grinder.
Preparation of M. oleifera aqueous leaf extract
The aqueous extract was prepared by extracting 10 g leaf powder in 200 mL autoclaved distilled water for 72 h. The solution obtained was filtered using a cheese cloth and later with a Whatman No. 1 filter paper. The stock solution was diluted to get 5% and 2.5% solutions. The various solutions formed were stored at 4°C for future use in the experiment. The extract obtained was denoted as MALE.
Bioassay
Seeds of maize (Zea Mays L.) variety Azam were obtained from Agriculture Research Station Sara-e-Nourang Lakki Marwat KP Pakistan. Seeds were sterilized by using 0.2% solution of HgCl2 for 3 min by shaking.
The soil was taken in plastic pots (12 × 10 cm2) and contaminated with 1 mg/kg soil and 0.5 mg/kg soil HgCl2. The control has no salt added. The pots were placed in a glass house of the Department of Botany, University of Science and Technology Bannu. Experimental design was complete randomized design with four replicates for each treatment.
The seeds were soaked in a calculated quantity of various concentrations of MALE separately for 3 h and control was soaked in distilled water. The treatments were control (seeds soaked in distilled water), seeds soaked in distilled water which were sown in soil having 1 mg/kg HgCl2, seeds soaked in distilled water which were sown in soil having 0.5 mg/kg HgCl2, seeds soaked in 5% MALE which were sown in soil having 1 mg/kg HgCl2, seeds soaked in 5% MALE which were sown in soil having 0.5 mg/kg HgCl2, seeds soaked in 2.5% MALE which were sown in soil having 1 mg/kg HgCl2, seeds soaked in 2.5% MALE which were sown in soil having 0.5 mg/kg HgCl2.
Determination of Moringa leaf nutrients composition
Both macronutrients (Na, K, Ca and Mg) and micronutrient content (Fe, Zn, Mn, Ni and Co) of Moringa leaf were determined using the nitric acid and perchloric acid digestion method (Rashid Citation1986). The dried leaf material was added to a mixture of nitric acid and perchloric acid (2:1) in a conical flask. The mixture was heated (150°C) until the color of the mixture was changed and white fumes appeared. The mixture was allowed to cool at room temperature and filtered. The volume of the filtrate was raised to 50 mL using distilled water. Elemental analysis was conducted by using an atomic absorption spectrophotometer (Perkin Elmer Analyst-200, USA).
The plants were harvested 21 days after sowing and were analyzed for the following morphological, physiological and biochemical attributes.
Germination (%)
Germination (%) was calculated when 95% seeds in the control were germinated.(1)
Both the fresh and dry weights of shoot and root were determined by using an electronic balance.
Determination of photosynthetic pigments of maize leaves
The determination of chlorophyll and carotenoids content was made according to the standard method of Arnon (Citation1949).
Determination of total soluble phenolics content of maize leaves
After harvest, roots were analyzed for endogenous phenolics content using the Folin–Ciocalteu method (Wolfe et al. Citation2003). The 0.5-g root tissue was extracted in 10 mL methanol (Sigma Aldrich Co. Ltd). After filtration, 0.5 mL of the methanolic extract was added to 1.5 mL Folin–Ciocalteu reagent and allowed to settle for 5 min. Later on, 1.5 mL sodium carbonate solution (7%) was added to the mixture. The mixture was incubated at room temperature in dark for 90 min. The optical density (OD) was noted at 760 nm using a spectrophotometer (Hitachi’s U-5100 Tokyo, Japan). The readings obtained were compared with a standard curve created from OD of known concentrations of pure gallic acid. The content of phenolics was expressed as µg gallic acid eq./gram root tissue.
Determination of Hg in maize roots
The roots were thoroughly washed with distilled water and extraction of Hg was carried out by the microwave-assisted digestion method. The 0.3-g dry root sample was digested into Teflon bombs. HNO3 (10 mL) was added and digestion of samples was carried out. Hg present in the digestion mixture was transferred into polypropylene tubes and the volume was raised to 10 mL. The detection of Hg in the digest was carried out using an inductively coupled plasma sector field mass spectrometer (Agilent, Japan). For external calibration, In114.8 was used, whereas Rh102.9 was used as an internal standard (Liu et al. Citation2006).
Statistical analyses
The analysis of variance technique (one-way ANOVA) was used for the data analyses. The mean values of all the treatments were compared by using the least significant differences (LSD) test (Steel & Torrie Citation1984). The statistical software Statistix (version 8.1 USA) was used for analysis of coefficient of correlation.
The equation for calculating correlations (Pearson) is as follows:(2) where X is the values in the first set of data and Y is the values in the second set of data. Value of ‘n’ is 21.
Results and discussion
The M. oleifera leaf was characterized for the content of total soluble phenolics content, both macronutrients and micronutrients. The content of total soluble phenolics was 52.12 mg gallic acid/g dry weight. Among macronutrients, Na (204 µg/g), K (197 µg/g), Ca (67 µg/g) and Mg (89 µg/g) were found. Among micronutrients, Fe (42 µg/g), Ni (38 µg/g), Co (48 µg/g), Mn (22 µg/g) and Zn (35.22 µg/g) were detected (). Plant extracts are reported for having higher contents of natural phenolics (Ullah et al. Citation2014; Khattak et al. Citation2015). These natural phenolics exhibit either beneficial or inhibitory effects on target plant species and thus are regarded as allelochemicals (Khan et al. Citation2011).
Table 1. Phytochemical composition of Moringa oleifera leaf.
Seed germination was significantly reduced by contamination of soil with HgCl2 (). However, the reduction in seed germination was significantly higher with increasing soil concentration of HgCl2. The seed soaking with MALE at both the concentrations (5% and 2.5%) significantly ameliorated adverse effects of HgCl2 on seed germination. These results are in agreement with previous findings of Iqbal et al. (Citation2014) that mercury stress significantly reduced seed germination of Albizia lebbeck. Mercury causes seed injuries and reduces seed viability. Mercury causes phytotoxicity by changing the permeability of cell membrane as it reacts with sulfhydryl (SH) groups and phosphate groups, replaces their ions and inhibits protein function (Patra & Sharma Citation2000). It also affects seed germination and embryo growth in SH-rich ligand tissues as mercury interacts with the SH group and forms S–Hg–S bridges making them unstable (Azevedo & Rodriguez Citation2012). The MALE effectively ameliorated HgCl2-induced inhibition in seed germination. This beneficial effect of MALE on seed germination might be due to its phytochemical composition (Pakade et al. Citation2013).
Table 2. Effect of MALE and HgCl2 on seed germination and seedling growth of maize.
Shoot fresh weight was not significantly affected by HgCl2. The effect of MALE on shoot fresh weight was stimulatory in the presence of HgCl2 as compared to untreated control. Higher concentration of HgCl2 significantly reduced shoot dry weight (p < .05). The seed soaking with MALE significantly reduced toxic effects of HgCl2 on shoot dry weight. The effect of HgCl2 on root fresh weight was non-significant as compared to untreated control. The MALE exhibited stimulatory effects on root fresh weight in the presence of HgCl2. The reduction in root dry weight by HgCl2 at both the concentrations was observed. However, MALE exhibited stimulatory effects and reduced adverse effects of HgCl2 on root dry weight (). These results resemble with previous findings of Sahu et al. (Citation2012) and Iqbal et al. (Citation2014) that mercury stress reduced growth attributes in Triticum aestivum and Albizia lebbeck. Mercury inhibits cell division in both roots and aerial parts of plants and thus causes a reduction in biomass production (Patra et al. Citation2004). The seed soaking with MALE prevented maize seedlings from adverse effects of HgCl2 and improved shoot dry weight. These growth promoting effects of MALE are in agreement with previous findings of Makkar and Becker (Citation1996) and Nouman et al. (Citation2011). Root is the organ of plant which comes in direct contact with the contaminant present in the soil. Therefore, its growth is severely affected. The effects of HgCl2 were inhibitory on root growth and caused a reduction in root dry weight. HgCl2 reduces the primary root elongation and inhibits gravimetric response in Zea mays (Patra et al. Citation2004). The MALE effectively protected maize roots from adverse effects of HgCl2.
During the present investigation, contamination of soil with HgCl2 (1 mg/kg soil) caused a reduction in chlorophyll content of maize leaves. The reduction in chlorophyll content was higher with increasing concentration of HgCl2. The seed soaking with MALE effectively prevented leaf chlorophyll of maize from adverse effects of mercury. The carotenoids content was not significantly affected by HgCl2. However, the seed soaking with MALE at 5% in the presence of 1 mg/kg HgCl2 significantly increased carotenoids content as compared to control (). These results are similar to previous findings of Moreno-Jimenéz et al. (Citation2007) who have reported that mercury caused a reduction in chlorophyll content of Marrubium vulgare. Mercury causes inactivation of the magnesium atom from chlorophyll and thus disrupts the photosynthetic machinery (Patra et al. Citation2004). Yasmeen et al. (Citation2014) have reported a significant increase in chlorophyll content of tomato in response to exogenous application of Moringa leaf extract.
Table 3. Effect of MALE and HgCl2 on leaf photosynthetic pigments and total soluble phenolics of maize.
During the present investigation, soil contamination with HgCl2 caused accumulation of phenolics in maize roots and which were further augmented by exogenous application of MALE (). The effect of MALE on phenolics accumulation was higher at 5%. High concentration of metals increases the amount of phenolic compounds in plants (Dietz et al. Citation1999; Rastgoo et al. Citation2014). The activity of enzymes responsible for phenolic compounds synthesis was increased under heavy metal stress and gave rise to de novo biosynthesis of phenolic compounds (Ustun et al. Citation2000). Many plants exude high levels of phenolic compounds when exposed to heavy metals (Winkel-Shirley Citation2002).
Soil contamination with HgCl2 resulted in the accumulation of Hg in roots of maize (). The accumulation of Hg was higher with increasing concentration of HgCl2. The seed soaking with MALE significantly increased the uptake of Hg. The increase in Hg accumulation was higher at 5% MALE. These results resemble with those of Khanna and Rai (Citation1995) and Velasco-Alinsug et al. (Citation2005) that the maximum accumulation of Hg takes place in roots. Higher concentrations of heavy metals such as Hg cause toxicity and lead to growth inhibition, decrease in biomass and death of the plant (Hussain et al. Citation2010). The correlation of Hg content in roots was positively significantly correlated with total soluble phenolics content of roots (r = .8007, r = .000) and carotenoids content of leaves (r = .792, p = .000) (). This indicated that phenolics present in MALE enhanced the endogenous level of total soluble phenolics in maize plants leading to their enhanced tolerance to Hg stress. An increase in soluble phenolics such as intermediates in lignin biosynthesis can reflect the typical anatomical change induced by stressors (Michalak Citation2006) increase in cell wall endurance and the creation of physical barriers preventing cells against the harmful action of heavy metals (Diáz et al. Citation2001). The phenolic compounds, having hydroxyl and carboxyl groups, possess greater tendency to make chelates with heavy metals and hence the antioxidant activities of phenolic compounds are increased (Arora et al. Citation1998). Hg showed direct chelation with polyphenols in methanolic extract of Nymphaea rhizome (Lavid et al. Citation2001). The seed soaking with MALE effectively ameliorated adverse effects of HgCl2-induced stress on growth of maize. The accumulation of phenolics under mercury stress seems as a possible mechanism of maize tolerance to heavy metals. The MALE proved its effectiveness as bioregulator for maize under mercury stress and is therefore recommended for trials in the farmer’s field.
Figure 1. Effect of MALE on bioaccumulation of Hg in roots (LSD: 0.085) of maize. Means sharing a common English letter are statistically similar.
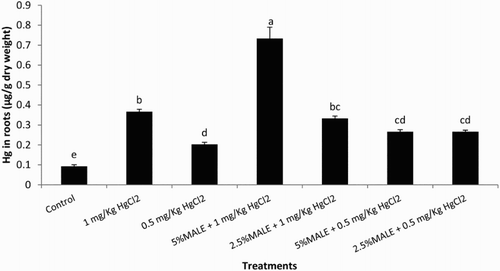
Table 4. Correlations (Pearson).
Disclosure statement
No potential conflict of interest was reported by the authors.
Notes on contributors
Miss Amina Bibi is working as M.Phil. Scholar in the Department of Botany, University of Science and Technology Bannu KP, Pakistan. She is investigating physiological and biochemical basis of heavy metals' stress tolerance in plants.
Dr Faizan ullah has obtained Ph.D. in the subject of plant physiology. Presently he is serving as Assistant Professor in the Department of Botany, University of Science and Technology Bannu KP, Pakistan. He has published 38 research papers in the international journals and one patent. Presently he is involved in research in the area of abiotic stress tolerance in plants and biofuel production technologies.
Prof Dr Sultan Mehmood has obtained Ph.D. in the plant ecology and has published 100 research papers in international journals. Presently he is working as Dean of Science in the University of Science and Technology Bannu KP, Pakistan.
Miss Kalsoom Bibi is enrolled as M.Phil. scholar in the Department of Botany, University of Science and Technology Bannu KP, Pakistan. She is working on abiotic stress tolerance in plants.
Mr Saad Ullah Khan is working as a lecturer in the Department of Botany, University of Science and Technology Bannu KP, Pakistan. He has published 30 research papers in the field of botany.
Mr Adnan Khattak is M.Phil. Scholar in the Department of Botany, University of Science and Technology Bannu KP, Pakistan. He has published three research papers in international journals in the field of plant physiology.
Mr Rehman Ullah Khan is working as Ph.D. scholar in the Department of Botany, University of Science and Technology Bannu KP, Pakistan. He has published 35 research papers in journals of international reputation.
References
- Arnon DI. 1949. Copper enzymes in isolated chloroplasts, polyphenol oxidase in Beta vulgaris L. Plant Physiol. 24:1–15. doi: 10.1104/pp.24.1.1
- Arora A, Nair MG, Strasburg GM. 1998. Structure–activity relationships for antioxidant activities of a series of flavonoids in a liposomal system. Free Radic Biol Med. 24:1355–1363. doi: 10.1016/S0891-5849(97)00458-9
- Azevedo R, Rodriguez E. 2012. Phytotoxicity of mercury in plants: a review. J Bot. 2012:1–6. doi: 10.1155/2012/848614
- Boening DW. 2000. Ecological effects, transport, and fate of mercury: a general review. Chemosphere. 40:1335–1351. doi: 10.1016/S0045-6535(99)00283-0
- Clarkson TW, Magos L. 2006. The toxicology of mercury and its chemical compounds. Crit Rev Toxicol. 36:609–662. doi: 10.1080/10408440600845619
- Diáz J, Bernal A, Pomar F, Merino F. 2001. Induction of shikimate dehydrogenase and peroxidase in pepper (Capsicum annum L.) seedlings in response to copper stress and its relation to lignification. Plant Sci. 161:179–188. doi: 10.1016/S0168-9452(01)00410-1
- Dietz KJ, Baier M, Kramer U. 1999. Free radicals and active oxygen species as mediators of heavy metal toxicity in plants. In: Prasad MNV, Hagemeyer J, editors. Heavy metal stress in plants. From molecule to ecosystems. Berlin: Springer; p. 73–97.
- Fuglie LJ. 2000. New uses of Moringa studied in Nicaragua. ECHO Development Notes #68, June, 2000.
- Goncalves Jr, Nacke AC, Schwantes D, Coelho GF. 2014. Heavy metals contamination in Brazilian agriculture soils to application of fertilizers. In: Hernandez-Soriano MC, editor. Environmental risk assessment of soil contamination. Intech. ISBN 978-983-51-1235-8; doi:10.5772/57268.
- Hassan MA, Fuertes MM, Sanchez FJR, Vicente O, Boscaiu M. 2015. Effects of salt and water stress on plant growth and on accumulation of osmolytes and antioxidant compounds in cherry tomato. Not Bot Horti Agrobot Cluj-Nap. 43:1–11.
- Hussain K, Sahadevan KK, Salim N. 2010. Bio-accumulation and release of mercury in Vigna mungo (l.) hepper seedlings. J Stress Physiol Biochem. 6:56–63.
- Iqbal MZ, Shafiq M, Athar M. 2014. Phytotoxic effects of mercury on seed germination and seedling growth of Albizia lebbeck (L.) Benth. (Leguminosae). Adv Environ Res. 3:207–216. doi: 10.12989/aer.2014.3.3.207
- Jung C, Maeder V, Funk F, Frey B, Sticher H, Frossard E. 2003. Release of phenols from Lupinus albus L. roots exposed to Cu and their possible role in Cu detoxification. Plant Soil. 252:301–312. doi: 10.1023/A:1024775803759
- Khan AG, Kuek TM, Chaudhury TM, Khoo CS, Hayes WJ. 2000. Role of plants, mycorrhizae and phytochelators in heavy metal contaminated land remediation. Chemosphere. 41:197–207. doi: 10.1016/S0045-6535(99)00412-9
- Khan MA, Qureshi RA, Ullah F, Gilani SA. 2011. Phytotoxic effects of selected medicinal plants collected from Margalla Hills, Islamabad Pakistan. J Med Plants Res. 5:4671–4675.
- Khanna S, Rai VK. 1995. Amelioration of mercury toxicity in radish (Raphanus sativus L.) seedlings by L-proline and other amino acids. Indian J Exp Biol. 3:766–770.
- Khattak A, Ullah F, Wazir SM, Shinwari ZK. 2015. Allelopathic potential of Jatropha curcas aqueous extracts on seedling growth of wheat. Pak J Bot. 47:2449–2454.
- Kumar PBAN, Dushenkov V, Motto H, Raskin I. 1995. Phytoextraction: the use of plants to remove heavy metals from soils. Environ Sci Technol. 29:1232–1238. doi: 10.1021/es00005a014
- Lavid DN, Schwartz A, Yarden O, Telor E. 2001. The involvement of polyphenols and peroxidase activities in heavy metal accumulation by epidermal glands of waterlily (Nymphaeceaea). Planta. 212:323–331. doi: 10.1007/s004250000400
- Liu D, Wang X, Chen Z, Xu H, Wang Y. 2006. Influence of mercury on chlorophyll content in winter wheat and mercury bioaccumulation. Plant Soil Environ. 56:139–143.
- Makkar HPS, Becker K. 1996. Nutritional value and antinutritional components of whole and ethanol extracted Moringa oleifera leaves. Anim Feed Sci Technol. 63:211–228. doi: 10.1016/S0377-8401(96)01023-1
- Martin LP. 2000. The Moringa Tree: Revised in 2000 by Kristin Davis, pp. 1–14.
- Michalak A. 2006. Phenolic compounds and their antioxidant activity in plants growing under heavy metal stress. Polish J Environ Stud. 15:523–530.
- Moreno-Jiménez E, Peñalosa JM, Esteban E, Carpena-Ruiz RO. 2007. Mercury accumulation and resistance to mercury stress in Rumex induratus and Marrubium vulgare grown in perlite. J Plant Nutr Soil Sci. 170:485–494. doi: 10.1002/jpln.200625238
- Nacke H, Goncalves Jr AC, Schwantes D, Nava IA, Strey L, Coelho GF. 2013. Availability of heavy metals (Cd, Pb, Cr) in agriculture from commercial fertilizers. Arch Enviorn Contam Toxicol. 64:537–544. doi: 10.1007/s00244-012-9867-z
- Narwal SS. 2004. Allelopathy in crop production. Jodhapur: Scientific Publishers. p. 326–332.
- Nouman W, Siddiqui MT, Basra SMA. 2011. Moringa oleifera leaf extract: an innovative priming tool for rangeland grasses. Turk J Agric Forestry. 36:65–75.
- Ortega-Villasante C, Rellán-Álvarez R, Del Campo FF, Carpena-Ruiz RO, Hernández LE. 2005. Cellular damage induced by cadmium and mercury in Medicago sativa. J Exp Bot. 56:2239–225. doi: 10.1093/jxb/eri223
- Pakade V, Cukrowska E, Chimuka L. 2013. Comparison of antioxidant activity of Moringa oleifra and selected vegetables in South Africa. S Afr J Sci. 109:1–5.
- Palanisamy K, Lenin M, Mycin R. 2012. Effects of mercuric chloride on growth and cytotoxicity of soybean glycine max (L.). Hepper Int J Toxicol App Pharmacol. 2:7–41.
- Patra M, Sharma A. 2000. Mercury toxicity in plants. Bot Rev. 66:379–422. doi: 10.1007/BF02868923
- Patra MAB, Bhowmik NC, Bandopadhyay B, Archana SK. 2004. Comparison of mercury, lead and arsenic with respect to genotoxic effects on plant systems and the development of genetic tolerance. Environ Experi Bot. 52:199–223. doi: 10.1016/j.envexpbot.2004.02.009
- Popa VI, Agache C, Beleca C, Popa M. 2002. Polyphenols from spruce bark as plant growth regulator. Crop Res. 24:398–406.
- Rashid A. 1986. Mapping zinc fertility of soils using indicator plants and soils-analyses [Ph.D. dissertation]. University of Hawaii, HI, USA.
- Rastgoo L, Alemazeh A, Tale AM, Tazangi SE, Eslamzadeh T. 2014. Effects of copper, nickel and zinc on biochemical parameters and metal accumulation in gouan, Aeluropus littoralis. Plant Knowledge J. 3:31–38.
- Sahu GK, Upadhyay S, Saho BB. 2012. Mercury induced phytotoxicity and oxidative stress in wheat (Triticum aestivum L.) plants. Physiol Mol Biol Plant. 18:21–31. doi: 10.1007/s12298-011-0090-6
- Steel RGD, Torrie JH. 1984. Principles and procedures of statistics. New York: McGraw Hill.
- Ullah F, Ullah A, Wazir SM, Shinwari ZK. 2014. Phytotoxic effects of safflower yellow exposure on seed germination and early seedling growth of canola (Brassica napus L). Pak J Bot. 46:1741–1746.
- Ustun AS, Keles Y, Oncel I. 2000. Interactive effect of temperature and heavy metal stress on the growth and some biochemical compounds in wheat seedlings. Environ Pollut. 107:315–320. doi: 10.1016/S0269-7491(99)00177-3
- Velasco-Alinsug MP, Rivero GC, Quibuyen TAO. 2005. Isolation of mercury binding peptides in vegetative parts of Chromolaena odorata. Z Naturforsch. 60:252–259.
- Winkel-Shirley B. 2002. Biosynthesis of flavonoids and effects of stress. Curr Opin Plant Biol. 5:218–223. doi: 10.1016/S1369-5266(02)00256-X
- Wolfe K, Wu X, Liu RH. 2003. Antioxidant activity of apple peels. J Agric Food Chem. 51:609–614. doi: 10.1021/jf020782a
- Yasmeen A, Nouman W, Basra SMA, Wahid A, Rehman HU, Hussain N, Afzal I. 2014. Morphological and physiological response of tomato (Solanum lycopersicum L.) to natural and synthetic cytokinin sources: a comparative study. Acta Physiol Plant. 36:3147–3155. doi: 10.1007/s11738-014-1662-1
- Zahir F, Rizwi SJ, Haq SK, Khan RH. 2005. Low dose mercury toxicity and human health. Environ Toxicol Pharmacol. 20:351–360. doi: 10.1016/j.etap.2005.03.007
- Zornoza P, Vázquez S, Esteban E, Fernández-Pascual M, Carpena R. 2002. Cadmium-stress in nodulated white lupin: strategies to avoid toxicity. Plant Physiol Biochem. 40:1003–1009. doi: 10.1016/S0981-9428(02)01464-X