ABSTRACT
The genetic diversity available in sweetpotato has not been explored to develop dual-purpose sweetpotato varieties (DPSVs). The objectives of this study were to assess the level of phenotypic diversity present among sweetpotato varieties grown in Rwanda, and to select suitable parents for breeding DPSVs. Fifty-one diverse sweetpotato genotypes were evaluated in field trials conducted at the Rubona and Karama experimental stations of the Rwanda Agriculture Board using a 6 × 9 unbalanced alpha lattice design with three replications. Genotypes and sites showed significant interaction (P < .05), indicating differential response of genotypes in fresh root yield (FRY), root dry matter content, dry root yield (DRY), marketable root number, marketable root weight, flowering frequency and harvest index. The top two genotypes selected for their high FRY were RW11-4923 (20.9 t ha−1) and RW11-2419 (20.18 t ha−1). RW11-4923 and Wagabolige were the best performers for vine yield, producing 23.67 and 23.45 t ha−1 of vines, respectively. Ukerewe performed well for its DRY (7.09 t ha−1), while RW11-4923 had the highest mean dry vine yield (5.17 t ha−1). RW11-2910 and 8-1038 had root-to-vine ratios of 2.0 and 1.5, respectively, suggesting their suitability as breeding parents, to breed DPSVs. Two main phenotypic groups with 10 sub-groups were detected through cluster analysis. Principal component analysis showed that the first four components accounted for 76.33% of the phenotypic variation present among the 51 genotypes. The selected sweetpotato genotypes that had a combination of high storage root yields and heavy vine production should be used as parents in developing DPSVs, concurrently incorporating farmer-preferred traits.
Introduction
Sweetpotato [Ipomoea batatas (L.) Lam; 2n = 6× = 90] is an important root crop in most tropical and subtropical regions of the world. Its roots serves as a major source of human food, especially in sub-Saharan Africa (SSA) (FAOSTAT Citation2013), while the above-ground biomass is potentially useful for livestock feed. Sweetpotato storage roots contain carbohydrates and essential micronutrients such as vitamin A and minerals, particularly iron (Fe), zinc (Zn), calcium (Ca) and magnesium (Mg) (Tumwegamire et al. Citation2011). The orange-fleshed sweetpotato varieties (OFSPs) are currently being promoted for their high beta-carotene content, a precursor of vitamin A, which is useful in combating vitamin A malnutrition. Storage roots of the purple-fleshed sweetpotato varieties are rich in antioxidants and have been reported to prevent asthma, gout and arthritis in humans (Jata et al. Citation2011). Young and succulent leaves of some varieties of sweetpotato are consumed as cooked vegetables by rural communities in SSA. Leaves are believed to be higher in protein content and vitamins than storage roots (Antia et al. Citation2006). Sweetpotato storage roots can be consumed as boiled, pureed, fried or baked products (Jata et al. Citation2011). In Rwanda, sweetpotato puree and flour are used as a substitute for wheat flour to prepare various baked products (Sindi et al. Citation2013), hence saving on the country’s foreign exchange earnings. The baking industry in Rwanda is heavily dependent on imported wheat flour. Use of sweetpotato flour to prepare baked products presents potential advantages for farmers, processors and consumers owing to the high yields of the crop per unit area, reduced cost of crop production and final product costs (Sindi et al. Citation2013). The above-ground biomass of sweetpotato is widely used to feed livestock in a crop-livestock mixed farming system in countries such as Rwanda, where grazing lands are limited.
In the past, the emphasis in sweetpotato breeding was to develop varieties with high storage root yields or improved pro-vitamin A content for human food. However, some varieties have shown the potential to produce high storage root and above-ground forage yields for human food and livestock feed, respectively (Leon-Velarde et al. Citation1997). These types of sweetpotato are referred to as dual-purpose varieties (Leon-Velarde et al. Citation1997; León-Velarde Citation2000; Lukuyu et al. Citation2014). Selection of varieties for dual purpose is largely based on the relative proportion of root-to-forage dry matter production (León-Velarde Citation2000). Dual-purpose sweetpotato varieties (DPSVs) have been developed for their storage roots for human food and vines for livestock feed. This has great economic significance in a crop-livestock mixed farming system such as in Rwanda, where agricultural lands are limited. A good dual-purpose variety has the ability to regenerate quickly after sequential harvesting of a portion of the above-ground biomass, ensuing continued forage production before the final storage root is harvested. Sweetpotato leaves and vines are nutritious with 11% crude protein content and over 50% of organic matter digestibility (Mutimura et al. Citation2015). Due to the high nutritional value of the above-ground biomass of the crop, sweetpotato fodder-based diary production has been reported to increase mean milk yield by approximately 1.5 litres per day (Peters Citation2008). Therefore, there is a need to develop farmer-preferred DPSVs to enhance storage root and above-ground biomass yields in a crop-livestock mixed farming system.
In Rwanda, the availability of agricultural lands is steadily dwindling and yet the population is expected to double by 2030 (UNFPA Citation2007). To satisfy the needs for human food and livestock feed, more biomass production is required by using improved genotypes and exploiting the genetic potential of crops with multiple uses. Growing dual-purpose crop varieties would enable efficient uses of natural resources such as limited agricultural lands for sustainable crop production. Also farming systems which combine crop and livestock production are reported with improved livelihoods and resilience (Gibon et al. Citation2012).
Sweetpotato is an important crop in Rwanda and various breeding efforts of the crop have been reported (Ndirigwe et al. Citation2012; Rukundo et al. Citation2013; Shumbusha et al. Citation2014a).
The crop is widely grown by smallholder farmers using diverse genetic resources. The extent of genetic diversity of the crop grown by farmers and maintained in the Rwanda gene bank is not well explored. Using a set of 54 genotypes, Rukundo et al. (Citation2015) reported the existence of considerable genetic variation in the crop. The gene bank of the sweetpotato research programme of Rwanda Agriculture Board (RAB) maintains about 150 accessions, which include landraces, locally bred clones and introductions. The country has acquired a considerable number of sweetpotato varieties, mostly from various national agricultural research institutes (NARIs) and Consultative Groups on International Agricultural Research (CGIAR) centres such as from the International Potato Center (CIP) and the International Institute for Tropical Agriculture (IITA). The genetic diversity of sweetpotato has not been explored to develop DPSVs in Rwanda. Well-characterised germplasm is a pre-requisite in any crop improvement programme.
Several methods are available to characterise germplasm and select complementary parents for breeding (Huaman Citation1992). Agro-morphological characterisation has been widely and successfully used to determine the level of genetic diversity among sweetpotato genotypes (Tairo et al. Citation2008; Manamela Citation2009; Yada et al. Citation2010). In sweetpotato improvement, understanding the level of genetic diversity is useful to prevent genetic depression and to ensure sufficient genetic variation for selection. Therefore, the objectives of this study were to assess the level of phenotypic diversity present among sweetpotato varieties grown in Rwanda, and to use this information in order to select suitable parents for breeding dual-purpose varieties.
Material and methods
Germplasm
The study used 51 sweetpotato genotypes, of which 48 were sourced from the existing sweetpotato germplasm collection of RAB and 3 accessions were introductions from the CIP for this study. Fourteen genotypes () were previously evaluated for root and vine yields on a dry weight basis. Among the 14, six new farmer-preferred sweetpotato varieties, namely RW11-17, RW11-1860, RW11-2419, RW11-2560, RW11-2910 and RW11-4923, were released in 2013 to be used for both food and fodder production in Rwanda (Shumbusha et al. Citation2014a). Eight genotypes: Cacearpedo, SPK004, Kwezikumwe, Mugande, Wagabolige, 2002-154, 2002-155 and Naspot1 were included for their good root-to-vine ratio (RV) (Niyireba et al. Citation2013a) (). The remaining 31 genotypes were selected based on their diverse geographical origin and variation in morphological traits, including root skin and flesh colour. Two genotypes, namely Kemb 10 and Kemb 37 were previously reported to be high yielding in both root and vines in Kenya (Lukuyu et al. Citation2011). Six genotypes (Kyabafuriki 538, Melesiyana, Naspot 8, Naspot 9, New Kawogo and Seruruseke) were selected based on results of previous experiments indicating their drought tolerance (Rukundo Citation2015).
Table 1. Description of the 51 sweetpotato genotypes used in this study.
Study sites
The experiments were conducted at Rubona and Karama research stations of the RAB during March to July 2015. Both sites are characterised by clay and sandy soils (Rukundo et al. Citation2015) with distinct altitude and rainfall distribution. Rubona is located at a mid-altitude in Rwanda (1700 metres above sea level [m.a.s.l]); at 02° 29′S, 29° 46′E; with a mean temperature of 18.7°C, and a mean annual rainfall of 1200 mm. Karama (1400 m.a.s.l) is located in the lowlands of Rwanda (02° 17′S; 30° 16′E), with rainfall ranging between 700 and 900 mm, with a mean temperature of 20.8°C (Murayi et al. Citation1987). The Karama site is a known hot spot area for sweetpotato virus disease in Rwanda.
Experimental design and field management
Field experiments were conducted using a 6 × 9 unbalanced alpha lattice design with three replications at each site. Each entry in a replication was represented by a 3-row plot comprising 36 plants, with 12 plants per row. Intra-row spacing was 30 cm, while inter-row and inter-plot spacing were 80 and 90 cm, respectively. The sweetpotato variety Ukerewe was planted as guard rows in the trials. The plots were kept weed free until the ground was fully covered by the plants. Organic manure (30 t ha−1) was used once during planting, and no mineral fertiliser or pesticides were applied.
Data collection
Data were collected on both root and vine characteristics to select complementary clones. Root-related traits included marketable root number (MRN), marketable root weight (MRW), fresh root yield (FRY), root dry matter content (RDMC) and dry root yield (DRY). Vine-based traits are composed of fresh vine yield (FVY), vine dry matter content (VDMC), dry vine yield (DVY), virus score (VS) and flowering frequency (FF). The latter two vine traits were measured one month and three months after planting. FF was considered as the percentage of individual plants that flowered in a single experimental plot. Data on FRY and root yield-related traits such as MRN and MRW were recorded from 10 middle plants of each row, providing a plot mean data. A guard plant was left at either end of the row, giving a harvest plot area of 2.4 m2.
Data calculations
After harvest, root and vine yields (t ha−1) were determined on a dry weight basis, using the method described by Rodríguez (Citation1999), Benesi et al. (Citation2005) and Cervantes-Flores et al. (Citation2011). Two to three undamaged roots were randomly sampled for each genotype, sliced thinly and mixed together. Vines were also selected randomly from mature plants to form a representative sample for the plot. A root or vine sample of 300 g from the thin root slices or vine mixture was collected for dry matter determination. To obtain the dry weight, samples were oven-dried at 65°C for 72 hours to a constant weight. The dry matter content (DMC) was calculated as: DMC (%) = (dry weight/fresh weight) × 100. Dry yields were expressed as: [fresh yield × DMC]/100. The total biomass on dry weight basis (TBDW) was considered as the total of dry yields of roots and vines, whereas the harvest index (HI) was calculated as dry yield of roots divided by the TBDW. The RV was calculated according to Leon-Velarde et al. (Citation1997), and then classified into five groups: forage type (R:V of 0–1), low dual-purpose type (R:V > 1.0–1.5), high dual-purpose type (R:V > 1.5–2.0), low root production type (R:V > 2.0–3.0) and high root production type (R:V above 3.0). Combined root and vine parameters such as RV, TBDW and HI were calculated.
Data analysis
Quantitative data analysis was performed using the General Linear Models (GLM) procedure of the SAS statistical program (SAS Citation2008) to calculate analysis of variance (ANOVA). When significant treatment differences were detected by the ANOVA, then treatment means were separated using Fisher’s Protected Least Significance Difference test (LSD) at the 5% significance level. Qualitative data were analysed using the Statistical Package for Social Sciences (SPSS), 16th version. Multivariate analysis comprising cluster and principal component (PC) analyses were conducted. In order to determine the similarity and the level of genetic distance between genotypes, cluster analysis was performed using Euclidean distance, and a dendogram was generated using the nearest neighbour method. PC analysis was performed to determine the number of influential components and to calculate explained variation. Communalities indicating the amount of variance in each variable were calculated. Eigenvalues were calculated as the amount of variance in the original variables accounted for by each component. Correlations between the first two PCs and traits were performed. Finally, a genotype and genotype by environment (GGE) interaction biplot analysis was used to determine the amount of variation explained by the genotypic and site effect.
Results
ANOVA of root and vine parameters
The ANOVA for root, vine and root-vine combined parameters is summarised in . Sites had a highly significant (P < .01) effect on all root and combined root-vine parameters, and most of vine traits such as FVY, DVY, VS and FF. Non-significant differences were detected between sites for VDMC. Genotypes had highly significant (P < .01) differences for all the root and vine parameters, RV and HI and TBDW. Genotypes and sites interacted significantly (P < .05) for MRN, MRW, FRY, DRY, FF, RDMC and HI. There were non-significant interactions (P < .5) between genotypes and sites for vine parameters such as FVY, VDMC, DVY, VS and combined root-vine traits namely RV ratio and TBDW.
Table 2. ANOVA showing mean squares and significance tests of root, vine and root and vine parameters of 51 sweetpotato genotypes evaluated at Rubona and Karama sites of Rwanda during 2015.
Reponses of genotypes for root and vine parameters
Means of root and vine-related traits on fresh- and dry-weight basis for the 51 genotypes are presented in . FRYs ranged between 3.69 and 20.91 t ha−1, while FVY varied from 6.07 to 23.67 t ha−1. The best performing genotypes for FRY were RW11-4923 (20.91 t ha−1), followed by RW11-2419 (20.18 t ha−1), while for FVY RW11-4923 (23.67 t ha−1), followed by Wagabolige (23.45 t ha−1) were the best performers. The best performers for RDMC and VDMC were the genotypes Melesiyana and Kakamega-7, with 39.46% and 25.37%, respectively. Ukerewe had the highest DRY of 7.09 t ha−1 followed by RW11-4923 with 6.8 t ha−1. The top-performing two genotypes for DVY were RW11-4923 and NASPOT13, which produced 5.07 and 4.57 t ha−1, respectively. The top three genotypes with the highest FF were 2005-179 (23.0%), RW11-2910 (20.5%) and SPK004 (19.8%). Genotype Seruruseke had the highest RV of 3.73, while Mafuta performed relatively well, displaying the highest HI of 0.71.
Table 3. Mean root and vine-related traits on fresh- and dry-weight bases for 51 sweetpotato genotypes evaluated at Rubona and Karama sites of Rwanda in 2015.
Overall, test genotypes performed well at the Rubona than the Karama site. At Rubona site, higher mean values were recorded for FRY, RDMC, DRY, FVY, DVY, FF, RV and HI. However, genotypes scored the lowest VDMC at this site (21.69%) when compared to the Karama site (22.15%).
Clustering of sweetpotato genotypes using root and vine-related traits
The clustering of sweetpotato genotypes according to root and vine parameters is presented in . The cluster analysis grouped the 51 test genotypes into 2 major groups I and II, with 33 and 18 genotypes, respectively. The 2 clusters had a total of 10 sub-clusters. Cluster I consisted of four sub-clusters (namely I-a, I-b, I-c and I-d) with 19, 4, 2 and 8 genotypes, respectively. Cluster II had six sub-clusters (namely II-a, II-b, II-c, II-d, II-e and II-f) with 3, 1, 6, 3, 4 and 1 genotype, in that order (). Sub-clusters II-b and II-f may be considered ‘unique’ since each is composed of only one genotype, Wagabolige and Ukerewe, respectively. Except for the genotype Wagabolige, all other introduced genotypes such as Kemb 37 and Kemb 10 were clustered together (Cluster I, Sub-cluster I-a). With the exception of Naspot A and Naspot W6, all Naspot series such as Naspot 1, Naspot 8, Naspot 9 and Naspot 10 were grouped in Cluster I. Apart from genotype Melesiyana which appeared in Cluster I, Sub-cluster I-b, other landraces such as Seruruseke, Giteke and Hakizakubyara were grouped together in Sub-cluster I-d. The newly released varieties such as RW11-2419, RW11-17, RW11-2560, RW11-4923 and RW11-1860 were grouped together in Cluster I, with RW11-2560 and RW11-4923 forming their own sub-cluster, i.e. I-c. In Cluster II, genotypes of related serial number (namely 2005-179, 2005-062 and 2005-110) were found, reflecting their genetic relatedness or breeding history.
Figure 1. Dendogram showing 6 main clusters of 51 sweetpotato genotypes evaluated using root, vine and related parameters across 2 sites in Rwanda.
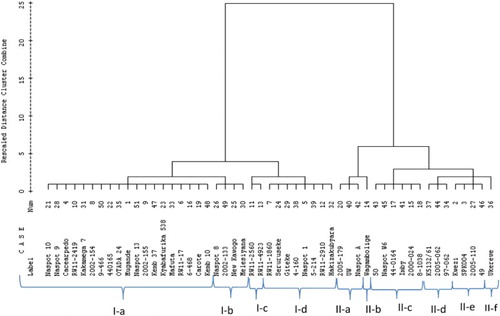
The cluster analysis allowed grouping of genotypes sharing common root characteristics. Consequently, the genotypes RW11-2419, Kakamega-7 and 2002-154 were grouped in Cluster I-a, associated with their high performance in FRY. The genotypes RW11-2910, 2000-024, Kyabafuriki 538, Seruruseke, Melesiyana, Otada 24 and 2002-133 were grouped in Custer I. These genotypes had high RDMC, which was above 37%. The genotypes RW11-4923, RW11-2419, Seruruseke, Kakamega-7 and Naspot 13 appeared in Cluster I, which could be explained by their high DRY above 6 t ha−1.
Further the genotypes were conveniently grouped using vine yield and related traits. The genotypes 2002-154, RW11-4923 and 9-466 were identified in Cluster I. These genotypes had a relatively high FVY of above 22 t ha−1. The genotypes Cacearpedo, Carote, Kakamega-7, Mafuta and Kemb 10 were grouped in Cluster I, Sub-cluster I-a, observed for their relatively high VDMC above 25%. The genotypes OTADA 24, Naspot 13, Kemb 10, 440165 and 2002-154 were identified in Cluster I, Sub-cluster I-a, which may have been due to their relatively high DVY.
Combined root and vine parameters may have influenced genotype grouping. The genotypes Kwezikumwe, 97-062, Imby and Naspot A were identified in Cluster II. These genotypes had a RV ranging between 1.5 and 2.0, a typical characteristic of genotypes with dual-purpose ability. Genotypes RW11-1860, Seruruseke, Mafuta, RW11-2419 and Naspot 9 were identified in Cluster I, with the last two grouped in Sub-cluster I-a. These five genotypes had a relatively high HI above 68%. The genotypes 2005-110, Naspot W6, and genotype denoted ‘50’ were grouped in Cluster II, with the last two identified in Sub-cluster II-c. These genotypes had a HI below 50%, characteristic of genotypes with a high yield of above-ground biomass. The genotypes Carote, Kemb 10, 2002-133, Giteke, OTADA 24 and 4-160 appeared in Cluster I. These genotypes had HI around 50%, characteristic of varieties with the ability to produce optimum yields of both roots and vines. The dissimilarity distance varied between 0% and 25% among the 51 genotypes evaluated in this study.
PC analysis
The first four components of the PC analysis explained 76.33% of the variation in root, vine and related parameters of the 51 test genotypes (). Only 23.67% of the total variation was un-explained in the original variables. Estimates of extraction communalities varied from 0.32 to 0.97, indicating that the extracted components were representative for all the variables.
Table 4. Eigenvectors and eigenvalues of the first 4 PCs of 13 storage root and vine-related parameters of 51 sweetpotato genotypes evaluated at the Rubona and Karama sites of Rwanda in 2015.
The first PC was highly correlated (0.96) with root-related traits, such as FRY and DRY. The second component was mainly correlated with vine-related traits such as FVY and DVY, with correlation values of 0.98 and 0.93, respectively. This component was inversely correlated with RV (−0.71). The third component was moderately correlated (0.89) with RDMC, while the fourth component was moderately correlated (0.74) with HI.
GGE interaction analysis
GGE interaction biplots of FRY are presented in . The first principal component (PC1) representing genotypic effect had a relatively high contribution (66.74%) to the total variance of FRY, while PC2 representing the test locations accounted for only 33.26% of the total variance. The genotypes RW11-4923, RW11-2419, Kakamega-7 and Ukerewe had relatively high PC1 values, with a high mean FRYs varying from 18.2 to 20.9 t ha−1. Conversely, the genotypes 2005-110, 6-468, 50, Hakizakubyara, Kemb 10 and 49 had high negative PC1 values, with low FRYs. Genotypes having PC2 scores near zero such as Hakizakubyara, Imby and Kyabafuriki were considered stable in terms of FRY.
Figure 2. GGE interaction bi-plot for FRY of 51 sweetpotato genotypes evaluated at the Karama and Rubona sites in 2016.
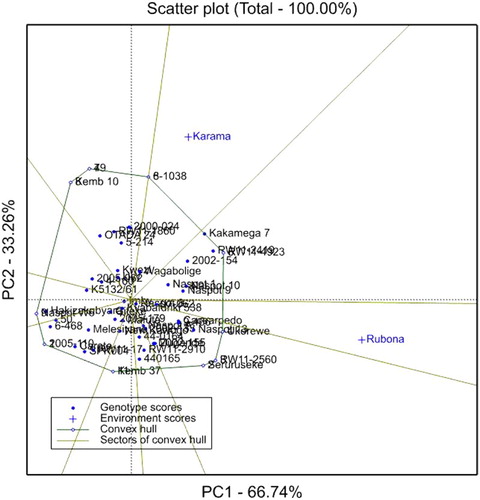
Both test environments (Rubona and Karama sites) had positive PC1 scores, with Rubona having a relatively high PC1 score. Rubona had a PC2 near zero, and therefore it was more representative of an average environment. Genotype RW11-2560 performed well at the Rubona site, whereas genotype 8-1038 was the best performer at the Karama site ().
The GGE biplots analysis of FVY is presented in . The PC1 representing genotypic effect showed a relatively high contribution (74.65%) to the total variance for FVY compared to PC2. The genotypes Wagabolige and RW11-4923 showed a relatively high PC1 values, with a high mean FVY of 23.45 and 23.67 t ha−1, respectively. In contrast, genotypes Kemb 37 and Mafuta had high negative PC1 values, with low FVYs of 8.39 and 6.07 t ha−1, in that order. The Rubona and Karama sites had positive PC1 scores. The Rubona site had a PC2 value relatively near zero, and was therefore more representative of an average environment. The genotype 9-466 was the best performer at Rubona, whereas genotype Kemb 10 performed best at the Karama site.
Discussion
Phenotypic diversity of sweetpotato genotypes for storage root yield and related traits
There were highly significant differences (P < .01) among 51 sweetpotato genotypes for most of storage root and vine-related parameters across 2 testing sites. Various authors reported the effect of test sites on storage root yields in sweetpotato (Grüneberg et al. Citation2005; Tumwegamire et al. Citation2011; Yada et al. Citation2011; Rukundo et al. Citation2015). Site differences could be attributed to variations in agro-ecological conditions characterising each site (Murayi et al. Citation1987; Rukundo et al. Citation2015). Genotypes and sites interacted significantly for MRN, MRW, FRY and DRY (). These results are consistent with previous studies (Adebola et al. Citation2013; Kivuva Citation2013; Lukuyu et al. Citation2014; Shumbusha et al. Citation2014b).
Marked differences in performance were detected among genotypes and across sites for their mean root and vine (). These findings were similar to those of Mwanga et al. (Citation2011). The FRY varied from 3.69 and 20.91 t ha−1, falling within the range reported previously by Gruneberg et al. (Citation2015) and Mwanga et al. (Citation2011). The wide range reflects the significant diversity of tested genotypes. Genotype RW11-4923 was the best performer for FRY (20.91 t ha−1) and the second in DRY (6.8 t ha−1) after Ukerewe (7.09 t ha−1). This was not surprising since the genotype RW11-4923 is among the newly released varieties with the ability to produce high biomass (Shumbusha et al. Citation2014a). The genotypes Melesiyana and Seruruseke had the highest relative RDMC of 39.46% and 38.89%, respectively (). These genotypes are landraces, and they have considerable genetic value for a breeding programme because they would incorporate farmer-preferred traits such as RDMC. DRY ranged between 1.45 and 7.09 t ha−1, which were higher values than those reported by Lukuyu et al. (Citation2014). Although the genotype RW11-2560 had a higher FRY (16.76 t ha−1) than OTADA 24 (11.97 t ha−1), the later exhibited a higher DRY value (4.68 t ha−1) than the former (4.3 t ha−1). This indicates that genotypes with low or moderate FRY values may still perform well in terms of DRY.
The Rubona site had higher overall mean values for FRY, RDMC and DRY than the Karama site (). The mean FRY at the Rubona site was 16.45 t ha−1, the double that at Karama site (8.12 t ha−1). The Karama site is prone to severe drought events, and high levels of sweetpotato virus incidence. Therefore, the two factors combined could probably inhibited storage root development, resulting in low crop yields. Rukundo et al. (Citation2015) reported relatively similar differences in site performance for root yield-related traits except for DRY.
Phenotypic diversity of sweetpotato genotypes for above-ground biomass and related traits
The test sites had a high significant effect (P < .01) on FVY, DVY, VS and FF, except VDMC (). These results partially disagree with Niyireba et al. (Citation2013b), who reported that VDMC varied between test locations. Genotypes differed significantly (P < .01) for FVY, DVY, VDMC, VS and FF. Acquaah (Citation2007) described the influence of genetic make-up to trait expression in different environments. A highly significant difference between sites and genotypes for vine-related traits was observed by previous authors (Larbi et al. Citation2007; Niyireba et al. Citation2013b). FVYs ranged between 6.07 and 23.67 t ha−1, while DVY varied between 1.34 and 5.07 t ha−1. These ranges were similar to those reported by Andrade et al. (Citation2010) and Lukuyu et al. (Citation2011). The top two genotypes that performed high in FVY were RW11-4923 (23.67 t ha−1) and Wagabolige (23.45 t ha−1). The former is among the newly released varieties in Rwanda producing high quantity of both root and vines (Shumbusha et al. Citation2014a), while the latter is reported ideal as a dual-purpose variety in Kenya (Lukuyu et al. Citation2011). The top three genotypes with the highest FF were 2005-179, RW11-2910 and SPK004, with 23.0%, 20.5% and 19.83%, respectively. Across sites, the mean FVY and DVY were higher at the Rubona site at 17.16 and 3.54 t ha−1 than at the Karama site with 12.91 and 2.74 t ha−1, in that order. The current results on FVY were contrary to those of Rukundo et al. (Citation2015), who reported different outcomes when evaluating another set of germplasm at the same sites.
Selection of sweetpotato genotypes with increased RV
Highly significant differences (P < .01) were detected among genotypes for RV, TBDW and HI (). A significant interaction effect (P < .05) was found between genotypes and sites for HI, which is a result similar to that of Gruneberg et al. (Citation2015). The genotypes RW11-2910 and 8-1038 had RV ratio values of 2.0 and 1.5, respectively, which are within the range of genotypes reported earlier to have dual-purpose ability (León-Velarde Citation2000). An increased HI was detected in the genotype Mafuta with 0.71, NASPOT9 at 0.69, and RW11-1860 and Seruruseke with HI values of 0.68. A high HI in sweetpotato indicates that storage roots constitute the main sink for photosynthesis (Bhagsari & Ashley Citation1990). The HI values ranged between 0.31 and 0.71, which were within the range reported by Bhagsari and Ashley (Citation1990). The site mean for HI was higher at Rubona at 0.61 than the mean HI achieved at Karama (0.47). Similarly, the mean RVs at Rubona and Karama were 2.0 and 1.23, respectively. These values were consistent with the findings of other authors (Niyireba et al. Citation2013a; Shumbusha et al. Citation2014a).
Genotypic diversity analysis classified the 51 genotypes into 2 major clusters of 33 and 18 genotypes, within which they were a further 10 sub-clusters (). The percentage dissimilarity varied from 0% to 25%, indicating a high level of phenotypic diversity between genotypes under study. Manamela (Citation2009), Tairo et al. (Citation2008) and Beah et al. (Citation2014) reported a relatively low distance coefficient ranging from 0.57 to 1.87, 0.0 to 0.52 and 0.6 to 1.0, respectively, indicating a low level of polymorphism in sweetpotato in their respective study environments.
In this study the first four PCs accounted for 76.33% of the total phenotypic variation (). The PC1 resulted in an eigenvalue of 4.65, contributing 35.81% of the total variability, whereas PC2 with eigenvalue of 2.91 accounted for 22.41% of the total observed variability. In a previous study by Koussao et al. (Citation2014), 4 PCs were detected explaining 67.22% of the variation measured, using 30 characters in sweetpotato. Afuape et al. (Citation2011) reported 3 PCs explaining 76% of the variability when testing 21 sweetpotato genotypes using 8 morphological characters, while Laurie et al. (Citation2013) reported a variation of 58% explained by 6 PCs in a study of 57 accessions, using 29 traits. In the present study the first component was mainly correlated with FRY and DRY with a correlation of 0.96. This indicates that the two traits contributed much (35.81%) of the observed variability in PC1. The PC2 was correlated with FVY and DVY with correlations of 0.98 and 0.93, respectively, suggesting that these two traits accounted for most of the 22.41% variability in PC2. This implies that, for breeding purposes, selection of parents with traits associated with high-storage root and vine yields in both fresh- and dry-weight bases would be important.
The results from the GGE analysis () revealed a large contribution of the PC1 to the total variance for FRY. Genotypes with relatively high positive PC1 values were RW11-4923, RW11-2419, Kakamega-7 and Ukerewe. Such genotypes were reported to be high yielders (Manrique & Hermann Citation2000). Accordingly, genotypes RW11-4923 and Wagabolige had a high above-average FVY in this study.
Marked phenotypic differences in the 51 sweetpotato genotypes evaluated for dual-purpose traits
Phenotypic differences were observed for storage root yield and related traits, above-ground biomass, RV, TBDW and HI. Genotypes RW11-4923, RW11-2419, Kakamega-7 and Ukerewe were the best performers for FRY, producing 20.91, 20.18, 20.08 and 18.25 t ha−1, respectively. Genotypes RW11-4923, Wagabolige, 9-466, 2002-154 and NASPOT13 performed well for FVY, producing 23.67, 23.45, 22.75, 2002-154 and 21.65 t ha−1, in that order. The outstanding performers for DRY were Ukerewe, Naspot 13, Seruruseke and Kakamega-7, which produced 7.09, 6.5, 6.14 and 6.13 t ha−1, respectively. Genotypes RW11-2560, Melesiyana and Kemb10 had relatively high DVY of 4.23, 4.12 and 4.02 t ha-1, in that order. Genotypes RW11-2910, NASPOT1 and 8-1038 had good RVs for dual-purpose applications, whereas Mafuta, RW11-1860 and the genotype ‘49’ had high HI values. Flowering ability varied between genotypes, and genotypes with a high FF were 2005-179, RW11-2910 and SPK004, with values of 23.0%, 20.5% and 19.83%, respectively. Using PC analysis, 2 major clusters, and 10 sub-clusters were detected through a genotypic diversity analysis of the tested germplasm. Out of the total phenotypic variation present among the 51 genotypes, PC analysis showed that 76.33% was due to the first four components. Genomic analysis needs to be performed with the selected set of genotypes using diagnostic molecular markers as a tool to identify superior parents for breeding DPSVs. The selected sweetpotato clones that consistently produced high yields of storage roots and vines may complement each other when used as parents, from which to develop DPSVs, while making sure to incorporate farmer-preferred traits.
Acknowledgements
The Rwanda Agriculture Board (RAB) is thanked for providing study leave for the first author. We are grateful to Drs Rukundo Placide and Walyaro Dick Jack for their assistance in data analysis and reading the manuscript.
Disclosure statement
No financial interest or any other benefit that was raised by the authors.
Notes on contributors
Damien Shumbusha is a plant breeder, Associate Research Fellow in Root and tubers programme at the Rwanda Agriculture Board (RAB). Since January 2014 to date, he is undertaking his Ph.D. studies in Plant Breeding at the University of KwaZulu-Natal, South Africa. In 2011, he graduated with MSc in Plant Breeding and Seed Systems from Makerere University, Uganda, a scholarship offered by the Alliance for a Green Revolution in Africa (AGRA). In 2005, he was awarded a BSc in Crop Production and Horticulture, University of Rwanda. He joined RAB (the former ISAR) in 2006, as a research assistant. After successfully completing his MSc studies, he re-joined his home institution, where he was leading sweetpotato research program, at the same time he was principal investigator of research projects such as 'Sweetpotato Action for Security and Health in Africa-Rwanda (SASHA)' and 'AGRA-Rwanda sweetpotato breeding project'. In 2013, he officially released six new high-yielding sweetpotato varieties namely RW11-17, RW11-1860, RW11-2419, RW11-2560, RW11-2910 and RW11-4923. He has published original papers in peer-reviewed journals. In 2014, he was awarded by AGRA a Ph.D. scholarship through the 'African Centre for Crop Improvement (ACCI)'. After successfully completing the Ph.D. course work, Damien was re-integrated in RAB, and he is currently conducting his Ph.D. research, while contributing to the day-to-day sweetpotato research program activities. While in RAB, Damien participated in various short-term trainings in Africa and Europe. He is a member of the African Potato Association (APA) since 2012.
Professor Hussein Shimelis (Ph.D.) is a Crop Scientist with a specialisation in Plant Breeding. He is Chair of Crop Science, Associate Professor of Plant Breeding and Deputy Director of the African Center for Crop Improvement (ACCI) at the University of KwaZulu-Natal, South Africa.
Professor Mark Laing (Ph.D.) is a Chair of Plant Pathology and Director of the African Center for Crop Improvement (ACCI) at the University of KwaZulu-Natal, South Africa.
Dr Theodore Asiimwe (Ph.D.) was born in Uganda on 23 December 1973. He attended school, up to university, in that country. He graduated with a BSc (Agriculture) from Makerere University in 2000. Dr Asiimwe later did his MSc (Plant Pathology) at Wageningen University, the Netherlands, from 2004 to 2006. He immediately competitively secured a Max Planck Society fellowship to conduct a Ph.D. (Plant Molecular Microbiology) at Max Planck Institute/Friedrich Schiller University, Germany, from 2006 to 2010. He also won a USDA fellowship to conduct postdoctoral research at Montana State University, USA (2011-2012). Since then, Dr Asiimwe has won a string of competitive grants and published a lot of scientific papers in peer-reviewed journals. Dr Asiimwe started working in Rwanda at the National Agricultural Research Institute, ISAR, in 2001, later joining other organizations to become RAB, steadily and rapidly going through research ranks up to his current rank of 'Senior Principal Research Fellow'. He is also the Head of Biotechnology Program at RAB. He chairs different committees, is member of different professional organizations and is reviewer of different high-quality journals.
ORCID
Damien Shumbusha http://orcid.org/0000-0001-9383-4685
Additional information
Funding
References
- Acquaah G. 2007. Principles of plant breeding and genetics. Malden(MA): Blackwell.
- Adebola P, Shegro A, Laurie S, Zulu L, Pillay M. 2013. Genotype x environment interaction and yield stability estimate of some sweetpotato [Ipomoea batatas (L.) Lam] breeding lines in South Africa. J Plant Breed Crop Sci. 5:182–186. doi: 10.5897/JPBCS2013.0387
- Afuape S, Okocha P, Njoku D. 2011. Multivariate assessment of the agromorphological variability and yield components among sweetpotato (Ipomoea batatas (L.) Lam) landraces. Afr J Plant Sci. 5:123–132.
- Andrade MI, Naico A, Ricardo J, Alvaro A, Moniz S, Sitoe E, Gruneberg W. 2010. Evaluation of 64 clones selected from advanced yield trials established between 2005/06 and 2009/10 in Maputo, Gaza, Zambezia and Tete. Maputo, Mozambique.
- Antia B, Akpan E, Okon P, Umoren I. 2006. Nutritive and anti-nutritive evaluation of sweetpotatoes (Ipomoea batatas) leaves. Pak J Nutr. 5:166–168. doi: 10.3923/pjn.2006.166.168
- Beah A, Samba J, Tucker M, Benya M, Fomba S. 2014. Agro-phenotypic characterization of sweetpotato (Ipomoea batatas L.) genotypes using factor and cluster analyses. Agric Sci Res J. 4:30–38.
- Benesi I, Labuschagne M, Dixon A, Mahungu N. 2005. Genotype x enviroment interaction effects on native cassava starch quality and potential for starch use in the commercial sector. Afr Crop Sci J. 12:205–216. doi:10.4314/acsj.v12i3.27880
- Bhagsari AS, Ashley DA. 1990. Relationship of photosynthesis and harvest index to sweetpotato yield. J Am Soc Hort Sci. 115:288–293.
- Cervantes-Flores J, Sosinski B, Pecota K, Mwanga R, Catignani G, Truong V-D, Watkins R, Ulmer M, Yencho GC. 2011. Identification of quantitative trait loci for dry-matter, starch, and β-carotene content in sweetpotato. Mol breed. 28:201–216. doi:10.1007/s11032-010-9474-5
- FAOSTAT. 2013. FAOSTAT statistical database. Rome: FAO.
- Gibon A, Ryschawy J, Schaller N, Fiorelli J, Havet A, Martel G. 2012. Why and how to analyse the potential of mixed crop-livestock farming systems for sustainable agricultural and rural development at landscape level. In: Proceedings of the 10th European IFSA Symposium, France. p. 9.
- Gruneberg WJ, Ma D, Mwanga ROM, Carey EE, Huamani K, Diaz F, Eyzaguirre R, Guaf E, Jusuf M, Karuniawan A, et al. 2015. Advances in sweetpotato breeding from 1993 to 2012. In: Low J, Nyongesa M, Quinn S, Parker M, editors. Potato and sweetpotato in Africa: transforming the value chains for food and nutrition security. Oxfordshire: CABI; p. 3–68.
- Grüneberg WJ, Manrique K, Zhang D, Hermann M. 2005. Genotype x environment interactions for a diverse set of sweetpotato clones evaluated across varying ecogeographic conditions in Peru. Crop Sci. 45:2160–2171. doi:10.2135/cropsci2003.0533
- Huaman Z. 1992. Systematic botany and morphology of the sweetpotato plant. Lima: CIP.
- Jata SK, Nedunchezhian M, Misra R. 2011. The triple ‘f’ (food, fodder and fuel) crop sweetpotato [Ipomoea batatas (L.) Lam.]. Orissa Rev. 5:82–92.
- Kivuva BM. 2013. Breeding sweetpotato (Ipomoea batatas [L.] Lam.) for drought tolerance in Kenya [PhD thesis]. Pietermaritzburg: University of KwaZulu-Natal.
- Koussao S, Gracen V, Asante I, Danquah EY, Ouedraogo JT, Baptiste TJ, Jerome B, Vianney TM. 2014. Diversity analysis of sweetpotato (Ipomoea batatas [L.] Lam) germplasm from Burkina Faso using morphological and simple sequence repeats markers. Afr J Biotechnol. 13:729–742. doi: 10.5897/AJB2013.13234
- Larbi A, Etela I, Nwokocha HN, Oji UI, Anyanwu NJ, Gbaraneh LD, Anioke SC, Balogun RO, Muhammad IR. 2007. Fodder and tuber yields, and fodder quality of sweetpotato cultivars at different maturity stages in the West African humid forest and savanna zones. Anim Feed Sci Technol. 135:126–138. doi:10.1016/j.anifeedsci.2006.05.021
- Laurie SM, Calitz FJ, Adebola PO, Lezar A. 2013. Characterization and evaluation of South African sweetpotato (Ipomoea batatas (L.) Lam) land races. S Afr J Bot. 85:10–16. doi:10.1016/j.sajb.2012.11.004
- León-Velarde C. 2000. Using competing traits to select dual-purpose sweetpotato in native germplasm. Lima: International Potato Centre (CIP).
- Leon-Velarde C, Roca J, Arteaga J, Quispe L, Parraga A. 1997. Perspectives on sweetpotato: dual-purpose varieties. Lima: International Potato Centre (CIP). ISSN 0256–6311.
- Lukuyu B, Kinyua J, Agili S, Gachuiri C, Low J. 2011. Evaluation of pre-screened sweetpotato germplasm for biomass production under different cropping regimes and their potential as dual-purpose varieties in Kenya. Nairobi: International Potato Centre (CIP).
- Lukuyu B, Kinyua J, Agili S, Gachuiri C, Low J. 2014. Evaluation of sweetpotato varieties for the potential of dual-purpose in different agroecological zones of Kenya. In: Vanlauwe B, VanAsten P, Blomme G, editors. Challenges and opportunities for agricultural intensification of the humid highland systems of sub-saharan Africa. Switzerland: Springer International; p. 217–231.
- Manamela MT. 2009. Morphological characterisation and cryopreservation of sweetpotato, Ipomoea batatas (L.) Lam., accessions at the NPGRC of South Africa [MSc thesis]. Uppsala, Sweden.
- Manrique K, Hermann M. 2000. Effect of GxE interaction on root yield and beta-carotene content of selected sweetpotato (Ipomoea batatas (L) Lam.) varieties and breeding clones. Lima: International Potato Centre. CIP program report 1999-2000.
- Murayi T, Sayers A, Wilson R. 1987. La Productivité des Petits Ruminants dans les Stations de Recherche de l’Institut des Sciences Agronomiques du Rwanda. Rapport de Recherche No. 15. Addis-Abeba: CIPEA. (9290530820).
- Mutimura M, Ebong C, Rao IM, Nsahlai IV. 2015. Nutritional values of available ruminant feed resources in smallholder dairy farms in Rwanda. Trop Anim Health Prod. 47:1131–1137. doi:10.1007/s11250-015-0839-y
- Mwanga RO, Niringiye C, Alajo A, Kigozi B, Namukula J, Mpembe I, Tumwegamire S, Gibson RW, Yencho GC. 2011. ‘NASPOT 11’, a sweetpotato cultivar bred by a participatory plant-breeding approach in Uganda. HortScience. 46:317–321.
- Ndirigwe J, Sindi K, Kankundiye L, Shumbusha D, Nshimiye J, Hakizimana S, Low J. 2012. Improving farmers’ access to clean planting material through partnership in the value chain. Kigali: International Potato Centre (CIP). CIP programme papers; [cited 2016 Sep 1]. Available from: https://cgspace.cgiar.org/handle/10568/66266.
- Niyireba RT, Ebong C, Agili S, Low J, Lukuyu B, Kirui J, Ndirigwe J, Uwimana G, Kankundiye L, Mutimura M, et al. 2013a. Evaluation of dual-purpose sweetpotato [Ipomoea batatas (L.) Lam] cultivars for root and fodder production in eastern province, Rwanda. Agric J. 8:242–247.
- Niyireba RT, Ebong C, Lukuyu B, Agili S, Low J, Gachuiri C. 2013b. Effects of location, genotype and ratooning on chemical composition of sweetpotato [Ipomea batatas (L.) Lam] vines and quality attributes of the roots. Agric J. 8:315–321.
- Peters D. 2008. Assessment of the potential of sweetpotato as livestock feed in East Africa Rwanda, Uganda, and Kenya. Nairobi: International Potato Centre (CIP).
- Rodríguez F. 1999. Methods to evaluate culinary quality and other attributes for processing sweetpotato storage roots. In: Huaman Z, editor. Sweetpotato (Ipomoea batatas) germplasm management training manual. Lima: CIP; p. 17.
- Rukundo P. 2015. Breeding of sweetpotato (Ipomoea batatas (L.) Lam.) for drought tolerance and high dry matter content in Rwanda [PhD thesis]. Pietermaritzburg: University of KwaZulu-Natal.
- Rukundo P, Shimelis H, Laing M, Gahakwa D. 2013. Storage root formation, dry matter synthesis, accumulation and genetics in sweetpotato. Aust J Crop Sci. 7:2054–2061.
- Rukundo P, Shimelis H, Laing M, Gahakwa D. 2015. Phenotypic characterisation of sweetpotato genotypes grown in East and Central Africa. S Afr J Plant Soil. 32: 1–10.
- SAS. 2008. SAS/STAT 9.2 user’s guide. Cary (NC): SAS Institute Inc.
- Shumbusha D, Ndirigwe J, Kankundiye L, Musabyemungu A, Gahakwa D, Ndayemeye PS, Mwanga ROM. 2014a. ‘RW11-17’, ‘RW11-1860’, ‘RW11-2419’, ‘RW11-2560’, ‘RW11-2910’, and ‘RW11-4923’ sweetpotato. HortScience. 49:1349–1352.
- Shumbusha D, Tusiime G, Edema R, Gibson P, Adipala E, Mwanga R. 2014b. Inheritance of root dry matter content in sweetpotato. Afr Crop Sci J. 22:69–78.
- Sindi K, Kirimi L, Low J. 2013. Can biofortified orange fleshed sweetpotato make commercially viable products and help in combatting Vitamin A deficiency? Conference paper presented in the 4th International Conference of the African Association of Agricultural Economists; 2013 Sep 22–25.
- Tairo F, Mneney E, Kullaya A. 2008. Morphological and agronomical characterization of sweetpotato [Ipomoea batatas (L.) Lam.] germplasm collection from Tanzania. Afr J Plant Sci. 2:77–85.
- Tumwegamire S, Kapinga R, Rubaihayo PR, LaBonte DR, Grüneberg WJ, Burgos G, zum Felde T, Carpio R, Pawelzik E, Mwanga RO. 2011. Evaluation of dry matter, protein, starch, sucrose, β-carotene, iron, zinc, calcium, and magnesium in East African sweetpotato [Ipomoea batatas (L.) Lam] germplasm. HortScience. 46:348–357.
- UNFPA. 2007. State of the world population: Unleashing the potential of urban growth. New York(NY): UNFPA.
- Yada B, Tukamuhabwa P, Alajo A, Mwanga R. 2010. Morphological characterization of Ugandan sweetpotato germplasm. Crop Sci. 50:2364–2371. doi:10.2135/cropsci2009.04.0199
- Yada B, Tukamuhabwa P, Alajo A, Mwanga R. 2011. Field evaluation of Ugandan sweetpotato germplasm for yield, dry matter and disease resistance. S Afr J Plant Soil. 28:142–146. doi:10.1080/02571862.2011.10640026