ABSTRACT
The aim of this study was to investigate what kind changes in the soil fertility parameters occur depending on different farming methods. The field experiment was performed on sandy loam Luvisol during 2008–2014. The following treatments were carried out: organic (ORG), organic with farmyard cattle manure (ORGFYM) and conventional with farmyard cattle manure and mineral fertilizers (CONFYM). Soil samples were collected yearly in September and analyzed for P, K, Mg and Ca by the Mehlich III method. In parallel, ammonium lactate extractable P and K (AL method), and ammonium acetate extractable Mg and Ca (NH4OAc-method) were determined. The Pearson correlation coefficients between the analysis results achieved by the Mehlich III method and alternative methods were calculated. In all the treatments, no significant changes in soil Corg content were established over seven years. A significant (p < .05) decrease in soil pH was shown for the CONFYM treatment. Organic management without nutrients’ input does not decrease the content of PAL in the soil but considerable decrease in the soil KAL content was revealed. The application of cattle manure (60 t ha−1 for the 5-year crop rotation) in both organic and conventional treatments sustained the status of available nutrients in the soil. The Pearson correlation coefficients between Mehlich III and AL methods for P (r = 0.770, p < .01) were lower than for K (r = 0.922, p < .01). The highest correlation between Mehlich III and NH4OAc-methods was found in the case of Mg (r = 0.951, p < .01) whereat for Ca the correlation was somewhat weaker (r = 0.841, p < .01). It was also shown that the PMehlich III/PAL quotient was inversely proportional with the CaMehlich III values.
Introduction
In recent years, organic farming has been expanding due to interests of consumers and EU agricultural policies. Organic farming is believed to improve soil fertility by enhancing soil organic matter (SOM) content (Pikuła & Rutkowska Citation2014). SOM plays a crucial role in maintaining the sustainability of cropping systems by improving soil physical, chemical and biological properties (Pospisilova et al. Citation2011, Citation2015; Fageria Citation2012). Depending on the stocks of SOM, organic cropping systems have caused different changes in soil organic carbon (SOC) content (Scherer et al. Citation2011; Pikuła & Rutkowska Citation2014; Kauer et al. Citation2015). There are differences among organic fertilizers in the quality and quantity of organic matter, for example between farmyard manure (FYM) and digestate from biogas plants (Losak et al. Citation2016). Stockdale et al. (Citation2002) have mentioned that although nutrient management in organically managed soils is fundamentally different to soils managed conventionally, the underlying processes supporting soil fertility are not. The same nutrient cycling processes operate in the organically farmed soils as those that are farmed conventionally although their relative importance and rates may differ. In the organically farmed soils, in the absence of regular fertilizer inputs, nutrient reserves in less-available pools will be of greater importance (Stockdale et al. Citation2002).
According to Leifeld and Fuhrer (Citation2010) the claim of beneficial effects of organic farming on SOC is premature and the reported advantages of organic farming for SOC are largely determined by higher and often disproportionate application of organic fertilizer compared to conventional farming. They concluded that in the few studies where crop rotation and organic fertilization were comparable in both systems, no consistent difference in SOC was found. Also, the study of Bakken et al. (Citation2006) gave no unambiguous support to the hypothesis that organic farming increases inherent overall soil fertility.
Organic production systems have been criticized for relying on soils’ nutrient reserves, which have been built up by fertilizer additions prior to organic management; organic farming cannot therefore sustain itself for longer (Gosling & Shepherd Citation2005). When the duration of such low-input cultivation systems are long term, a depletion of soil nutrients will take place (Ellmer & Baumecker Citation2005; Sánchez de Cima et al. Citation2015; Masilionyté & Maikšténiené Citation2016). However, regular applications of manure led to balanced nutrient fluxes (Ellmer & Baumecker Citation2005; Masilionyté & Maikšténiené Citation2016). Long-term application of FYM promoted microbiological activities and P cycling (Parham et al. Citation2002). FYM is also a better source of available potassium and magnesium (Kulhánek et al. Citation2014).
In organic systems, plant production depends primarily on nutrient cycling in soils that are controlled by microbes and their activities (Monokrousos et al. Citation2006). A number of bacteria and fungi are known to increase availability of P to plants either by mineralization of organic phosphates or by solubilization of insoluble inorganic phosphates in soils through the production of organic acids (Richardson & Simpson Citation2011; Ram et al. Citation2015). Soil microbes have been reported to play a key role in the natural K cycle and, therefore, potassium-solubilizing microorganisms present in the soil could make potassium available for uptake by plants (Parmar & Sindhu Citation2013; Meena et al. Citation2014). The nutrients’ status and fertility of soils depend highly on their enzymatic activities. According to Wolinska and Stepniewska (Citation2012) dehydrogenase activity (DHA) is one of the most adequate, important and one of the most sensitive bioindicators relating to soil fertility.
Different analytical tests have been implemented to evaluate the status of nutrients in soil available for plants. The relevance and comparability of soil tests are largely discussed by many researchers. In the Estonian service of agricultural chemistry, the transition from double lactate (DL) and ammonium lactate (AL) extraction methods to the multi-element extraction with the Mehlich III solution has been carried out since 2004. Before this transition, comparative analyses of the set of soil samples characterizing the predominant soil types of Estonia were carried out. The results of these analyses showed that the contents of P, K, Ca, Mg, Mn and Cu determined by the Mehlich III extraction method correlated significantly with the respective results obtained with the DL- and AL-extraction solutions (Loide et al. Citation2004). However, according to the unpublished data by Ph.D. Roostalu (Citation2014) the suitability of the Mehlich III extraction is questionable, especially for assessment of P status because the results’ deviation for some soil types is too large. Furthermore, the Mehlich III results obtained with different end-point analytical techniques –Inductively Coupled Plasma Optical Emission Spectroscopy and Microwave Plasma-Atomic Emission Spectrometry – may remarkably differ (Matula Citation2010; Tõnutare et al. Citation2015).
In the present work several research questions were set. Firstly, does the soil depletion concerning nutrients available for plants come about when the soil is managed organically without nutrients’ input for over seven years? Secondly, does in this case the application of farmyard cattle manure once during the crop rotation to sustain the soil fertility status. The third research question rose up relating to the data of soil analyses which were carried out in parallel by several methods. As shown by Roostalu (Citation2014) and Wünscher et al. (Citation2015), the content of phosphorus extracted by the Mehlich III solution depended largely on the soil texture and carbonate content. Consequently, in the soil conditions of sandy loam with the moderate content of carbonates, we would like to investigate which are the correlations for phosphorus and potassium between the analyzed data of soils extracted in parallel by the Mehlich III and by the AL methods. Also the correlations for magnesium and calcium between different soil extraction methods were investigated.
Materials and methods
The field experiment was performed in Central-Estonia at Olustvere (58° 33′ N, 25° 34′ E) during 2008–2014. The soil type was stagnic Luvisol according to the WRB 2014 classification.
The texture of the soil was sandy loam (61.6% sand, 30.4% silt and 8.0% clay).
In 2007, before the experiment was set up the average agrochemical parameters of the soil in the 6 ha experiment area were the following: pHKCl 5.96, organic matter content 2.78%, PAL 96, KAL 108, CaNH4OAc 735 and MgNH4OAc 64 mg kg−1. Formerly, in this area, five-field crop rotation had been cultivated according to the principles of organic farming. During 2002–2007 this area was cultivated and harvested whereas no kind of mineral and organic fertilizers has been used.
In 2008, the crop rotation was marginally modified and established as follows: winter rye (Secale cereale L.), potato (Solanum tuberosum L.), oats (Avena sativa L.), barley (Hordeum vulgare L.) with undersown red clover (Trifolium pratense L.) and red clover. The size of each field was 1.2 ha, which was divided into three equal parts (0.4 ha) between the cultivation methods. Since 2007, the following treatments have been carried out: organic without manure (ORG), organic with solid cattle farmyard manure (ORGFYM) and conventional where solid cattle manure, mineral fertilizers and pesticides were used (CONFYM). The tillage method in all three treatments was the moldboard ploughing to a depth of 20 cm in autumn. In all treatments clover was cut and ploughed into the soil in the beginning of July. Straw and crop residues were not removed from the field. Weeds were controlled in the oats and barley fields after sowing and in the rye field at the end of April by spring-tine harrowing. In the potato field the inter-rows were harrowed and cultivated two and three times, respectively.
In the ORGFYM and CONFYM treatments, in the rye field after the rye harvesting and prior to the autumn ploughing, solid cattle manure (FYM) at the rate of 60 t ha−1 fresh weight was applied for potato grown in the next year. For the 5-year crop rotation the following amounts of nutrients were applied by FYM into the soil: N 287, P 65, K 164, Ca 130 and Mg 60 kg ha−1.
In the CONFYM treatment the crops received mineral complex fertilizers at the following rates: potato – N 60, P 60, K 120 kg ha−1, oats – N 72, P 18, K 36 kg ha−1 and barley with undersown clover – N 48, P 12, K 24 kg ha−1. For rye N 15, P 30, K 75 kg ha−1 as complex fertilizers were given before sowing and N 34+34 kg ha−1 as ammonium nitrate in the next spring. Red clover alone did not receive any mineral fertilizers. During a 5-year crop rotation the CONFYM treatment received from mineral fertilizers and cattle manure in total the following amounts of plant nutrients: N 550, P 185, K 419, Ca 130 and Mg 60 kg ha−1 (). The CONFYM area was treated with several synthetic pesticides during the vegetation period depending on the crop. A more detailed description of the pesticides used is presented in Järvan and Edesi (Citation2015).
Table 1. Fertilizer application for the 5-year crop rotation during 2008–2014.
Every year, the harvested yields of cereals and potato tubers were documented (). Their chemical composition (content of P, K, Ca and Mg) was determined at the Biochemistry Laboratory of the Estonian University of Life Sciences. The nutrients’ removal with yields from the rotation fields was evaluated yearly. The average nutrient balances for 2008–2014 are also presented.
Table 2. The crop yields during the experiment period, nutrient removal with yields and nutrient balances for the 5-yeara crop rotation.
Every year in September, after crop harvesting and before manure application the soil samples were taken for chemical analyses from all treatments of all rotation fields. Every sample was collected from 45 randomly selected points from the 0–20 cm layer. After air-drying the soil samples were homogenized by screening through a 2-mm sieve and archived into carton boxes.
To compare the results of the past and the future measurements and to collect additional information for comparison of the Mehlich III and AL methods, all soil samples were analyzed in parallel with these methods for the content of mineral nutrients. At the Agricultural Research Centre located at Saku, soils were tested for phosphorus (P), potassium (K), magnesium (Mg) and calcium (Ca) content according to the Mehlich III method (Mehlich Citation1984). At the Biochemistry Laboratory of the Estonian University of Life Sciences, for available phosphorus (PAL) and potassium (KAL) the soils were extracted with the AL method. That is, AL (0.1 M) and acetic acid (0.4 M) at pH 3.75 and a soil/solution ratio of 1:20 (Egner et al. Citation1960) were used. PAL was determined by flow injection analysis with the use of the Tecator ASTN 9/84 (FiaStar5000). The content of KAL was determined from the same solution by the flame photometric method. The exchangeable magnesium and calcium in the soils were extracted with 1 M ammonium acetate solution at pH 7.0 and a soil/solution ratio of 1:10. Soil magnesium (MgNH4OAc) content was determined by flow injection analysis with the use of the Tecator ASTN 90/92 (Methods of soil analysis Citation1982). From the same solution calcium (CaNH4OAc) was determined by the flame photometric method. Soil pH in 1 M KCl suspension was measured at a ratio of 10 g:25 ml. Organic carbon content in the soil was determined after dry combustion by elementary analysis (ISO 10694:1995).
In September 2012, 2013 and 2014, the DHA of soils was investigated. The soil samples in three replications were taken from the rotation fields of all treatments by a random method from the 0 to 20 cm layer. Samples were sieved (2 mm) and kept at 4°C until analysis. DHA was measured in accordance with Tabatabai (Citation1982) in 5 g soil samples incubated at 30°C for 24 h in the presence of an alternative electron acceptor triphenyltetrazoliumchloride. The red-tinted product triphenylformazan (TPF) was extracted with acetone and measured in a spectrophotometer at 546 nm.
Weather conditions of the experiment period were quite different. As regards precipitation, the vegetation periods (April–September) of years 2008, 2012 and 2014 were characterized as very rainy (450–479 mm), 2009 and 2010 were normal (about 390 mm) and 2011 and 2013 with low precipitation (300 and 282 mm). The mean air temperature (April–September) reached from 12.3°C (2012) up to 14.3°C (2011).
The coefficients of linear correlation were calculated between the analysis results achieved by the AL and Mehlich III methods for P and K, and between NH4OAc and Mehlich III methods for Mg and Ca. The correlation coefficients were calculated separately for each year and treatment as well as above years and treatments, and above measurement together. Statistical significance of the correlation coefficients was assessed by t-test at the levels p < .01 and p < .05. The means of MS Excel were used for statistical calculations.
Results and discussion
Organic carbon (Corg)
Changes in soil Corg content caused from different farming systems are difficult to evaluate because the Corg content of soil changes very slowly (Körschens et al. Citation1998; Kauer et al. Citation2015). The annual changes of Corg content amounted only to about 0.01% of Corg, even under extreme changes of the fertilizing system (Körschens et al. Citation1998).
To assess the trend in soil Corg content as affected by different managements, we calculated the mean value of Corg in soils from all five fields of rotation together for each treatment (). In our experiment that lasted only seven years, no significant changes in the soil Corg content occurred. However, a light tendency to the decrease of Corg in the soil of ORG treatment became evident. In the ORGFYM and CONFYM treatments, 8.2 t/ha of organic dry matter was given to soil per 5-year crop rotation as cattle manure. As known, carbon from manure undergoes mineralization within a relatively short period (Pikuła & Rutkowska Citation2014). Nevertheless, a light tendency to increase Corg in the soil of the ORGFYM and CONFYM treatments appeared.
Table 3. The organic carbon (Corg) content and pHKCl of soils at the beginning (2008) and at the end (2014) of the experiment.
In many experiments the long-term application of FYM increased the Corg content in soil (Pikuła & Rutkowska Citation2014; Šimon & Czakό Citation2014; Sanchez de Cima et al. Citation2015). However, the effect of manure application had a weaker impact on Corg accumulation than the effect of crop rotation, especially when clover with grasses was included (Pikula & Rutkowska Citation2014).
Generally, the duration of our study was too short for looking to significant changes in the soil Corg content.
Soil pH
For both organic treatments, the light tendency to soil acidification during seven years was registered (). For the CONFYM treatment the significant (p < .05) decrease of soil pH occurred as to compare the results of the years 2008 and 2014. This decrease could have been caused partially for the mineral fertilizers applied in the CONFYM treatment. As is known, several of them can accelerate the acidification of soils. Overall, however, the lasting tendency to acidification is absolutely normal for the many types of Estonian soils because here in Estonia the precipitation exceeds the evaporation (Järvan & Järvan Citation2010).
Phosphorus
The concentration of determinable phosphorus in soil solution is highly dependent on the extracting agent and its pH, soil:solution ratio, shaking duration, different soil properties and other factors (Sardi & Füleky Citation2002; Eriksson Citation2009; Otabbong et al. Citation2009; Schroder et al. Citation2009; Wünscher et al. Citation2015). The composition of active elements in the extraction solution is probably the main reason for the large variations observed in the amount of P extracted with the different methods (Eriksson Citation2009). The components in PAL extract have a good capacity to chelate cations and to desorb P from Al- and Fe-oxides. In the PMehlich III method an additional agent, NH3F, is included for extracting adsorbed P (Eriksson Citation2009). However, NH3F is not efficient in extracting P from Ca-phosphates, but very efficient in extracting P from Al- and Fe-oxides, which could result in overestimation of available P in Al- and Fe-oxides (Eriksson Citation2009).
On the basis of the results of individual soil samples (n = 105) in which P was determined in parallel by means of the Mehlich III method and the AL method we calculated for both methods the yearly mean values for P (and also for K, Mg and Ca) content in the soil from all five crops together. The results are presented graphically ().
Figure 1. The dynamics of elements in differently managed soils: (a) determined by the Mehlich III method and (b) determined by the AL method (P and K), and by NH4OAc-method (Mg and Ca). Treatments: ORG – organic without manure, ORGFYM – organic with farmyard cattle manure, CONFYM – conventional with farmyard cattle manure, mineral fertilizers and pesticides.
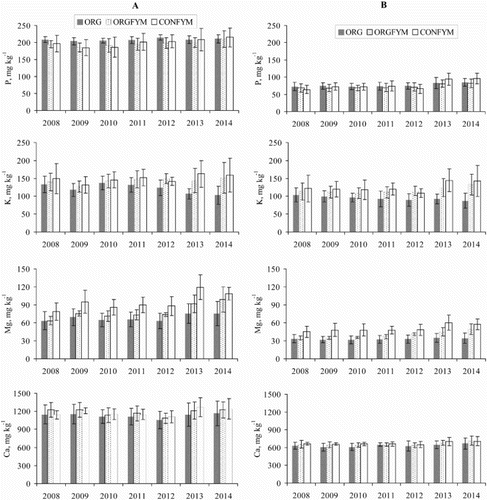
At first the soil samples were tested for P, K, Mg and Ca according to the Mehlich III method ((a)). The Mehlich III solution is one of the strongest extractants (pH 2.5) to estimate bioavailable nutrients from soils (Schroder et al. Citation2009; Kulhánek et al. Citation2014). The values of PMehlich III were very high for all the soils sampled during the experiment period. In the case of ORG treatment, no differences were observed in the soil PMehlich III content which ranged between 209 ± 9 (2008) and 211 ± 12 mg kg−1 (2014), as the average for five-field crop rotation. In the ORGFYM treatment the PMehlich III content, which was 195 ± 11 mg kg−1 in the soil in 2008, was on the same level as for the ORG treatment, that is, 210 ± 24 mg kg−1 at the end of experiment period (2014). In the CONFYM treatment, light fluctuation of the soil PMehlich III content occurred between 185 ± 24 mg kg−1 (2009) and 215 ± 28 mg kg−1 (2014).
In parallel we tested the soils for P available for plants also with an alternative method which is based on a weaker extractant (AL, pH 3.7). The average initial (2008) PAL contents in the soils of the ORG, ORGFYM and CONFYM treatments were 72 ± 11, 68 ± 12 and 64 ± 12 mg kg−1, respectively ((b)). For the soils of both organic treatments, no changes in the PAL content occurred from 2008 until 2012. However, since the sixth year of experiment (2013), there appeared an increase in PAL content in the soils of both treatments. In 2014 the average PAL contents for the ORG and ORGFYM treatments reached up to 85 ± 12 and 82 ± 12 mg kg−1, respectively.
The increase in PAL content in 2013 could have been caused by several factors. Firstly, it could have been caused by very different amounts of precipitation of neighboring years. The year 2012 was very rainy because from April to the end of September there were rains of 450 mm in total, including 94 mm during one and half months before soil sampling at the end of September. In such conditions in this year the leaching of available nutrients from the topsoil layer might occur. The vegetation period of 2013, contrary to 2012, was very dry (282 mm during April–September) and one and half months before soil sampling the sum of precipitation reached only 14 mm. In 2013, the upward movement of soluble nutrients from soil bottom layers with evaporation stocks might have taken place, and this probably was one reason for the increase of PAL (also KAL, and exchangeable Mg and Ca) contents for all treatments in 2013. As mentioned by Li et al. (Citation2014), the evaporation process moves water and soluble salts upward and may bring the risk of salinization to the topsoil.
Secondly, the increase of PAL for the ORGFYM treatment may be partially as a result of manure application, because for 5-field crop rotation with FYM 65 kg/ha of total P was given under potato thereat only 35 kg P ha−1 (as an annual average, calculated on the basis of crop yields and their P content) was removed with yields of all rotation crops.
Thirdly, the increase of PAL may have been caused by the cumulative effect of microbial activity. In organically managed soils where no pesticides and mineral fertilizers are used the microbial activities are quite high (Monokrousos et al. Citation2006). Thereby, a number of microorganisms play a significant role in the solubilization of soil phosphates (Mengel & Kirkby Citation1987; Ram et al. Citation2015). It is also well known that the application of cattle manure promoted microbiological activities and P cycling (Parham et al. Citation2002). In our other research on the basis of the present field experiment the microbial communities and their activities were discussed. It was evident that since 2013 the DHA in organically managed soils was increasing (Järvan et al. Citation2014). In 2014, the increase of soil DHA continued. According to the unpublished data of Liina Edesi, the average values of DHA for five-field crop rotation in the soil of the ORG and ORGFYM treatments were 3.56 and 4.35 (TPF µg/g/h), respectively. Therefore, it may be supposed that the activity of soil microbes also contributed to the increasing contents of PAL in organically managed soils.
For the soil samples of the CONFYM treatment, the five-field average PAL contents were quite stable in the first years of the experiment but showed a significant increase since 2013. In 2014 the PAL content reached up to 96 ± 16 mg kg−1, as the average of 5-field crop rotation. One reason for this significant increase in the soil PAL content was, in all likelihood, the great amount of plant nutrients which accumulated through the yearly application of fertilizers. During 5-year crop rotation, totally 120 kg P ha−1 with mineral fertilizers and 65 kg P ha−1 with FYM were given to the soil of CONFYM treatment. At an average, 73 kg P ha−1 was removed from the rotation fields, as calculated on the basis of annual crop yields and their chemical composition.
It is also interesting to note that, as a rule, the soils sampled from the field where red clover was ploughed into the soil showed higher contents of PAL than soils sampled under other crops. According to Talgre and Lauringson (Citation2015) a rapid release of N, P and K from belowground biomass of red clover occurred. By Mengel and Kirkby (Citation1987) microbial density in the rhizosphere of red clover was by 4- and 8-fold higher than that of oats and barley whereas microbes do not appear to be major competitors with plant for phosphate uptake. Consequently, it is quite likely that in the conditions of our experiment the concentration of bioavailable P increases shortly after ploughing clover into the soil. According to Feiziene et al. (Citation2016) red clover involved in the crop rotation contributes to the soil sustainability and increases the reserves of available plant nutrients.
On the basis of the above, it can be concluded that for this 7-year period, organic farming does not decrease the content of available phosphorus in the soil.
Potassium
For all the treatments, the contents of potassium in the soil samples taken from the arable layer showed more fluctuating tendencies than the contents of phosphorus. The standard deviations for the five-field average K-content of soil were quite high for both extraction methods. The average initial (2008) KMehlich III contents in the soils of ORG, ORGFYM and CONFYM treatments were 132 ± 23, 140 ± 25 and 149 ± 42 mg kg−1, respectively ((a)). The average initial KAL contents for the treatments were 104 ± 20, 114 ± 24 and 122 ± 37 mg kg−1, respectively ((b)).
For ORGFYM and CONFYM treatments, no practically clear tendencies in KMehlich III contents were revealed during the experiment period. For ORG treatment, the KMehlich III content stayed more or less stable during the first five years but then fell strongly down, reaching 103 mg kg−1 (2014). It means that for this treatment – without nutrients’ input in such a light soil texture conditions (sandy loam) – a lasting depletion of soil potassium will take place in the future. This soil in which the clay content was very low, cannot release potassium in quantities needed for the covering potassium removed with the yields. We hereby mention that for the ORG treatment the nutrient balance for potassium was strongly negative (−105 kg ha−1 for 5-year crop rotation).
The soil samples of ORG treatment analyzed for AL extractable potassium (KAL) showed in results of driving years a more little decreasing tendency than the results of KMehlich III. For ORG treatment the average KAL content decreased during seven years from 104 up to 87 mg kg−1. In the soil samples of ORGFYM and CONFYM treatments, the annual averages of KAL contents were practically stable up to the sixth year of research but since 2013 a remarkable rise in KAL for both the treatments was shown. In 2014, the average KAL contents of ORGFYM and CONFYM treatments increased by 19 and 20 mg kg−1, respectively, when compared with the values in 2008.
During our study, there was no evidence that the soil KAL content was influenced by the crop cultivated. At first, we supposed that the KAL content in the soil samples from the potato field of ORGFYM and CONFYM treatments might be higher than under other crops because for potato a big amount of potassium (164 kg/ha, as the average of years) was applied with FYM. It is well known that FYM is a good source of bioavailable potassium (Kulhánek et al. Citation2014). Depending on potato yield which for ORGFYM treatment varied from 10.5 to 37.7 t ha−1 and for CONFYM treatment from 38.1 up to 64.0 t ha−1, with tubers K was removed yearly 56–142 and 163–267 kg ha−1, respectively. Must take into consideration that in CONFYM treatment for potato also 120 kg ha−1 of potassium with mineral fertilizer was applied.
We assume that the increasing tendency in the soil KAL dynamics in ORGFYM and CONFYM treatments in the latest years of our research might have been evoked also by microorganisms capable of releasing potassium from fixed K forms of soil. As is known, their activity will be significantly enhanced by the FYM applied (Edesi et al. Citation2012).
The studies of other researchers have shown different results and tendencies in dynamics of available K in soil, depending on experiment conditions. According Andrist-Rangel et al. (Citation2007) the annual balances of exchangeable K during long-term research were equally negative for the organic and conventional management systems. Løes and Øgaard (Citation2003), on the contrary, mentioned that in organic dairy farms aiming at self-sufficiency with nutrients, topsoil KAL concentrations were medium high and did not decrease during the 6–14 years of study period. Comparing soil nutrients’ dynamics in differently farmed crop rotations, Sanchez de Cima et al. (Citation2015) have shown that by organic farming after the first 5-year rotation a significant decrease in available K occurred. According to Bučiene et al. (Citation2003) an organic regime receiving FYM maintained its K status and seemed the most sustainable of the low-input systems.
From the soil analysis results of our experiment, it can be concluded that organic farming without nutrients’ input might lead to soil depletion in potassium available for plants. To sustain the soil potassium status, regular application of FYM is needed.
Magnesium and calcium
At the beginning of the experiment, the average content of Mg extracted with the Mehlich III solution was 64 mg kg−1 in soils of both organic treatments ((a)). For ORG treatment this level stayed unchanged up to 2012 but in the following years showed a light increasing tendency. For ORGFYM and CONFYM treatments an increasing tendency of MgMehlich III from the beginning of the experiment was shown. In 2014, the average contents of MgMehlich III in soils of ORG, ORGFYM and CONFYM treatments increased by 12, 35 and 30 mg kg−1, respectively, as compared with their initial contents in 2008.
The same soil samples were tested also for ammonium acetate extractable magnesium (MgNH4OAc) and calcium (CaNH4OAc). In 2008 the average contents of MgNH4OAc in the soils of ORG and ORGFYM treatments were 33 and 35 mg/kg, respectively ((b)). During seven years the content of MgNH4OAc in the soil of ORG treatment remained rather stable regardless 12.9 kg ha−1 of Mg, as the average for 5-field rotation, was removed with crop yields. Although Mg like Ca is relatively easily leached, the soils developed on Mg-rich rocks are well supplied with Mg (Mengel & Kirkby Citation1987). It must be mentioned that the parent material of soil that covered the area of our experiment was dolomitic moraine in which Mg content is high.
For ORGFYM and CONFYM treatments, the MgNH4OAc contents of soil were more or less stable in the first four-five years but after this they increased. In 2014, the average contents of MgNH4OAc in soils of ORGFYM and CONFYM treatments increased by about 13–14 mg kg−1, compared with their initial contents in 2008. For both these treatments for 5-year period was given into soil 60 kg ha−1 of total Mg with cattle manure. According to Kulhánek et al. (Citation2014) FYM is a good source of bioavailable magnesium. In the conditions of our experiment, also the demineralization of natural Mg-bearing compounds of soil, in all likelihood, played a role in maintaining the stable to lightly increasing level of soil MgNH4OAc. Incidentally, according to our calculations on the basis of annual yields of crops cultivated and their Mg contents, the removal of Mg with yields during 5-year crop rotation was quite small – about 16 kg ha−1 for ORGFYM treatment and 28 kg ha−1 for CONFYM treatment.
Depending on soil peculiarities of the experiment area, the soil calcium contents of the crop rotation fields were quite variable at the beginning of the research. In 2008, the CaMehlich III content in soil samples varied from 1050 up to 1,380 mg kg−1, and the CaNH4OAc content from 579 up to 726 mg kg−1. For that reason the five-field average Ca values of the treatments showed quite high standard deviations ((a) and 1(b)). The average content of CaMehlich III in the soil of ORG treatment was practically unchanged during the research period. In ORGFYM treatment, the CaMehlich III content showed fluctuating tendency during the research period but their values in 2008 and 2014 were on the same level (1220 mg kg−1). Unfortunately, we cannot make reliable conclusions concerning CaMehlich III dynamics in the soils of the treatments. It must be mentioned that according to Schroder et al. (Citation2009) the results of Ca determined from soils by using the strongest extractant of Mehlich III are often not repeatable. Also according to Kulhánek et al. (Citation2014) it was not possible to deduce the tendency in soil calcium content changes during the experiment.
The annual 5-field average values of soil CaNH4OAc content were lightly fluctuating and showed no clear tendencies for all three treatments ((b)). However, in the last two years of our experiment a marginal rise in the soil CaNH4OAc of ORGFYM and CONFYM treatments was shown. This might have been caused by the application of FYM wherewith considerable amounts of calcium were given to the soil. But generally, it may be concluded that in the conditions of our experiment the soil CaNH4OAc concentrations did not decrease after seven years of different managements. In such soil the leaching of Ca by precipitation probably was counterbalanced with naturally released Ca from soil reserves and with Ca from FYM applied for two treatments.
Comparing the results of different analysis methods
As can be seen from , application of Mehlich III and alternative soil extraction methods yields in very different values by the size. The comparability of results obtained by different analysis methods demands recalculations which quality depends on the strength of correlative relationships.
The Pearson correlation coefficients between the soil extraction methods are presented in . The Pearson correlation coefficients between Mehlich III and AL methods for P (r = 0.770, p < .01) were lower than for K (r = 0.922, p < .01). The highest correlation between Mehlich III and NH4OAc-methods was found in case of Mg (r = 0.951, p < .01) whereat for Ca the Pearson correlation was somewhat weaker (r = 0.841, p < .01). Also Staugaitis and Rutkauskiené (Citation2010) have found that the correlations between the magnesium contents extracted with the Mehlich III solution and with ammonium acetate solution (NH4OAc-method) are very strong – 0.96–0.99.
Table 4. The Pearson correlation between the Mehlich III and the AL methods (P, K) and between the Mehlich III and the NH4OAc-methods (Mg, Ca).
As can be seen from , the Pearson correlation coefficients between Mehlich III and AL methods for P and also for K differed largely depending on the treatments. The lowest values of the correlation coefficients appeared for ORG treatment. The reasons behind these fluctuating coefficients need further investigations and explanations.
In addition, we decided also to investigate if there was any kind of relationship between the results of soil P and Ca contents. As mentioned by Wünscher et al. (Citation2015), the values of PMehlich III were negatively correlated with carbonate content and pH because CaCO3 may bind readily available P in Ca-phosphates, thereby fixing P in the soil. In our study, for every soil sample (n = 105) analyzed in parallel by means of Mehlich III and AL methods, the PMehlich III/PAL quotient was calculated and the results were collected in the groups according to the CaMehlich III value of the same soil samples. It is interesting to note that the PMehlich III/PAL quotient was inversely proportional to the CaMehlich III values, being 2.96 ± 0.28, 2.79 ± 0.32 and 2.54 ± 0.28 as the means for the following sets of CaMehlich III values, respectively: < 1100 (n = 34), 1101–1200 (n = 31), and >1201 (n = 40) mg Ca kg−1 dry soil.
In conclusion, the research questions which were set up at the start of the present work are answered in the following results. During the 7-year period, organic farming without nutrients’ input does not deplete the reserves of sandy loam soil in the organic carbon and available phosphorus but decreased the content of available potassium. The application of farmyard cattle manure at the rate of 60 t ha−1 during the 5-year crop rotation sustained the soil fertility status. Comparison of the soil analysis results performed by the Mehlich III method with the results obtained by the alternative extraction methods showed that the correlations for phosphorus and calcium are weaker than for potassium and magnesium.
Acknowledgements
The authors would like to thank Miralda Paivel, Mai Olesk, Liina Edesi and Jüri Kadaja for their help.
Disclosure statement
No potential conflict of interest was reported by the authors.
Additional information
Funding
References
- Andrist-Rangel Y, Edwards AC, Hillier S, Öborn I. 2007. Long-term K dynamics in organic and conventional mixed cropping systems as related to management and soil properties. Agric Ecosyst Environ. 122:413–426. doi: 10.1016/j.agee.2007.02.007
- Bakken AK, Breland TA, Haraldsen TK, Aamlid TS, Sveinstrup TR. 2006. Soil fertility in three cropping systems after conversion from conventional to organic farming. Acta Agric Scand Sect B Soil Plant Sci. 56:81–90.
- Bučiené A, Šlepetiené A, Šimanskaité D, Svirskiené A, Butkuté B. 2003. Changes in soil properties under high- and low-input cropping systems in Lithuania. Soil Use Manage. 19:291–297. doi: 10.1079/SUM2003209
- Edesi L, Järvan M, Noormets M, Lauringson E, Adamson A, Akk E. 2012. The importance of solid cattle manure application on soil microorganisms in organic and conventional cultivation. Acta Agric Scand Sect B Soil Plant Sci. 62:583–594.
- Egner H, Riehm H, Domingo WR. 1960. Untersuchungen über die chemische Bodenanalyse als Grundlage für die Beurteilung des Nährstoffzustandes der Boden. II.Chemische Extraktionsmethoden zur Phosphor- und Kaliumbestimmung. Ref.: Kungliga Lantbrucksstyrelsens Kungorelser M.M., Nr. 1, 1965. Stockholm.
- Ellmer F, Baumecker M. 2005. Static nutrient depletion experiment Thyrow. Results after 65 experimental years. Arch Agron Soil Sci. 51:151–161. doi: 10.1080/03650340400026669
- Eriksson AK. 2009. Phosphorus in agricultural soils around the Baltic Sea – comparisons of different laboratory methods as indices for phosphorus leaching to waters. Seminarier och examensarbeten Nr. 64. Uppsala: Swedish University of Agricultural Sciences.
- Fageria NK. 2012. Role of soil organic matter in maintaining sustainability of cropping systems. Commun Soil Sci Plan. 43:2063–2113. doi: 10.1080/00103624.2012.697234
- Feiziene D, Feiza V, Povilaitis V, Putramentaite A, Janušauskaite D, Seibutis V, Slepetys J. 2016. Soil sustainability changes in organic crop rotations with diverse crop species and the share of legumes. Acta Agric Scand Sect B Soil Plant Sci. 66:36–51.
- Gosling P, Shepherd M. 2005. Long-term changes in soil fertility in organic arable farming systems in England, with particular reference to phosphorus and potassium. Agric Ecosyst Environ. 105:425–432. doi: 10.1016/j.agee.2004.03.007
- Järvan M, Edesi L. 2015. Nitrogen cycle bacteria in soils of organically and conventionally managed crop rotations. Zemdirbysté/Agriculture. 102:15–22.
- Järvan M, Edesi L, Adamson A, Võsa T. 2014. Soil microbial communities and dehydrogenase activity depending on farming systems. Plant, Soil Environ. 60:459–463.
- Järvan M, Järvan U. 2010. Liming of soils. Saku: AS Rebellis; p. 115 . (in Estonian).
- Kauer K, Tein B, Talgre L, Eremeev V, Luik A. 2015. Estimation of soil carbon dynamics and its dependence on the farming system. Agronomy 2015, Tartu; p. 16–21 (in Estonian, with English abstract).
- Körschens M, Weigel A, Schulz E. 1998. Turnover of soil organic matter (SOM) and long-term balances. Tools for evaluating sustainable productivity of soils. J Plant Nutr Soil Sci. 161:409–424.
- Kulhánek M, Balík J, Černý F, Vašák F, Shejbalová Š. 2014. Influence of long-term fertilizer application on changes of the content of Mehlich-3 estimated soil macronutrients. Plant, Soil, Environ. 60:151–157.
- Leifeld J, Fuhrer J. 2010. Organic farming and soil carbon sequestration: what do we really know about the benefits? Ambio. 39:585–599. doi: 10.1007/s13280-010-0082-8
- Li X, Chang SX, Salifu KF. 2014. Soil texture and layering effects on water and salt dynamics in the presence of a water table: a review. Environ Rev. 22:41–50. doi: 10.1139/er-2013-0035
- Løes A-K, Øgaard AF. 2003. Concentrations of soil potassium after long-term organic dairy production. Int J Agr Sustain. 1:14–29. doi: 10.3763/ijas.2003.0103
- Loide V, Nõges M, Rebane J. 2004. Assessment of the fertilizer requirement using the extraction solution Mehlich 3. Agraarteadus/J Agr Sci. XV:206–215. (in Estonian, with English summary)
- Losak T, Hlusek J, Valka T, Elbl J, Vitez T, Belikova H, Von Bennewitz E. 2016. The effect of fertilization with digestate on kohlrabi yields and quality. Plant, Soil Environ. 62:274–278. doi: 10.17221/16/2016-PSE
- Masilionyté L, Maikšténiené S. 2016. The effect of alternative cropping systems on the changes of the main nutritional elements in the soil. Zemdirbyste-Agriculture. 103:3–10. doi: 10.13080/z-a.2016.103.001
- Matula J. 2010. Differences in available phosphorus evaluated by soil tests in relation to detection by colorimetric and ICP-AES techniques. Plant, Soil Environ. 56:297–304. doi: 10.1111/j.1747-0765.2010.00454.x
- Meena VS, Maurya BR, Verma JP. 2014. Does a rhizospheric microorganism enhance K+ availability in agricultural soils? Microbiol Res. 169:337–347. doi: 10.1016/j.micres.2013.09.003
- Mehlich A. 1984. Mehlich-3 soil test extractant: a modification of Mehlich-2 extractant. Commun Soil Sci Plan. 15:1409–1416. doi: 10.1080/00103628409367568
- Mengel K, Kirkby EA. 1987. Principles of plant nutrition. 4th ed. Bern: International Potash Institute.
- Methods of soil analysis. Part 2-chemical and microbiological properties. 1982. 2nd ed. Page AL, Miller RH, Keeney DR, editors. Madison, WI: American Society of Agronomy, Inc. & Soil Science Society of America, Inc.; p. 1159.
- Monokrousos N, Papatheodorou EM, Diamantopoulos JD, Stamou GP. 2006. Soil quality variables in organically and conventionally cultivated field sites. Soil Biol Biochem. 38:1282–1289. doi: 10.1016/j.soilbio.2005.09.023
- Otabbong E, Börling K, Kätterer T, Mattson L. 2009. Compatibility of the ammonium lactate (AL) and sodium bicarbonate (Olsen) methods for determining available phosphorus in Swedish soils. Acta Agric Scand Sect B Soil Plant Sci. 59:373–378.
- Parham JA, Deng SP, Raun WR, Johnson GV. 2002. Long-term cattle manure application in soil. I. Effect on soil phosphorus levels, microbial biomass C, and dehydrogenase and phosphatase activities. Biol Fert Soils. 35:328–337. doi: 10.1007/s00374-002-0476-2
- Parmar P, Sindhu SS. 2013. Potassium solubilization by rhizosphere bacteria: influence of nutritional and environmental conditions. J Microbiol Res. 3:25–31.
- Pikuła D, Rutkowska A. 2014. Effect of leguminous crop and fertilization on soil organic carbon in 30-years field experiment. Plant, Soil, Environ. 60:507–511.
- Pospisilova L, Formanek P, Liptaj T, Losak T, Martensson A. 2011. Land use effects on carbon quality and soil biological properties in Eutric Cambisol. Acta Agric Scand Sect B Soil Plant Sci. 61:661–669.
- Pospisilova L, Kominkova M, Zitka O, Kizek R, Barancikova G, Litavec T, Losak T, Hlusek J, Martensson A, Liptaj T. 2015. Fate of humic acids isolated from natural humic substances. Acta Agric Scand Sect B Soil Plant Sci. 65:517–528.
- Ram H, Malik SS, Dhaliwal SS, Kumar B, Singh, Y. 2015. Growth and productivity of wheat affected by phosphorus-solubilizing fungi and phosphorus levels. Plant, Soil Environ. 61:122–126. doi: 10.17221/982/2014-PSE
- Richardson AE, Simpson RJ. 2011. Soil microorganisms mediating phosphorus availability. Plant Physiol. 156:989–996. doi: 10.1104/pp.111.175448
- Roostalu H. 2014. Methods for fertilizer requirement of soils, interpretation and reliability of analyze results, and their use in agriculture; p. 57. (in Estonian) [Internet]; [cited 2016 Apr 18]. Available from: http://www.slideshare.net/meitjurgens/muldade-vetistarbe-meetodid…
- Sánchez de Cima D, Reintam E, Tein B, Eremeev V, Luik A. 2015. Soil nutrient evolution during the first rotation in organic and conventional farming systems. Commun Soil SciPlan. 46:2675–2687. doi: 10.1080/00103624.2015.1089268
- Sardi K, Füleky G. 2002. Comparison of extractants used for evaluating the bioavailability of soil P and K. Commun Soil Sci Plan. 33:2803–2812. doi: 10.1081/CSS-120014482
- Scherer HW, Metker DJ, Welp G. 2011. Effect of long-term organic amendments on chemical and microbial properties of a luvisol. Plant, Soil, Environ. 57:513–518.
- Schroder JL, Zhang H, Richards JR. 2009. Interlaboratory validation of the Mehlich 3 method as a universal extractant for plant nutrients. J AOAC Int. 92:995–1008.
- Šimon T, Czakό A. 2014. Influence of long-term application of organic and inorganic fertilizers on soil properties. Plant, Soil, Environ. 60:314–319. doi: 10.1080/00380768.2014.881706
- Staugaitis G, Rutkauskiené R. 2010. Comparison of magnesium determination methods as influenced by soil properties. Zemdirbysté/Agriculture. 97:105–116.
- Stockdale EA, Shepherd MA, Fortune S, Cuttle SP. 2002. Soil fertility in organic farming systems – fundamentally different? Soil Use Manage. 18:301–308. doi: 10.1079/SUM2002143
- Tabatabai MA. 1982. Soil enzymes. In: Page AL, Miller RH, Keeney DR, editors. Methods of soil analysis, Part 2. Madison (WI): American Society of Agronomy and Soil Science Society of America; p. 903–947.
- Talgre L, Lauringson E. 2015. Nutrient release during decomposition of green manure crops. Agronomy 2015, Tartu; p. 45–50 (in Estonian, with English abstract).
- Tõnutare T, Rodimas A, Kõlli R, Krebstein K, Penu P, Rebane J, Künnapas A. 2015. About determination of macroelements content in soil by Mehlich 3 method. Agronomy 2015, p. 219–224 (in Estonian, with English abstract).
- Wolinska A, Stepniewska Z. 2012. Dehydrogenase activity in the soil environment. In: Canuto RA, editor. Dehydrogenases, section 3, chapter 8. Rijeka; p. 183–210.
- Wünscher R, Unterfrauner H, Peticzka R, Zehetner F. 2015. A comparison of 14 soil phosphorus extraction methods applied to 50 agricultural soils from Central Europe. Plant, Soil, Environ. 61:86–96. doi: 10.17221/932/2014-PSE