ABSTRACT
In order to assess to what degree the ongoing global warming has affected flowering time of apple trees in the Nordic climate, we studied flowering phenology of trees in an orchard in Southern Norway. Correlation analysis of a 70-year (1946–2016) data series for flowering time of the cultivar ‘Gravenstein’ revealed a close relationship of the flowering phenophase with April–May heat accumulation (r = −0.98). Over the last 50-year period, flowering was advanced by 16 days in response to the temperature rises. Similarly, on average for 12 cultivars of varying earliness, flowering was advanced by 9 days over the latest 30-year period (1986–2016). Furthermore, the interrelationship between various phenological stages and floral morphogenesis of the same cultivars in the years 2013 and 2014 was studied, and related to flower-bud formation. The different stages of floral morphogenesis were identified, presented and used as a basis for assessment of flower-bud development in serial dissections. Floral initiation in spurs of actively growing trees took place in late July, approx. 8 weeks after full bloom, whereas in extension shoots, initiation took place after growth cessation in August, approximately 2 weeks later. Decreasing temperatures in mid-August coincided with the cessation of growth and floral initiation in extension shoots, suggesting that the processes may be causally related. The results show that, in the Nordic climate, the period between blooming and initiation of new floral primordia is considerably shorter than in warmer climates, suggesting that the period is only marginally long for completion of shoot growth, and hence, timely floral initiation. This may be an important reason for the irregular flowering and frequent incidences of alternate bearing in apple trees in the cool Nordic climate. The presented information can be useful for determination of the correct timing of flower and fruit thinning to counterbalance biennial bearing.
Introduction
Apple, a deciduous fruit species, is adapted to temperate regions in which they survive large seasonal changes in temperature. Long-term adaptation has resulted in an annual growth cycle in which all developmental processes in the tree are finely tuned with the annual course of growing conditions (Hänninen & Kramer Citation2007). This one-year cycle includes the processes of budburst, blooming (anthesis), extension growth, fruit set and development, cessation of growth, leaf senescence and abscission, as well as winter dormancy. During this cycle, the tree also shifts from vegetative to reproductive growth. Transition to reproductive growth comprises the processes of floral induction, initiation and differentiation of the different organs in the flower cluster (Tromp Citation2005b; Hanke et al. Citation2007; Dadpour et al. Citation2011). A manifold of internal and external cues are involved in the activation of floral induction genes, which leads to changes in the shoot apical meristem (SAM), the inception of floral primordia and later differentiation of floral organs (Buban & Faust Citation1982; Hanke et al. Citation2007).
Since flowers are a prerequisite for the formation of the crop, flower numbers and quality are major factors determining the potential yield in an orchard (Tromp et al. Citation2005b; Hanke et al. Citation2007). Instability of flowering from year to year is a worldwide problem in apple trees because of its relation to the phenomenon called alternate (biennial) bearing in pome fruits (Jonkers Citation1979). It is characterized by a biennial oscillation between large yields of under-sized fruit in ‘on-years’ and small yields of oversized fruit in ‘off-years’. The underlying cause is an adverse effect of endogenous hormones produced in the embryos of developing fruits during the period when flower induction should take place (Hanke et al. Citation2007 and ref. therein). The occurrence and severity of the biennial-bearing problem is particularly pronounced in Nordic climates with short growing seasons (Skogerbø Citation1987).
Despite the scientific and commercial importance of flower-bud formation (FBF) in apple, and despite extensive research efforts for more than a century, the environmental control of the process is still poorly understood. Our knowledge about the timing of FBF and its causal and temporal interrelations with other processes of the annual cycle of the tree, such as anthesis, extension growth, growth cessation and leaf abscission is also rather fragmented (Skogerbø Citation1987; Foster et al. Citation2003; Tromp Citation2005b; Koutinas et al. Citation2010). Also, most studies are conducted in countries where apple production is of high importance, but largely restricted to climates and cultivars of limited relevance to Nordic growing conditions. The complexity of the FBF process, its significance as a crucial step in the reproductive cycle of the tree and as a model for other woody species in the Rosaceae (Buban & Faust Citation1982; Kurokura et al. Citation2013), make it an important research objective. A better understanding of the processes would provide useful background for development of horticultural practices for improvement of flowering (e.g. fertilization, pruning, flower- and fruit thinning) and achievement of regular yields (Bangerth Citation2005). Furthermore, the impact of the predicted and ongoing global warming (Kaukoranta et al. Citation2010) on flowering and related processes in apple is of particular importance in the Nordic environment where the temperature is marginal for apple production and where the climatic changes will be the largest.
In the present investigation, we have analyzed two long-term series of flowering phenology of apples grown in an experimental orchard in southern Norway in relation to the ongoing climatic changes. We have also studied the onset and progress of FBF in 12 apple cultivars grown in the same orchard in one particular year and assessed the temporal relations to other developmental processes such as budburst, anthesis, extension growth, growth cessation and leaf abscission. The morphological changes occurring at the SAM during floral initiation and differentiation were identified and illustrated by means of scanning electron microscopy. The results of this part of the work were presented as an M.Sc. thesis by Rivero Casique (Citation2015).
Materials and methods
Plant material and growth site
Long- and short-term phenology of flowering (blooming) were studied in the experimental orchard at the Norwegian University of Life Sciences at Ås, Norway (59° 39′N, 10°47′E; 95 m a.s.l.). Long-term flowering data for the cultivar ‘Gravenstein’ were assembled over the 70-year period 1946–2016, as well as for this and 11 other cultivars over the 30-year period 1986–2016. In the year 2013, shoot growth and its temporal relationship to floral initiation and a range of other developmental processes were studied in detail in the same 12 cultivars. Scanning electron microscopy was employed to facilitate an accurate identification of the distinct stages of flower formation and differentiation. The following 12 apple cultivars (Malus domestica Borkh.) were studied: ‘Aroma’*, ‘Elstar’*, ‘Franskar’, ‘Gravenstein’*, ‘Julyred’, ‘Lobo’, ‘Mutsu’*, ‘Prins’, ‘Quinte’, ‘Summerred’*, ‘Sävstaholm’ and ‘Vista Bella’. Cultivars marked with an asterix (*) were grafted on M9 rootstock, while the rootstocks of the other cultivars are unknown.
Growth measurements, assessment of anthesis and leaf abscission
Early in the growing season, 10 current year’s shoots on one selected and representative tree of each cultivar were labeled on well-exposed, south-facing branches. Cumulative shoot growth and addition of new leaves were registered weekly on these shoots during the period 25 June–21 August 2013. For the long-term series and the spring observations in 2013 and 2014, the onset of the phenological stages of bud-break and flowering ‘green tip’ (GT), ‘pink’ (P) and ‘full bloom’ (FB) were registered based on the scale proposed by Chapman and Catlin (Citation1976). The dates on which more than 80% of the buds were in the same stage were recorded as FB. From 1 October until 25 November 2013, the progress of leaf abscission was followed, and visually estimated in percentage by weekly assessment of the total tree area covered by leaves.
Calculation of growing degree days (GDD)
In order to evaluate the relationship between developmental processes in the annual growth cycle of the tree and temperature, in terms of accumulation of heat, GDD were calculated by the following equation:where ‘Tmax’ and ‘Tmin’ are the mean daily maximum and minimum temperatures, respectively, for the studied period, and ‘Tbase’ is the threshold temperature below which the specific process studied does not progress (e.g. shoot growth, anthesis). The Tbase used was 4°C, and the accumulated GDD were calculated for the relevant time period. Temperature data were obtained from the Norwegian Meteorological Institute (Citation2016) (http://www.met.no/) and The Online Meteorological Service for Agriculture (Citation2016) (http://lmt.bioforsk.no/).
Scanning electron microscopy of the stages of floral morphogenesis
Terminal buds from spurs of ‘Summerred’ were fixed overnight in glutaraldehyde (1.25%) and para formaldehyde in 0.05 M PIPES buffer, pH 7.2, and subsequently kept in the same buffer at 4°C. Buds from a minimum of 20 shoots were sampled at regular intervals and dissected by removal of expanded leaves and bud scales. The dissected shoot apices were dehydrated in a series of ethanol solutions at 70, 90, 96 and 100%. Once dehydrated, the samples were dried in liquid CO2 using a critical point dryer (CPD 030, Bal-Tec, Balzers, Lichtenstein) mounted on stubs using double-faced carbon tabs (Agar Scientific, Essex, UK) and sputter coated with approximately 500 Å Pt in a SC7640 sputter coater (Quorum Technologies Ltd, Newhaven, UK). Specimens were examined in a Zeiss EVO-50 scanning electron microscope (Zeiss, Jena, Germany), operated at 20–25 kV and pictures of each stage of floral development were taken. Such stages were identified according to the scale proposed by Foster et al. (Citation2003) and used for scoring of stages in further dissections of the various cultivars.
Scoring of floral morphogenesis stages by serial dissections
Buds from spurs and extension shoots of the 12 cultivars were collected randomly at weekly intervals from well-exposed branches and then dissected under a binocular microscope (Wild Heerbrugg 50X, Switzerland). Expanded leaves and bud scales were removed, and the morphological stage of development of the shoot apex was assessed on the basis of the electron microscopy results and classified according to the scale proposed by Foster et al. (Citation2003).
The time of floral initiation in spurs was determined by weekly random sampling of five spurs from one selected and representative tree of each cultivar during the period 4 July–11 September 2013. Samples were fixed overnight in glutaraldehyde (1.25%) and paraformaldehyde (2%), and further kept in PIPES buffer 0.05 M at 4°C until dissection. In extension shoots, the time of floral initiation was assessed by weekly sampling of three shoots from one selected and representative tree of the cultivars ‘Aroma’, ‘Elstar’, ‘Franskar’, ‘Gravenstein’ and ‘Summerred’ during the period 9 August–26 September 2013.
Statistical analysis
Data were statistically analyzed using analysis of variance for the separation of means with a confidence interval of 95%. Grouping information was obtained by comparing means using Tukey’s method. To divide the multivariate dataset of measurements into groups of cultivars that shared similarities, a cluster analysis was performed using a hierarchical agglomerative clustering procedure with complete linkage and Euclidean distance. Due to large unit variations between vegetative parameters, the data were standardized by subtracting the means and dividing by the standard deviation before the distance matrix was calculated. Diagrams of relationships (dendrograms) are presented. Pearson correlation analysis was used to measure the strength of the relationship between the growth period vs. growth rate, and simple linear regression analysis was used for the parameters phenological stages vs. accumulated GDD. All analyses were performed using MiniTab® statistical software (17.1.0 MiniTab®, MiniTab Inc., PA, USA).
Results and discussion
Long-term flowering phenology
The 70-year April–May temperature series from Ås (1946–2016) comprises two distinct phases with contrasting trends (The Norwegian Meteorological Institute, Oslo). Up to the late 1960s, there was a moderate decreasing temperature trend, followed by a marked warming period lasting up to the present time. These temperature changes were clearly reflected in the flowering time of ‘Gravenstein’ apple trees with an early phase of delayed flowering culminating in 1966, and a subsequent phase with advanced flowering continuing up to the present time. During the decreasing temperature phase (1946–1966), FB of ‘Gravenstein’ apple trees was delayed by 13 days, while from the inflection point in 1966, flowering time was advanced by 16 days (). The same trends were observed for the P flowering stage (data not shown). A Pearson correlation analysis revealed a highly significant (P < .001) negative correlation between days to flowering (after April 1) and April–May heat accumulation (), indicating that temperature is the main causal factor behind the changes in flowering time. Inclusion of heat accumulation data for March slightly increased the correlation coefficient due to some very warm springs in the studied period (especially 1990).
Figure 1. Annual variation in the earliness of the occurrence of the spring phenology stage ‘full bloom’ for cultivar ‘Gravenstein’ at Ås, Norway, during the period 1946–2016. The regression lines for the two time phases (1946–1966 and 1966–2016) denote correlations between the number of days for the occurrence of full bloom and progression of time (calendar years). The parallel April–May heat sums are included for comparison.
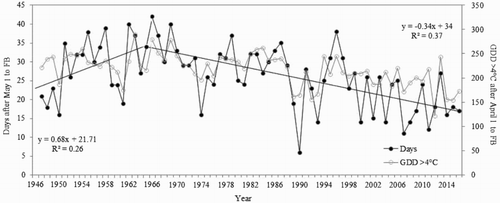
Figure 2. Regression analysis of the relationship between spring heat accumulation (GDD > 4°C for April and May) versus days after April 1 for the occurrence of full bloom stage (FB) in cultivar ‘Gravenstein’ for the 70 years’ period 1946–2016.
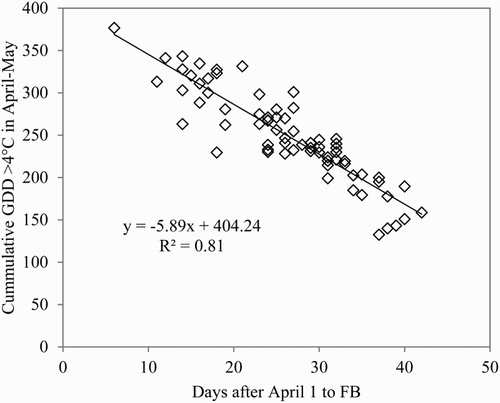
A parallel trend of advanced flowering was also found for the 12 cultivars observed over the 30-year period 1986–2016 (). On average for all cultivars, the FB stage was advanced by 9 days over this 30-year period, with a parallel trend for the P flowering stage (not shown for clarity reasons). The cultivar variation in earliness is shown in . Despite the variation in earliness, the temperature response was unanimous among the cultivars. These results are in close agreement with the trends for budburst and flowering reported by Nordli et al. (Citation2008) for apple and a range of other temperate zone trees in Norway.
Figure 3. Annual variation in the occurrence of the spring phenology stage ‘full bloom’ for 12 apple cultivars at Ås, Norway, during the period 1986–2016. Triangles and the connecting line denote mean number of days after 1 May, and small circles show the variation among the cultivars.
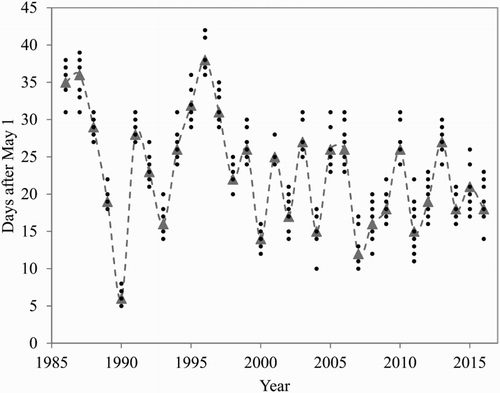
Table 1. Cultivar differences in the occurrence of the flowering phenology stages ‘pink’ and ‘full bloom’ among 12 apple cultivars at Ås, Norway, during the period 1986–2016.
Floral morphogenesis in apple as assessed by scanning electron microscopy
The morphological changes in the SAM during floral initiation and differentiation are illustrated in as an artificial denomination of concrete stages of the continuous process of floral morphogenesis. The doming of the SAM was considered the first visible sign of floral initiation (stage 2), since meristem diameter was not measured in this study (cf. Foster et al. Citation2003). With the latter method, the transition change can be detected a couple of days earlier, while the quality and reliability of the data are not improved. The seven morphological stages identified in the current study also closely agree with those reported by Foster et al. (Citation2003). Identification of stage 2 was based on the rounded, protuberant appearance of the SAM ((B)), and subsequent stages of development (3–7) were identified based on the order of appearance of floral meristems, bractlets and differentiation of sepals and petals ((C–H)). The last identified stage was the differentiation of sepals on all lateral flowers (stage 7, (G,H)).
Figure 4. Stages of floral morphogenesis in spurs of ‘Summerred’. (A) Narrow, flat apex (stage 1). (B) Domed apex with bract primordia (stage 2). (C) Inflorescence meristem and floral meristems with bracts (stage 3). (D) Terminal and lateral floral meristems with bractlets (stage 4). (E) Terminal and proximal floral meristems with sepals (stage 5). (F) Terminal floral meristem with initiated petals and lateral floral meristems with initiated sepals (stage 6). (G,H) All floral meristems with sepals (stage 7). LP, leaf primordium, rLP, removed LP, BP, bract primordium, rBP, removed BP, tB, terminal bract, FM, floral meristem, tFM, terminal FM, dFM, distal lateral FM, br, bractlet, S, sepal, tF, terminal (king) flower, Pe, petal.
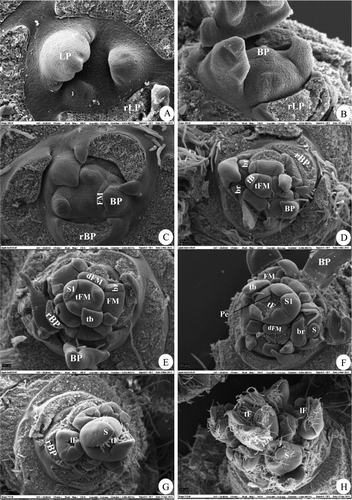
Floral morphogenesis
The average stage of development of the SAM in the 12 cultivars studied in 2013 increased with time in both spurs and extension shoots; the increment being nearly linear from the first sampling date until the maximum stage was reached ((A)). Floral initiation, as indicated by doming of the SAM (stage 2), was observed first in spurs in late July (on average 66 days after full bloom (DAFB), but not until August in extension shoots (on average 80 DAFB) (). Although of generally earlier appearance, these timing differences generally agree with those reported by Pogorelov (Citation1970) in Siberia, Buban and Faust (Citation1982) in Hungary, Tromp (Citation1984) in The Netherlands, and Hoover et al. (Citation2004) and Koutinas et al. (Citation2010) in New Zealand. The results in (B) and show considerable cultivar differences in the earliness of floral initiation. Spurs of ‘Julyred’, ‘Sävstaholm’, ‘Quinte’, ‘Lobo’ and ‘Summerred’ were particularly early, reaching stage 2 at 51–59 DAFB. ‘Gravenstein’, ‘Vista Bella’ and ‘Aroma’ reached stage 2 at 62–70 DAFB, while ‘Mutsu’, ‘Prins’, ‘Franskar’ and ‘Elstar’ were last, reaching stage 2 at 72–83 DAFB.
Figure 5. Time courses of floral morphogenesis for 12 apple cultivars in an experimental orchard at Ås, Norway, in the year 2013. (A) Total averages including all cultivars. (B) Individual cultivar curves for spurs. (C) Individual cultivar curves for extension shoots. For spurs, each data point represents the mean of five buds per cultivar; and for extension shoots, the mean of all buds of three shoots per cultivar.
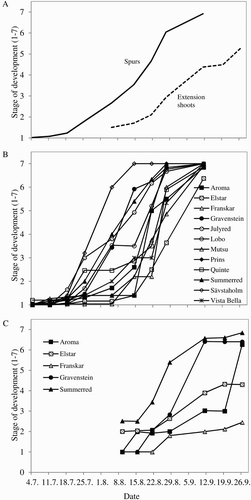
Table 2. Periods of floral development in spurs and extension shoots of 12 apple cultivars grown in an experimental orchard at Ås, Norway, in the year 2013.
Cultivars also differed in the length of the period of floral development (period between stages 2 and 7; Column ‘Diff. 2-7’ in ). In general, the elapsed time from meristem doming to fully developed sepals in all flower primordia (stage 7) was on average around 33 days for spurs and 45 days for extension shoots. In spurs, cultivar ‘Prins’ had the shortest period (11 days), ‘Elstar’, ‘Franskar’, ‘Sävstaholm’ and ‘Mutsu’ had a period of 23–28 days and for ‘Summerred’, ‘Aroma’, ‘Vista Bella’ and ‘Gravenstein’ the period lasted for 31–38 days. Cultivars ‘Lobo’, ‘Quinte’ and ‘Julyred’ had the longest periods of floral development in spurs (45–52 days). In extension shoots, floral development progressed faster in ‘Gravenstein’ (37 days between stages 2 and 7) than in ‘Summerred’ and ‘Aroma’. Cultivars ‘Franskar’ and ‘Elstar’ did not reach stage 7 by the last collection date in late September. No correlation was found between the earliness of floral initiation and the length of the period of floral development. Although not systematically investigated, we observed that the ability to form flower buds on extension shoots varied much among cultivars. In the cultivars ‘Discovery’, ‘Elstar’ and ‘Summerred’, this was commonly observed, while in cultivars such as ‘Aroma’ and ‘Franskar’ it was rarely seen.
On average, stage 7 was reached in spurs by late summer (98 DAFB), and in extension shoots by mid- autumn (123 DAFB). By mid-September, no domed meristems were observed in spurs, thus demonstrating that stages 2–6 are transient so that all domed meristems reached stage 7 by the end of the season. Similar results have been reported by Faust (Citation1989), Foster et al. (Citation2003) and Hoover et al. (Citation2004). In extension shoots on the other hand, stage 7 was not reached in all buds by the last sampling date ((C)); for instance, ‘Elstar’ and ‘Franskar’ reached only stages 4 and 2, respectively. These results agree with those reported by Costes (Citation2003), Faust (Citation1989), Jackson (Citation2003) and Zeller (Citation1960), and indicate that floral differentiation in extension shoots may extend throughout autumn and winter and possibly into the following spring, as long as temperatures are high enough to allow organogenic activity in the meristem.
Blooming, shoot growth and leaf abscission
Blooming. The progress of spring phenology in the years 2013 and 2014 (GT, P and FB) was positively correlated with spring heat accumulation, and quite representative for the studied 30-year period ((A)). This correlation is in accordance with the fact that the processes involved in bud development, that is, cell division and growth, are temperature dependent (Hänninen & Tanino Citation2011). Spring temperatures in 2014 were considerably higher than in 2013, resulting in higher accumulated GDD and earlier occurrence of GT ((B)). These temperature effects are in agreement with the results of Landsberg (Citation1979) and Jackson (Citation2003). Although temperatures from stage GT onwards were quite variable in both years, the period between GT and subsequent stages of bud growth was considerably longer in 2014.
Figure 6. Relations between progress of spring phenology stages (GT, P, FB) and April–May temperature for 12 apple cultivars in an experimental orchard at Ås, Norway, in the years 2013 and 2014. (A) Occurrence of the respective stages as related to April–May heat accumulation. Coefficient of determination (R²) for the linear regression is shown for each curve. (B) Daily mean temperatures during floral development to blooming. The occurrences of the respective stages are indicated for each curve. (C) Diagram of relationships between cultivars based on standardized GDD data for bud-break. Distance on the Y-axis shows the similarity level between clusters (0 = dissimilar, 100 = identical).
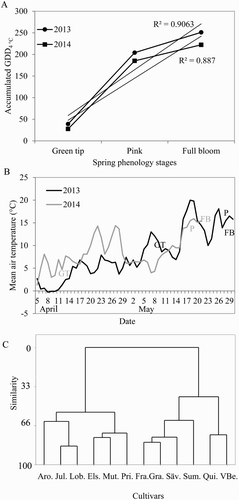
In general, the cultivar pattern of bud-break and flowering was similar in 2013 and 2014, that is, early cultivars being early in both years, and vice versa for late cultivars. However, the occurrence of the different phenological stages differed considerably between cultivars. Based on a cluster analysis of GDD data for each stage (), the cultivars studied were classified into early flowering: ‘Franskar’, ‘Gravenstein’, ‘Summerred’ and ‘Sävstaholm’; mid-season flowering: ‘Quinte’ and ‘Vista Bella’ and late flowering: ‘Aroma’, ‘Elstar’, ‘Julyred’, ‘Lobo’, ‘Mutsu’ and ‘Prins’ ((C)). Similar classifications have been proposed by Stedje and Skard (Citation1939) and Bø et al. (Citation1998) for ‘Franskar’, ‘Gravenstein’, ‘Lobo’, ‘Prins’, ‘Summerred’, ‘Sävstaholm’ and ‘Vista Bella’ in Norway, and Grauslund (Citation1996) for ‘Summerred’, ‘Gravenstein’, ‘Aroma’, ‘Mutsu’ and ‘Elstar’ in Denmark.
Table 3. Accumulated GDDT = 4°C (growing degree days at a base temperature of 4°C) at which the stages of bud-break green tip (GT), pink (P) and full bloom (FB) occurred in 12 apple cultivars in 2013 and 2014 in an experimental orchard at Ås, Norway.
Shoot growth. The progress of shoot growth followed a normal sigmoid pattern in all cultivars. Since shoot growth was recorded from FB onwards, only the linear part of the growth curve (period of active growth) and the plateau (time of growth cessation) are shown ((A)). The grand period of growth started right after FB and extended on average for 67 DAFB, at a rate of 5.8 cm/week and 1.8 leaves/week (). Considerable cultivar differences were observed, and these were due to longer internodes and not higher number of leaves. Shoot growth, in terms of total length and growth rate, was positively correlated with accumulated GDD ((B)).
Figure 7. Shoot growth in 12 apple cultivars grown in an experimental orchard at Ås, Norway, in the year 2013. (A) Growth curves for each of the cultivars. (B) Relation between shoot length and accumulated number of leaves versus summer heat accumulation. Coefficient of determination (R²) for the linear regression is shown for each curve.
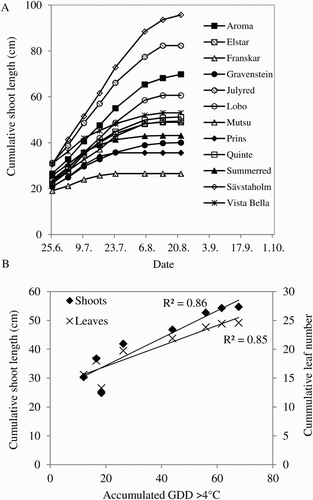
Table 4. Average growth rates, growth period (DAFB) to growth cessation and total shoot length for 12 apple cultivars grown in an experimental orchard at Ås, Norway, in 2013.
Leaf abscission. The onset of leaf abscission was earliest in ‘Julyred’, ‘Prins’, ‘Quinte’ and ‘Vista Bella’, cultivars which by October 1 had yellow leaves, and had shed about 20% of their leaves (data not shown). For the remaining cultivars leaf abscission was delayed to various degrees. For instance, the onset of leaf abscission in ‘Lobo’, ‘Mutsu’ and ‘Sävstaholm’ occurred by 10 October, and in ‘Franskar’, ‘Gravenstein’ and ‘Summerred’ by 24 October. For ‘Aroma’ and ‘Elstar’ the onset of leaf abscission occurred much later, about 30 calendar days after the first registration date. In late November, total leaf abscission was observed in most cultivars, except ‘Aroma’ and ‘Elstar’ which had shed only 40% of their leaves by that time.
Floral initiation and its relation to growth cessation, blooming and climate
In extension shoots, floral initiation was closely related to the date of growth cessation. On average for all cultivars, shoot growth stopped 67 DAFB, while floral initiation occurred around 80 DAFB, this representing a delay of about 2 weeks ( and ). This delay was considered by Tromp (Citation2005b) and Bangerth (Citation2005) as a consequence of the apical dominance of actively growing shoots, which prevents the outgrowth of lateral buds. Results in and show cultivar differences in the earliness of floral initiation and growth cessation, respectively. Extension shoots of ‘Summerred’, ‘Aroma’ and ‘Elstar’ stopped growth 60–77 DAFB, and became florally committed 71–84 DAFB. Extension shoots of ‘Gravenstein’ and ‘Franskar’ stopped growth 60–67 DAFB and became florally committed as late as 85–108 DAFB.
In spurs, the relationship between floral initiation and growth cessation (in the extension shoots) was not clear, and differed between cultivars. Domed meristems in spurs were observed before growth cessation in the cultivars ‘Aroma’, ‘Gravenstein’, ‘Julyred’, ‘Lobo’, ‘Quinte’ and ‘Sävstaholm’, close to growth cessation in ‘Summerred’, and after growth cessation in ‘Elstar’, ‘Franskar’, ‘Mutsu’, ‘Prins’ and ‘Vista Bella’. Since growth cessation is difficult to assess in spurs and was not determined in this study, our data did not allow any assessment of the relations between growth cessation and floral initiation in spurs. Nevertheless, studies by Fullford (Citation1966a, Citation1966b) showed that in spurs, the first sign of growth cessation is the formation of bud scales, which occurs before floral initiation. Likewise, Abbott (Citation1977) also reported that floral development in spurs takes place after growth has stopped and bud scales are formed. It, therefore, seems likely that also in spurs, the floral initiation is closely preceded by growth cessation in the spur itself.
Two aspects of climate, that is, temperature and precipitation, have been reported to influence the formation of floral buds in apple trees (Jackson & Sweet Citation1972; Buban & Faust Citation1982; Tromp Citation2005b). In the present study, no correlation was found between the proportion of floral buds and temperature or precipitation during the growing season in 2013. However, decreasing temperatures during mid-August coincided with the cessation of growth and floral initiation in extension shoots (), possibly due to the release from apical dominance. Low temperatures rather than short photoperiods were reported by Heide and Prestrud (Citation2005) to control growth cessation in apple trees. Possibly, there may be two different effects of temperature on FBF in apple extension shoots; one indirect effect of high temperatures through advancement of shoot growth and maturation, and another direct effect of low temperature on cessation of growth and floral initiation itself. The average daily temperature at which growth cessation and floral development occurred in spurs and extension shoots in 2013 was in the range of 15–20°C (). This is in agreement with the optimal range of 13–20°C reported by Tromp (Citation1984), Verheij (Citation1996) and Zhu et al. (Citation1997) for stimulation of FBF in apple trees under controlled conditions.
Figure 8. Relations between average daily mean temperatures during the growing season and the mean dates of occurrence of three critical developmental events in 12 apple cultivars grown at Ås, Norway, in the year 2013.
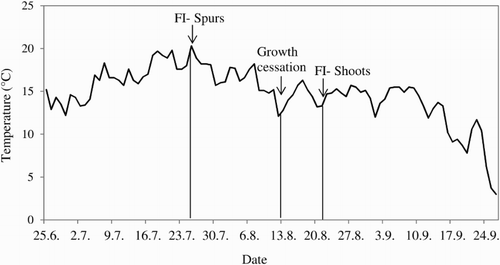
For some cultivars, timing of floral initiation was positively related to the earliness of bud-break and blooming. For instance, ‘Sävstaholm’ was one of the earliest cultivars to reach the stages of both bud-break and formation of flower buds in spurs (, (B)). Similarly, ‘Vista Bella’ was a mid-season bloomer and differentiated flowers in mid-season. ‘Elstar’ was late for both bud-break and floral initiation in spurs. It has been suggested that these relationships may be indirect and mediated by temperature, which affects the completion of pre-dormancy development, the time of growth cessation, the onset of dormancy and the subsequent emergence from dormancy (related to heat and chilling requirements) (Landsberg Citation1979; Heide & Prestrud Citation2005; Tromp Citation2005a). No clear relationship was found between the two processes in the remaining cultivars. The same lack of correspondence between the timing of floral initiation and blooming was reported by Hanke (Citation1981) and Hoover et al. (Citation2004).
In conclusion, the results of this investigation revealed a close positive correlation between spring temperature and earliness of flowering in apple, and hence, an advancement of flowering in the wake of the global warming. This is in general agreement with the results of a range of temperate zone trees (Nordli et al. Citation2008 and ref. therein). The present study also identified some interesting interrelations between floral development and blooming, growth and growth cessation of 12 apple cultivars adapted to the Nordic climate, which is characterized by a rather short growing season compared to other fruit-growing regions in the world. On average for these 12 cultivars, floral initiation in spurs took place about 66 DAFB. This is a considerably shorter time span than reported for apple cultivars in the USA (85–109 DAFB) (Hirst & Ferree Citation1995), New Zealand (76–127 DAFB) (Foster et al. Citation2003; Hoover et al. Citation2004) and Iran (90 DAFB) (Dadpour et al. Citation2011). These results show that in the Nordic climate, the period between blossoming and initiation of new floral primordia is much shorter than in warmer climates, suggesting that the period is marginally long for completion of shoot growth, and hence, timely floral initiation. This may be an important reason for the irregular flowering and frequent incidences of biennial bearing in apple in the cool Nordic climate. Observations over the last few decades also suggest a reduced frequency of biennial bearing in apple in Scandinavia in the wake of the ongoing global warming.
The presented information on the interrelations between the various phenological phases can also be useful for determination of the correct timing of horticultural practices applied for the achievement of good, stable crops, in particular flower and fruit thinning to counterbalance biennial bearing. Based on the results in this study, it seems that the critical time of fruit thinning would be different for the current year’s crop, and for FBF, which determines next year’s crop. For the former, fruit thinning can have a positive effect even late in the season, whereas the effect on FBF will be limited to an early period when seed development and gibberellic acid production takes place (cf. Forshey & Elfving Citation1989; Jackson Citation2003; Hanke et al. Citation2007). Of particular interest is the different timing of floral initiation in spurs and extension shoots, and the marked variation among cultivars in the ability to initiate flower buds on the current year’s extension shoots.
Acknowledgements
We thank Signe Hansen, Kari Grønnerød, and Elin Ørmen for excellent technical assistance.
Disclosure statement
No potential conflict of interest was reported by the authors.
Notes on contributors
Rodmar Rivero holds an MSc in Plant Science. He is currently a Research assistant at the Norwegian Institute of Bioeconomy Research, Norway, working with plant physiology in fruit and berries.
Anita Sønsteby is a Research Professor at the Department of Horticulture at Norwegian Institute of Bioeconomy Research, Norway. She has published numerous research articles on berries.
Ola M. Heide is Professor Emeritus of Plant Physiology at the Department of Ecology and Natural Resource Management, at the Norwegian University of Life Sciences, Ås, Norway. He has published numerous research articles on various aspects of plant development.
Finn Måge is Professor Emeritus of Horticulture at the Department of Plant Sciences, Norwegian University of Life Sciences, Ås, Norway. He has published numerous research articles on fruit and berries.
Siv Fagertun Remberg is an Associate Professor in Horticulture at the Department of Plant Sciences, Norwegian University of Life Sciences, Ås, Norway. She is teaching fruit and berry courses at the university, and has published numerous articles based on fruit and berry research.
Additional information
Funding
References
- Abbott D. 1977. Fruit bud formation in ‘Cox’s Orange Pippin’. Bristol: University of Bristol, Long Ashton Research Station.
- Bangerth F. 2005. Flower induction in perennial fruit trees: still an enigma? Acta Hortic. 727:177–196.
- Bø S, Lønø K, Skaarer N. 1998. Hageselskapets sortsliste. Oslo: Det norske hageselskap.
- Buban T, Faust M. 1982. Flower bud induction in apple trees: internal control and differentiation. Hortic Rev. 4:174–203. doi: 10.1007/978-1-349-06519-6_6
- Chapman P, Catlin G. 1976. Growth stages in fruit trees-from dormant to fruit set. New York Food Life Sci Bull. 58:1–11.
- Costes E. 2003. Winter bud content according to position in 3-year-old branching systems of ‘Granny Smith’ apple. Ann Bot. 92:581–588. doi: 10.1093/aob/mcg178
- Dadpour MR, Movafeghi A, Grigorian W, Omidi Y. 2011. Determination of floral initiation in Malus domestica: a novel morphogenetic approach. Biol Plantarum. 55:243–252. doi: 10.1007/s10535-011-0035-5
- Faust M. 1989. Physiology of temperate zone fruit trees. New York, NY: Wiley.
- Forshey CG, Elfving DC. 1989. The relationship between vegetative growth and fruiting in apple trees. Hortic Rev. 11:229–287.
- Foster T, Johnston R, Seleznyova A. 2003. A morphological and quantitative characterization of early floral development in apple (Malus × domestica Borkh.). Ann Bot. 92:199–206. doi: 10.1093/aob/mcg120
- Fulford R. 1966a. The morphogenesis of apple buds II. The development of the bud. Ann Bot. 30:25–38.
- Fulford R. 1966b. The morphogenesis of apple buds III. The inception of flowers. Ann Bot. 30:207–219.
- Grauslund J. 1996. Flowering dates of pome and stone fruit cultivars – 10 years results. Acta Hortic. 423:31–38. doi: 10.17660/ActaHortic.1996.423.3
- Hanke MV. 1981. Histologische Untersuchungen zur Blütenknospendifferenzierung bei Malus domestica Borkh. (‘Golden Delicious’) [Histological studies on the flower bud differentiation of Malus domestica Borkh. (‘Golden Delicious’)] [dissertation]. Berlin: Akademie der Landwirtschaftswissenschaften. German.
- Hanke MV, Flachowsky H, Peil A, Hättasch C. 2007. No flower no fruit – genetic potentials to trigger flowering in fruit trees. Genes Genomes Genomics. 1:1–20.
- Hänninen H, Kramer K. 2007. A framework for modelling the annual cycle of trees in boreal and temperate regions. Silva Fennica. 41:167.
- Hänninen H, Tanino K. 2011. Tree seasonality in a warming climate. Trends Plant Sci. 16:412–416. doi: 10.1016/j.tplants.2011.05.001
- Heide OM, Prestrud AK. 2005. Low temperature, but not photoperiod, controls growth cessation and dormancy induction and release in apple and pear. Tree Physiol. 25:109–114. doi: 10.1093/treephys/25.1.109
- Hirst PM, Ferree DC. 1995. Rootstock effects on shoot morphology and spur quality of ‘Delicious’ apple and relationships with precocity and productivity. J Am Soc Hortic Sci. 120:622–634.
- Hoover E, De Silva N, McArtney S, Hirst P. 2004. Bud development and floral morphogenesis in four apple cultivars. J Hortic Sci Biotec. 79:981–984. doi: 10.1080/14620316.2004.11511877
- Jackson DI, Sweet GB. 1972. Flower initiation in temperate woody plants. A review based largely on the literature of conifers and deciduous fruit trees. Hortic Abstr. 42:9–24.
- Jackson JE. 2003. The biology of apples and pears. New York, NY: Cambridge University Press.
- Jonkers, H. 1979. Biennial bearing in apple and pear: a literature survey. Sci Hortic. 11:303–317. doi: 10.1016/0304-4238(79)90015-3
- Kaukoranta T, Tahvonen R, Ylamaki A. 2010. Climatic potential and risks for apple growing by 2040. Agric Food Sci. 19:144–159. doi: 10.2137/145960610791542352
- Koutinas N, Pepelyankov G, Lichev V. 2010. Flower induction and flower bud development in apple and sweet cherry. Biotechnol Biotec Eq. 24:1549–1558. doi: 10.2478/V10133-010-0003-9
- Kurokura T, Mimida M, Battey NH, Hytönen T. 2013. The regulation of seasonal flowering in the Rosaceae. J Exp Bot. 64:4131–4141. doi: 10.1093/jxb/ert233
- Landsberg J. 1979. From bud to bursting blossom: weather and the apple crop. Weather. 34:394–407. doi: 10.1002/j.1477-8696.1979.tb03382.x
- Nordli Ø, Wielgolaski FE, Bakken AK, Hjeltnes SH, Måge F, Sivle A, Skre O. 2008. Regional trends for bud burst and flowering of woody plants in Norway as related to climatic change. Int J Biometeor. 52:625–639. doi: 10.1007/s00484-008-0156-5
- Norwegian Meteorological Institute [Internet]. 2016. Weather- and climate data from historical data. Oslo, Norway: Available from: http://www.met.no/.
- Online Meteorological Service for Agriculture [Internet]. 2016. Ås: Norwegian Institute of Bioeconomy Research (NIBIO). Available from: http://lmt.bioforsk.no/.
- Pogorelov P. 1970. Developmental cycle of apple flower buds. Sbornik Nauchnykh Rabot Sibirskii NII. 15:137–141.
- Rivero Casique R. 2015. Phenology and effect of climate on apple cultivars (Malus domestica Borkh.) in Norway [master thesis]. Norwegian University of Life Sciences. p. 79.
- Skogerbø G. 1987. Blomsterknoppdanning i frukttre med særlig vekt på eple [Flower-bud formation in fruit trees with special attention to apples] [dissertation]. Ås: Norges landbrukshøgskole. Norwegian.
- Stedje P, Skard OM. 1939. Norsk pomologi. Oslo: Gyldendal.
- Tromp J. 1984. Flower-bud formation in apple as affected by air and root temperature, air humidity, light intensity, and day length. Acta Hortic. 149:39–48. doi: 10.17660/ActaHortic.1984.149.3
- Tromp J. 2005a. Dormancy. In: Tromp J, Webster AD, Wertheim SJ, editors. Fundamentals of temperate zone tree fruit production. Leiden: Backhuys; p. 65–73.
- Tromp J. 2005b. Flower-bud formation. In: Tromp J, Webster AD, Wertheim SJ, editors. Fundamentals of temperate zone tree fruit production. Leiden: Backhuys; p. 204–215.
- Verheij FA. 1996. Morphological and physiological aspects of the early phases of flower bud formation of apple [dissertation]. Wageningen: Wageningen Agricultural University.
- Zeller O. 1960. Entwicklungsgeschichte der Blütenknospen und Fruchtanlagen an einjährigen Langtrieben von Apfelbüschen. [Development of flower buds and fruits in one-year old long shoots]. Z Pflanzenzucht. 44:175–214. German.
- Zhu LH, Borsboom O, Tromp J. 1997. The effect of temperature on flower-bud formation in apple including some morphological aspects. Sci Hortic. 70:1–8. doi: 10.1016/S0304-4238(97)00018-6