ABSTRACT
We evaluated phytoremediation potential of a calcareous saline-sodic soil with Prosopis glandulosa relying on either N2 fixation (NFIX) or inorganic N (IN) and gypsum. Straw and root dry matter of NFIX were 60% and 67% higher than that of IN, respectively. While both plants relying on IN or NFIX retained nearly similar Ca2+, Na+ level in IN lysimeters was 1.8 folds that of NFIX, whereas levels of Na+ and K+ in roots of NFIX were 13% and 24% higher than that of IN plants. P. glandulosa relying on NFIX and IN released 39% and 50% Na+ of that released by the gypsum. P. glandulosa produced more than the gypsum treatment and induced 24% of Ca2+ solubilization found in the gypsum treatment. Translocation factor of Na+ and the K+/Na+ ratio in NFIX and IN were 0.58, 0.99 and 13.2, 7.0, respectively. The practical implication of this study conclude that P. glandulosa grown with NFIX or IN is an alternative practice for amelioration of calcareous salt-affected soils.
Introduction
Soils with salts to levels that can adversely affect several of their properties as well as growth of most crops are termed salt-affected soils. The accumulation of salts can both adversely affect plants’ performance (Aslam et al. Citation2011) and the physico-chemical characteristics of the soil and thereby limiting its potential use (Qadir & Schubert Citation2002). The rate of salinization of soil and groundwater can be further accelerated with climate change and increased irrigation with brackish water because it leads to increased evaporation and promotes capillary rise of saline ground water (Jesus et al. Citation2015). In arid and semi-arid regions, spatial and temporal (1999–2000) changes in electrical conductivity (EC) and CI−1 were 16.8 µS cm−1 and 0.18 meq I−1 Y−1, respectively (Yazdanpanah Citation2016). In more than 100 countries worldwide, occurrence of salt-affected soils is estimated at 1–10 billion ha with a potential of 10–16% increase/year (Qadir & Oster Citation2002; Yensen & Biel Citation2006; Aydemir & Sünger Citation2011). In irrigated dry lands, processes such as salinization and sodication are major driving forces for desertification and thereby threatening the productive capacity of agricultural lands, forestlands and rangelands (Dregne et al. Citation1991; Mustafa Citation2007).
The native plants of Prosopis sp are adapted to the conditions of arid and semi-arid regions and are very important in controlling desertification through stabilization of sand dunes, improving soil fertility, reducing soil salinity, providing fuel energy resources, supplying feed and forage for grazing animals, furnishing construction timber, supplementing food for humans and promoting honey production (Manzano & Navar Citation2000). Prosopis was therefore introduced in Sudan in the year 1917 with the aim of combating desertification by stopping sheet sand encroachment on sandy soils (e.g. Salih Citation1998; Elfadl & Luukkanen Citation2003). Prosopis juliflora grows in saline soils with EC from 8 to 51 dS m−1 (Joshi & Hinglajia Citation2000) and in sodic soils at pH of 10 and exchangeable sodium percentage (ESP) of 60 in the rooting zone (Singh et al. Citation1998). However, some Prosopis sp such as Prosopis koelziana can tolerate salinity up to 185 dS m−1 (Emtahani & Elmi Citation2006).
The genus Prosopis contains many N2-fixing species throughout the world’s semi-arid regions. Previous work with Prosopis glandulosa found that small young trees obtained most of their N from N2-fixation (NFIX), while mature trees that had accumulated 1.3 Mg N ha−1 in the soil beneath their canopy derived a much smaller percentage of their N2 from NFIX. Prosopis was earlier said to be an active N2 fixing tree where N derived from fixation (%Ndfa) determined in wood trunk and leaves were 75% and 25%, respectively (Geesing et al. Citation2000). Due to the limited infiltration of arid and semi-arid soils, the need for organic amendment is necessary to improve soil hydraulic conductivity (De Boever et al. Citation2016), which is controlled by particle size distribution (Mazaheri & Mahmoodabadi Citation2012). This clearly showed that salinity not only negatively affects soil physico-chemical conditions but also microbial functional properties (Min et al. Citation2016). Although gypsum is superior in reclamation of sodic soils (Ahmed et al. Citation2015), the ability of organic amendments in depleting deleterious salts from soil have reported positive effects. For example, combined application of organic amendments (Pistacia vera) with gypsum enhanced solubility of Na+ (Mahmoodabadi et al. Citation2013), improving the availability of macronutrients such as N, P and K+ (Yazdanpanah et al. Citation2013) and protect the soil by favoring the appearance of spontaneous vegetation (Tejada et al. Citation2006). Amelioration strategies mainly rely on leaching of soluble salts with consequent decrease in EC. During reclamation, the final EC and ESP in the leachate may drop to 18% and 11.6% of their respective initial values (Yazdanpanah & Mahmoodabadi Citation2013). Amendments may increase the soil cation exchange capacity (CEC) and have greater adsorption of divalent cations than Na+ and thereby leachate contains high concentrations of Na+ (Jalali & Ranjbar Citation2009). Later, Yazdanpanah et al. (Citation2011) stated that leachate characteristics depend not only on the soil properties but also on the characteristics of the amendment.
The physical reclamation of salt-affected soils through leaching excess salts might be constrained by availability and high cost of high-quality irrigation water (Zhang et al. Citation2005). Amelioration through proton release from salt-tolerant legumes is increasingly becoming more widely used. Qadir et al. (Citation2003a, Citation2003b) reported that Na+ leaching was equivalent to that of gypsum. Mubarak and Nortcliff (Citation2010) concluded that some legumes that are known to fix N2 in calcareous salt-affected soils might be an alternative ameliorant to the extremely expensive gypsum through calcite solubilization and a consequent release of Ca2+. However, they tested cultivated legumes (mainly: hyacinth bean Dolichos lablab, cowpea Vigna unguiculata L.). Therefore, the aim of this work was to study the phytoremediation potential of P. glandulosa grown in calcareous saline-sodic soil. We hypothesized that bioremediation of such soils could be through (a) solubilization of native Ca2+ by the H+ released during NFIX and (b) Na+ exclusion.
Materials and methods
Soil
The soil, known as Jahgaru in Japan, was collected from the Okinawa Prefectural Kyushu Agricultural Research Center, Okinawa, Itoman city, Makabe 820, Japan. Jahgaru is a heavy-textured smectitic soil and shows problematic physical properties such as severe hardening after air-drying. The soil is classified as loamy, siliceous, thermic Typic Udorthents (CSCC Citation1994).
Addition of salts
The soil was air-dried, crushed and sieved through 2.0 mm sieve before additions of salts. The soil was made saline-sodic by dissolving NaCl (99.9% purity) in distilled water and sprayed on the soil surface to 80% of saturation and air-dried at 30°C followed by adding only distilled water to 80% saturation and left overnight then after oven dried at 80°C for three days. Addition of distilled water to 80% saturation and drying was repeated for three cycles before the soil was made ready for the next stage. The content of Na+ needed to raise the sodium adsorption ratio (SAR) to 19.9 and EC of the extract to 16.0 dS m−1 was calculated according to the following equation:
SAR = Na/[(Ca + Mg)/2]0.5.
However, the exchangeable sodium percent (ESP) was calculated from SAR values according to the following equation:
ESP (%) = 1.95 + 1.23 SAR (Rashidi & Seilsepour Citation2008).
The gypsum required (GR) to replace 60% of the exchangeable Na+ was calculated according to USDA (Citation1954) as follows:
GR (g/kg soil) = 60% × ESP (33.4%) × 0.86 = 3.75
This was followed by adjustment of CaCO3 from 5% to 8.3% using analytical powder CaCO3. shows some selected properties of the soil used in the study.
Table 1. Selected physico-chemical properties of the calcareous saline-sodic soil prepared from Jahgaru soil added to the lysimeters (mean ± standard deviation).
Remediation by P. glandulosa studied in soil lysimeters
The experiment involved the following treatments:
Control (no plant-no gypsum)
Gypsum added according to GR
Prosopis relying on inorganic N (IN)
Prosopis relying on NFIX
Sixteen lysimeters made from polyvinyl chloride (11.7 cm internal diameter and 30 cm height) were prepared. A hole was drilled (1 cm diameter) in the bottom of each lysimeter and a 25 cm leaching hose was tightly fixed inside the hole. A filter paper (No. 5A 110 circle mm, 0.09 mg/circle) was placed at the bottom of each lysimeter. About 2 cm height of acid/distilled-water-washed sand obtained from Japan Sea was then placed on top of the filter paper. Exactly 3.0 kg of the developed saline-sodic soil was then added to the lysimeters. In order to adjust the bulk density to 1.2 g cm−3 (almost similar to filed bulk density), the soil height inside the lysimeters was reduced to 25.3 cm by gently hand packing against a wooden table. About 4 cm was then left on top of each lysimeter for irrigation. The amount of distilled water equivalent to 70% of the soil water-holding capacity (WHC) was added in increments to all lysimeters before sowing. Prosopis seeds were immersed in boiled water (100°C) for 10 min, incubated for germination in moistened Petri-dishes at 35°C for 24 h before sowing (Majd et al. Citation2013). The inoculum (EN 15) supplied by the National Center for Research, Khartoum, Sudan was added at the rate of 10 g/kg soil and thoroughly mixed in lysimeters designated with NFIX and immediately 70% of the soil WHC was added. At sowing (9/12/2015), four germinated seeds were sown (1 cm deep) in the treatments with Prosopis (both relying on NFIX and IN) and soil moisture content was kept at 70% of WHC by regular weighing of an extra lysimeter filled with similar soil and packed to the same bulk density and then kept at 70% WHC. The lysimeters were arranged in a completely randomized design with four replications and placed in biohazard greenhouse. The temperature and light (500 W) during the growth period were maintained at 25°C ± 0.5 and 12 h, respectively.
After 150 (07/05/2016) days from sowing, plants were harvested at 2 cm height from the soil surface using a sharp blade. Plant height was measured (cm), oven dried (80°C for two days), weighed (mg plant−1), finely blended and analyzed for total carbon (TC) and total nitrogen (TN) by dry combustion (JM-MACRO CORDER 1000CN, J-SCIENCE CO, LTD, JAPAN) and content of Na+, Ca2+, Mg2+ and K+ (Chapman & Pratt Citation1982) after wet-acid digestion in a mixture of HNO3 and 30% H2O2 (Jones Citation2001) using auto-analyzer AA-6800, SHIMADZU Corporation, Japan). The soil in each lysimeter was removed carefully and divided into 0–15 and 15–30 cm, air-dried, crushed and sieved (2.0 mm). Soil paste was made and the pHpaste and EC of the paste extract were measured with DKK-TOA HM-25R, Japan and DKK-TOA CM-30R, Japan, respectively. From each extract, soluble cations (Na+, K+, Ca2+ and Mg2+) were determined with AA analyzer, (Bower & Wilcox Citation1965), Cl−,
,
and
were determined using ion Chromatography (Model L-7610 DEGASSER, Hitachi, Japan). The translocation factor (TF) or mobilization ratio of the element is used to determine the relative translocation of metals from the growing medium to other parts (root and shoot) and was calculated as the ratio of the element content in shoots to that in roots (Padmavathiamma & Li Citation2007). For salinity stress tolerance, the K+/Na+ ratio in the straw and root was calculated (Flowers Citation2004). The SAS (Citation1999) package was used to separate means between treatments, whereas differences between N2 fixing and non-fixing were determined using the T-Test.
Results
Plant parameters
Straw and root dry matter content and plant height of Prosopis relying on NFIX were higher (P ≤ .02,.01 and .02 for straw, root and plant height, respectively) than those relying on IN by 60%, 67% and 31%, respectively (). Although straw N content was higher (P ≤ .02) in plants relying on IN (by 29%), the TN in the root of NFIX treatment was higher (P ≤ .02) by 29%. Both Prosopis relying on NFIX or IN contained similar Ca2+ level in either the shoot or root. But Ca2+ level in the shoot was 50% (1.5 fold) than that in the root (P ≤ .01).
Table 2. Effects of NFIX and IN on dry matter content (DM, mg Plant−1), Plant height (cm), shoot and root content of C, N, Na+, Mg2+, Ca2+ (mg g−1) and C/N ratio of P. glandulosa grown in a calcareous saline-sodic soil. (mean ± standard deviation).
The content of Na+ in the above ground plant material relying on IN was 1.8 folds (P ≤ .03) than that relying on NFIX. However, the content of Na+ in the roots of plants relying on NFIX was almost 13% higher (P ≤ .04) than that relying on IN. The K+ level in the shoot was not different (P ≤ .67) between N2 fixing and non-fixing plants, however, roots of Prosopis relying on NFIX accumulated higher (P ≤ .02) K+ (by 24%) level than that relying on IN. In both plants, the ratio of shoot to root K+ was 1.5:1–1.8:1. The content of Mg2+ in the shoot (P ≤ .12) and roots (P ≤ .93) of both Prosopis was almost similar and varied from 0.86 to 1.05 mg g−1. Accordingly, the K+/Na+ ratio in the shoot of N2 fixing Prosopis was almost twofolds (P ≤ .005) that of non-fixing N2, whereas the ratio was similar (P ≤ .48) in the roots (). Only the translocation values (TF) of Na+ were higher (P ≤ .05) in non-fixing than N fixing Prosopis by 71% while the TF of K+ in the former was 20% higher (P ≤ .37) than in the latter by 20%, though not significant ().
Figure 1. Effects of NFIX and IN on K+/Na+ ratio in the straw and root of Prosopis glandulosa grown in a calcareous saline-sodic soil. Note: data are represented as means of four replications. Vertical bars are standard deviations. K+/Na+ ratio values of Straw IN and Straw NFIX or Root IN and Root NFIX with P ≤ .05 are significantly different using T-Test. Straw IN: straw in lysimeters with P. glandulosa relying on IN; Straw NFIX: straw in lysimeters with P. glandulosa relying on IN; Root IN: root in lysimeters with P. glandulosa relying on IN; Root NFIX: straw in lysimeters with P. glandulosa relying on nitrogen fixation.
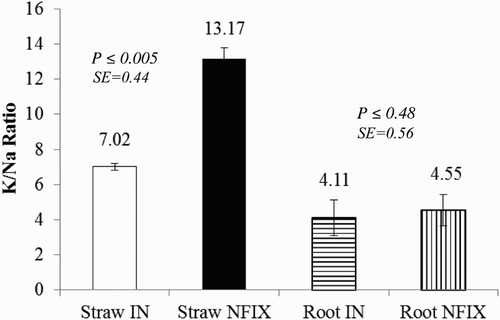
Table 3. Effects of NFIX and IN on TF (concentration in shoot/concentration in root) of Na+, K+, Ca2+ and Mg2+ of P. glandulosa grown in a calcareous saline-sodic soil (mean ± standard deviation).
Soil parameters
Treatments showed different effects on parameters determined in the 0–15 and 15–30 cm depths (). Incorporation of gypsum or planting Prosopis relying on NFIX has reduced (P ≤ .02) pH by 0.1–0.2 folds when compared with the control. The EC in the 0–15 cm depth was similarly reduced (P ≤ .001) with both gypsum application and planting Prosopis by 7–14% while in the lower depth, the gypsum treatment reduced (P ≤ .0006) salinity by 27%. In the 0–15 cm depth, the gypsum treatment showed the highest total salts’ concentration. The highest (P ≤ .0001) Na+ level was obtained in the gypsum lysimeter (more than twofolds of the control), whereas Na+ level in the NFIX lysimeter was higher (P ≤ .0001) than that obtained in the control (1.6 folds). Accordingly, the Na+ released in the gypsum, IN and N2 fixing lysimeters were 75.8, 29.6 and 37.4 meq L−1, respectively (P ≤ .0001). Therefore, the N2 fixing and non-fixing P. glandulosa released 39% and 50% Na+ of that released by the gypsum. At both depths, soluble Ca2+ level in Prosopis lysimeters was higher (P ≤ .0001–.01) than that obtained in the respective control lysimeter by 92–100% and 8–15%, respectively. The content of Mg2+ in both depths followed similar trend to Ca2+ where the levels in lysimeters with Prosopis were higher (P ≤ .002–.0001) than that obtained in control lysimeters by 100% and 17%, respectively. The levels of K+ in the soil solution of the gypsum and Prosopis treatments were higher (P ≤ .0001) than that of the control. However, K+ level in Prosopis relying on IN obtained in the 0–15 cm depth was exceptionally high (P ≤ .01). The SAR values of the 15–30 cm depth were lower (P ≤ .0001) in gypsum and lysimeters with Prosopis. The level of in the upper depth of lysimeters with Prosopis was higher (P ≤ .02) than in other treatments by 51–57%. However, in the lower depth of NFIX treatment, the level of
was higher (P ≤ .05) than other treatments by almost 22%. The level of Cl− in both depths was erratic where it was higher (P ≤ .0008) in the gypsum treatment of the upper depth and lower (P ≤ .0002) than the same treatment in the lower depth. At the 0–15 cm depth, the level of
was not different (P ≤ .18) among treatments, whereas in the 15–30 cm depth, the level in N2 fixing lysimeters was almost 10 folds (P ≤ .005) that found in the inorganic lysimeter. The
in the upper depth of the inorganic treatment was 3.5 folds (P ≤ .02) of other treatments. The level of soluble
in both depths was high (P ≤ .0001) and showed 1.8–6 folds of other treatments.
Table 4. Effects of NFIX, IN, Gypsum and Control on soil chemical properties (pHpaste, EC, ECe; soluble cations, Na+, K+, Ca2+, Mg2+ and anions, HCO3−, CI−, NO2−, NO3−, SO42− and sodium adsorption ratio, SAR) in the 0–15 and 15–30 cm depths after harvest of P. glandulosa grown in a calcareous saline-sodic soil (mean ± standard deviation).
Discussion
Plant parameters
Our data presented here aimed at answering a question if P. glandulosa could be used in reclaiming calcareous saline-sodic soils. The capacity of the plant to fix N2 and release H+ ion involved in solubilization of inherent CaCO3 is an important principle. Our data illustrated in showed that straw and root dry matter and root N content of P. glandulosa relying on NFIX are higher than that found in lysimeters with P. glandulosa relying on IN. These could possibly be attributed to tolerance of the shrub to salinity stress. These results corroborated findings of previous workers who reported high salinity tolerance for various Prosopis sp. (e.g. Ahmad et al. Citation1994; Baker et al. Citation1995; Cony & Trione Citation1998). Our results suggest that salinity and sodicity stresses could be augmented by supplementing the plants with N2-fixing bacteria. Accordingly, these findings may indicate that P. glandulosa tree could be a (1) strong alternative for rehabilitation of saline-sodic soils, (2) stabilizing active sands and (3) providing both fuel wood and browse for livestock in drylands ecosystems. Our results verified that of Geesing et al. (Citation2000), who indicated that the tree is an actively fixing N2 and this suggests that more attention should be given to the inclusion of tree legumes in arid ecosystems. However, it should be carefully noted that some Prosopis species are sensitive to Na2SO4 compared to NaCl when EC was more than 5 dS m−1 (Gómez et al. Citation2008). In this work, NaCl was the main source of salt used to develop salt stress. The question how Prosopis continued to fix N2 under salt-stress conditions may be explained by the work of Kurdali et al. (Citation2003), who reported 18% higher NFIX by dhaincha (Sesbania aculeate Pers.) under saline than under non-saline soil conditions, and attributed the plant’s fixing ability to the presence of effective salt-tolerant indigenous rhizobial strains and a lower N2 content of the soil.
The results presented in showed that the content of Na+ in the shoot of plants relying on NFIX was almost half that in lysimeters with plants relying solely on IN. This low Na+ accumulation indicates that Prosopis under saline-sodic conditions has an effective mechanism of excluding the element from the metabolically sensitive parts such as leaves. In contrast, the roots in the non-fixing N2 Prosopis transferred the Na+ to the shoot. Therefore, when plants were forced to fix N2 under salt-affected soil, the element tend to be retained in the roots of the N2 fixing plants. Moreover, results of our work also shown in indicated that the plants have accumulated Na+ in the roots and were able to regulate its transfer to the shoot. That our findings supported other results showed that the Na+ in leaves of Prosopis cineraria grown under saline conditions (EC of up to 13.3 dS m−1) exhibited a gradual increase while rapidly increased in roots in response to salinization of soil (Ramoliya et al. Citation2006). Because high concentration of Na+ may be detrimental to root tissues, exclusion of the element might happen during the turnover of fine roots or stem tissue may act as a barrier for translocation. It would be worthwhile to state that these results may indicate the priming effects of the rhizobium in increasing salt tolerance through element exclusion. Our results supported the earlier findings by Misra (Citation2000), who reported that Na+ in P. juliflora was confined to older storage components such as stem and branches, suggesting suitability for sodic soil amelioration and that the tree root systems contribute significantly to sodicity alleviation. The use of N2-fixing legumes in amelioration of calcareous (12.8% CaCO3) salt-affected (EC of 9.72 dS m−1) soils through exclusion of Na+ have been recently assessed by Cao et al. (Citation2012), who found 28.6% reduction of the element in the shoot of N2-fixing alfalfa. The exclusion of Na+ shown in of this study is consistent with the fact that ion-specific toxicity eventuates when individual salt ions’ concentration in the soil is in high quantity and plants store them beyond their holding capacity (Ferguson & Grattan Citation2005). For example, high levels of Na+ ions tend to disrupt the uptake of K+ ion transporters and thereby resulting in ion-specific toxicity damages photosynthesis (Dubery Citation2005). Although, in this study, K+ content in the shoot of P. glandulosa () was similar in N2-fixing and IN treatments, roots of the latter contained less K+ concentration. This might indicate that if the salinity stress continued in the absence of plant/microbial mutual benefits, disruption of K+ transfer to shoot would be expected. As presented in , our study revealed that the accumulation of K+ in the shoot and root was greater than that of Na+. This trend has been reflected in , which showed that the ratio between K+ and Na+ in both the shoot and root were more than 1. In the roots of both N2 fixing and non-fixing, this ratio was similar, whereas in the shoot of the former, the ratio was almost double the non-N2-fixing (). Additionally, the content of K+ in the straw of P. glandulosa presented in showed no significant difference between plants in lysimeters relying on either NFIX or IN. This explained that there was no decline in straw K+, suggesting that salt stress is not limiting the growth performance of Prosopis (Erdei & Teleisnik Citation1993). Despite the high concentration of Na+ in the soil solution that might compete with K+, our results revealed that the plant is tolerant to salinity stress at the EC level of the study with further positive priming effects of N2-fixing bacteria. These were recently proved by Bhuiyan et al. (Citation2015), who classified some grasses as salt tolerant when the K+/Na+ ratio was reported to be more than 1. Maintaining high cytosolic K+/Na+ ratio as a critical indicator of salt tolerance capacity of plants is well documented (Maathuis & Amtmann Citation1999; Flowers Citation2004; Akram et al. Citation2007). The efficiency of the plant in translocating accumulated elements from roots to shoots measured as TF is a direct estimate of the efficiency of phytoremediation. The effects of salt stress on TF values of Na+, K+, Ca2+ and Mg2+ observed in indicated that supplying N2-fixing rhizobium has greatly reduced the translocation of Na+ ion to the leaves and thereby increasing the K+/Na+ ratio in the leaves and improving salt tolerance stress. However, in absence of the N2-fixing bacteria, P. glandulosa tended to accumulate more Na+ ions, thereby may relatively reduce stress tolerance. The similar TF values of Ca2+ and Mg2+ () were consistent with similar content of Ca2+ and Mg2+ in the straw of mycorrhizal and non-mycorrhizal P. glandulosa presented in . Our study also observed that the content of Ca2+ in the straw of both P. glandulosa was almost threefolds that of Mg2+. These findings corroborated of Ramyoliya et al. (Citation2006), who observed that under saline conditions, content of Mg2+ in the leaves of P. cineraria was lower than that of both Ca2+ and Mg2+. Similarly, our results supported those obtained by Scambato et al. (Citation2011), who suggested that mycorrhizal colonization turned Prosopis alba more tolerant to salinity by maintaining the net photosynthesis and controlling transpiration rate.
Soil parameters
The effects of NFIX, IN, application of gypsum on soil chemical properties after harvest of the P. glandulosa shown in revealed that there is a reduction in pH found in lysimeters with Prosopis relying on NFIX. This may possibly be attributed to the extra H+ proton released during NFIX. The CEC of the soil used in this study is only 16.3 cmolc kg−1 (), which indicates a low buffering capacity to resist changes in pH. This result was similar to Schubert et al. (Citation1990), who found, in a laboratory experiment, acidification of soils with low CEC (from 7.11 to 20.88 cmolc kg−1) under N2-fixing filed bean (Vicia faba). Significant reduction (0.15 units) in pH was also determined under field conditions of 4 years cultivation of N2 fixing alfalfa in salt-affected soils (Cao et al. Citation2012). Our findings supported earlier suggestions that that the acidified rhizosphere with legumes resulted in a considerable extrusion of H+ to reduce soil pH (Tang et al. Citation1999). The present study showed reduction in total soluble salts obtained in Prosopis treatments (). In both lysimeters planted with Prosopis, the reduction in salinity was almost similar to that of the gypsum treatment. This suggests that Prosopis could have considerable potentials for bioremediation of salt-affected soils. The results of the present study supported previous finding that this tree can be used in bioremediation/ phytoremediation of salt-affected soils (e.g. Bhojvaid & Timmer Citation1998; Arya Citation2003). The content of soluble Na+ in the gypsum and Prosopis treatments presented earlier in was greater than that in the control; this shows that Na+ adsorbed to the exchange complex was replaced and injected into the soil solution. This could possibly be explained by high available Ca2+ and Mg2+ released either directly from the gypsum or through solubilization in non-gypsum lysimeters. Normally, reclamation of such soils based on phytoremediation or organic materials involves enhancement of the ability of Ca2+ and Mg2+ to replace Na+ from the exchangeable complex (Benbouali et al. Citation2013). Under such conditions, microbial respiration is generally high, resulting in better water aggregate stability and improvement of hydraulic conductivity that enhance removal of salts (Yazdanpanah et al. Citation2016). In this study, high levels of K+ in the soil solution and straw () found in the IN treatment is consistent with earlier findings by Fox and Guerinot (Citation1998), who reported that high Na+ normally depresses the uptake of K+. The low SAR values in the 15–30 cm depth found in the gypsum and Prosopis treatments of this work may further indicate the potential capacity of the plant in amelioration of sodic soils. Due to tolerance and adaptability of Prosopis affinis and Prosopis pallid, Goel and Behl (Citation1998) have recommended Prosopis for afforestation of sodic soils. However, less considerable changes in the SAR of the top 0–15 cm depth in Prosopis lysimeters compared to the gypsum treatment suggests that longer time may be needed to induce significant changes. In elsewhere, reduction in Na+ toxicity was observed in a field experiment with Prosopis after 5–7 years when there was build up of macro-elements (Bhojvaid & Timmer Citation1998).
The ionic composition of the saturated soil paste of this work revealed that the CI−1 and were the dominant anions in both depths. The use of gypsum in our study has increased the level of soluble
in the soil to extremely high levels. Sulfate ion is expected to be released in the soil solution during incorporation of gypsum (CaSO4·2H2O). The use of gypsum in reclamation of saline-sodic soils is well documented (e.g. Abdel-Fattah et al. Citation2015). As shown in of this study, the concentration of
in the top 0–15 cm depth of lysimeters with Prosopis was similar. This similarity could be explained in two possible scenarios: (1) few amount of fine roots that have been reflected in production of low concentration of H+ ions to induce significant solubilization of CaCO3 or (2) the
produced as the results of solubilization have been leached down to the 15–30 cm depth. We speculate that the latter scenario was the main reason as the level of
in the lower depth in lysimeters with NFIX was 21% higher (P ≤ .05) than that in lysimeters with IN (). Therefore, the present work revealed that roots of both Prosopis might have similar CO2 released during respiration, enhancing the partial pressure of CO2 (PCO2) that could be involved in dissolution of calcite and thereby resulted in elevated
concentration. These findings are similar to others reported elsewhere (Badía Citation2000; Qadir et al. Citation2005). As for the lower depth (15–30 cm) where treatments with Prosopis relying on NFIX showed higher levels of
, this extra anion concentration could be due to both the influence of the PCO2 and reaction of the H+ proton released during NFIX with calcite and thereby releasing additional
content. The content of Ca2+ reported in this study indicates that solubilization by the gypsum was more than fourfolds that obtained in both Prosopis treatments. Our results may suggest that the increase in Ca2+ and
concentrations in the soil solution of Prosopis treatments shown in could be due to either dissolution of the calcite as a source of Ca2+ potentially enhanced by releases of H+ proton or enhanced root respiration that releases CO2 in the rhizosphere as a result of exposure of Prosopis to salinity, thereby increasing demand for energy (Lambers et al. Citation1998). Higher levels of Ca2+ and
levels during phytoremediation of calcareous saline soils using N2 fixing legumes were earlier reported (Qadir et al. Citation2003a, Citation2003b; Mubarak & Nortcliff Citation2010; Cao et al. Citation2012).
In conclusion, this study showed that both plants retained similar Ca2+, and the Na+ level in the straw of non-fixing plants was 1.8 folds that of N2 fixing plants, whereas levels of Na+ and K+ in roots of N2 fixing were 13% and 24% higher than that of non-fixing plants. Based on the findings reported here, it can be inferred that (1) N2 fixing and non-fixing plants released 39% and 50% Na+ of that released by the gypsum and (2) both Prosopis produced higher than the gypsum, which induced extra Ca2+ solubilization of the gypsum, growing P. glandulosa on calcareous salt-affected soils could be an alternative management practice to reduce salinity and sodicity effects.
Notes on contributors
Mubarak Abdelrahman Abdalla is a Professor of Soil Science at the Department of Soil and Environment Sciences, Faculty of Agriculture, University of Khartoum, Shambat, Sudan. He has written more than 50 articles in the field of land degradation and development.
Abdelkarim Hassan Awad Elkarim is an Associate Professor of Soil Science at the Department of Soil and Environment Sciences, Faculty of Agriculture, University of Khartoum, Shambat, Sudan. He has written more than 20 articles in the field of Soil Chemistry.
Takeshi Taniguchi is an Associate Professor at the Arid Land Research Center, Tottori University. He published many articles in the science of ecology of plant symbiotic microorganisms in drylands. He concentrated in search for effective plants symbiotic microorganisms (mycorrhizal fungi, endophytic fungi, and rhizosphere bacteria) to enhance drought and salt stress tolerance of plants, analysis of the ecological and functional features of symbiotic microorganisms and use of plant symbiotic microorganisms for ecosystem restoration and agricultural production in drylands.
Tsuneyoshi Endo is a Professor in the Faculty of Agriculture, Tottori University, Tottori, Japan. He has excellent achievements in land use effects on soil quality.
Norikazu Yamanaka is a Professor in the Arid Land Research Center, Tottori University. He is specialized in revegetation science: plant ecology and ecosystem restoration in arid lands. Currently he is the Director of the center. He has ample publications and text books in ecological studies of plant communities in arid areas, ecophysiological studies on drought and salt tolerance of woody plants and studies on the ecosystem restoration in arid areas.
Acknowledgements
This study was supported by the Arid Land Research Center, Tottori University, Japan. We are grateful to members of the Division of Environmental Conservation, ALRC, Tottori University for helping us during preparation of soil samples and University of Khartoum, Sudan for providing sabbatical leave.
Disclosure statement
No potential conflict of interest was reported by the authors.
Additional information
Funding
References
- Abdel-Fattah MK, Fouda S, Schmidhalter U. 2015. Effects of gypsum particle size on reclaiming saline-sodic soils in Egypt. Commun Soil Sci Plant Anal. 46:1112–1122.
- Ahmad RS, Ismail S, Moinuddin M, Shaheen T. 1994. Screening of mesquite (Prosopis spp) for biomass production at barren sandy areas using highly saline water for irrigation. Pak J Bot. 26:265–282.
- Ahmad S, Ghafoor A, Akhtar ME, Khan MZ. 2015. Implication of gypsum rates to optimize hydraulic conductivity for variable-texture saline-sodic soils reclamation. Land Degrad Dev. 27(3):550–556.
- Akram M, Malik MA, Ashraf MY, Saleem MF, Hussain M. 2007. Competitive seedling growth and K+/Na+ ratio in different maize (Zea mays L.) hybrids under salinity stress. Pak J Bot. 39:2553–2563.
- Arya R. 2003. Vegetation status of salt-affected soil five years plantation. In: Narain P, Kathju P, Kar S, Singh MP, Kumar P, editors. Human impact on desert environment. Jodhpur: Arid Zone Research Association of India; p. 213–219.
- Aslam R, Bostan N, Nabghae A, Maria M, Safdar W. 2011. A critical review on halophytes: salt tolerant plants. [Review]. J Med Plants Res. 5(33):7108–7118.
- Aydemir S, Sünger H. 2011. Bioreclamation effect and growth of a leguminous forage plant (Lotus corniculatus) in calcareous saline sodic soils. Afr J Biotechnol. 10(69):15571–15577.
- Badía D. 2000. Straw management effects on organic matter mineralization and salinity in semiarid agricultural soils. Arid Soil Res. 14:193–203.
- Baker A, Sprent JI, Wilson J. 1995. Effects of sodium chloride and mycorrhizal infection on the growth and nitrogen fixation of Prosopis juliflora. Symbiosis. 19:39–51.
- Benbouali EH, Hamoudi SAEA, Larich A. 2013. Short-term effect of organic residue incorporation on soil aggregate stability along gradient in salinity in the Lower Cheliff plain (Algeria). Afr J Agric Res. 8(19):2144–2152.
- Bhojvaid PP, Timmer VR. 1998. Soil dynamics in an age sequence of Prosopis juliflora planted for sodic soil restoration in India. For Ecol Manag. 106:181–193.
- Bhuiyan MSI, Raman A, Hodgkins DS, Mitchell D, Nicol HI. 2015. Salt accumulation and physiology of naturally occurring grasses in saline soils in Australia. Pedosphere. 25:501–511.
- Bower CA, Wilcox LV. 1965. Soluble salts. In: Black CA, editor. Methods of soil analysis. Madison (WI): American Society of Agronomy; p. 933–951.
- Cao J, Xianting L, Kong X, Zed R, Dong L. 2012. Using alfalfa (Medicago sativa) to ameliorate salt-affected soils in Yingda irrigation district in Northwest China. Acta Ecol Sin. 32:68–73.
- Chapman HD, Pratt PF. 1982. Methods of analysis for soils, plants and water. Methods of soil analysis Part 1: Physical and mineralogical methods, 2nd ed. Agronomy series No: 9. Madison (WI): Am. Soc. of Agronomy and Soil Sci of Am. Inc. Publisher; p. 363–381.
- Cony MA, Trione SO. 1998. Inter and intraspecific variability in Prosopis flexuosa and P. chilensis: Seed germination under salt and moisture stress. J Arid Environ. 40:307–317.
- Cultivated Soil Classification Committee. 1994. Classification of cultivated soils in Japan, third approximation.
- De Boever M, Gabriels D, Ouessar M, Cornelis W. 2016. Influence of acacia trees on near-surface soil hydraulic properties in arid Tunisia. Land Degrad Dev. 27(8):1805–1812.
- Dregne H, Kassas M, Rosanov B. 1991. A new assessment of the world status of desertification. Desertif Control Bull. 20:6–18.
- Dubery RS. 2005. Photosynthesis in plants under stressful conditions. In: Pessarakli M, editor. Handbook of photosynthesis. 2nd ed. New York (NY): CRC Press; p. 717–718.
- Elfadl MA, Luukkanen O. 2003. Effect of pruning on Prosopis juliflora: considerations for tropical dryland agroforestry. J Arid Environ 53:441–455.
- Emtahani MR, Elmi M. 2006. The ecological studies of Prosopis koelziana in south of Iran. J Desertif. 1:1–11.
- Erdei L, Teleisnik F. 1993. Changes in water relation parameters under osmotic and salt stresses in maize and sorghum. Physiol Plant. 9:381–387.
- Ferguson L, Grattan SR. 2005. How salinity damages citrus: osmotic effects and specific ion toxicities. Hortic Technol. 15:95–99.
- Flowers TJ. 2004. Improving crop salt tolerance. J Exp Bot. 55:307–319.
- Fox TC, Guerinot ML. 1998. Molecular biology of cation transport in plants. Ann Rev Plant Physiol Plant Mol Biol. 49:669–696.
- Geesing D, Felker P, Bingham L. 2000. Influence of mesquite (Prosopis glandulosa) on soil nitrogen and carbon development: implications for global carbon sequestration. J Arid Environ. 46:157–180.
- Goel VL, Behi HM. 1998. Screening prosopis germlasm for afforestation of degraded soil sites. J Sustain For. 8:1–13.
- Gómez RR, Salas-García CE, Monroy-Ata A, Solano E. 2008. Salinity effect on Prosopis laevigata seedlings. Publicado Terra Latinoam. 28:99–107.
- Jalali M, Ranjbar F. 2009. Effects of sodic water on soil sodicity and nutrient leaching in poultry and sheep manure amended soils. Geoderma. 153:194–204.
- Jesus J, Danko AS, Fúza A, Borges MT. 2015. Phytoremediation of salt affected soils: a review of processes, applicability and the impact of climate change. Environ Sci Pollut Res. 22:6511–6525.
- Jones Jr JB. 2001. Laboratory guide for conducting soil tests and plant analysis. Boca Raton (FL): CRC Press.
- Joshi AJ, Hinglajia H. 2000. Accumulation of organic and inorganic solutes in Prosopis juliflora (Sw) DC. growing in coastal belt of Gujarat. Proc Natl Acad Sci India; Sect B. Biol Sci. 70:171–178.
- Kurdali F, Janat M, Khalifa K. 2003. Growth and nitrogen fixation and uptake in dhaincha/sorghum intercropping system under saline and non-saline conditions. Commun Soil Sci Plant Anal. 34:2471–2494.
- Lambers H, Chapin FS, Pons TL. 1998. Plant physiological ecology. New York (NY): Springer-Verlag.
- Maathuis FJM, Amtamann A. 1999. K+ nutrition and Na+ toxicity. The basis for cellular K+/Na+ rations. Ann Bot. 84:123–133.
- Mahmoodabadi M, Yazdanpanah N, Sinobas LR, Pazira E, Neshat A. 2013. Reclamation of calcareous saline sodic soil with different amendments (I): redistribution of soluble cations within the soil profile. Agric Water Manag. 120:30–38.
- Majd R, Aghaie P, Monfared EK, Alebrahim MT. 2013. Evaluating of some treatments on breaking seed dormancy in Mesquite. Int J Agron Plant Nutr. 4(7):1433–1438.
- Manzano MG, Navar J. 2000. Processes of desertification by goats heavy grazing in the Tamaulipan thornscrub (matorral) in northeastern Mexico. J Arid Environ. 44:1–17.
- Mazaheri MR, Mahmoodabadi M. 2012. Study on infiltration rate based on primary particle size distribution data in arid and semi arid region soils. Arabian J Geosci. 5(5):1039–1046.
- Min W, Guo H, Zhang W, Zhou G, Ma L, Ye J, Liang Y, Hou Z. 2016. Response of soil microbial community and diversity to increasing water salinity and nitrogen fertilization rate in an arid soil. Acta Agric Scandin, Section B-Soil and Plant Sci. 66:117–126.
- Misra D. 2000. Early tree-root relationships of prosopis, eucalyptus, and azadirachta planted on sodic soils [M.Sc. Thesis]. (Canada): Department of Forestry, University of Toronto.
- Mubarak AR, Nortcliff S. 2010. Calcium carbonate solubilization through H-proton release from some legumes grown in calcareous saline-sodic soil. Land Degrad Dev. 21(1):29–39.
- Mustafa MA. 2007. Desertification processes [ UNESCO Chair of Desertification]. Khartoum: University of Khartoum Printing Press; p. 230.
- Padmavathiamma PK, Li LY. 2007. Phytoremediation technology: hyper-accumulation metals in plants. Water Air Soil Pollut. 184:105–126.
- Qadir M, Noble AD, Oster JD, Schubert S, Ghafoor A. 2005. Driving forces for sodium removal during phytoremediation of calcareous sodic and saline-sodic soils: a review. Soil Manag. 21:173–180.
- Qadir M, Oster J. 2002. Vegetative bioremediation of calcareous sodic soils: history, mechanisms, and evaluation. Irrig Sci. 21:91–101.
- Qadir M, Schubert S. 2002. Degradation processes and nutrient constraints in sodic soils. Land Degrad Dev. 13(4):275–294.
- Qadir M, Steffens D, Yan F, Schubert S. 2003a. Proton release by N2-fixing plant roots: a possible contribution to phytoremediation of calcareous sodic soils. J Plant Nutr Soil Sci. 166:14–22.
- Qadir M, Steffens D, Yan Y, Schuber S. 2003b. Sodium removal from a calcareous saline-sodic soil through leaching and plant uptake during phytoremediation. Land Degrad Dev. 4:301–307.
- Ramoliya PJ, Patel HM, Joshi JB, Pandey AN. 2006. Effect of salinization of soil on growth and nutrient accumulation in seedlings of Prosopis cineraria. J Plant Nutr. 29:283–303.
- Rashidi M, Seilsepour M. 2008. Modeling of soil exchangeable sodium percentage based on soil adsorption ratio. J Agric Biol Sci. 3(4):22–26.
- Salih AA. 1998. Root and shoot growth of Prosopis chilensis in response to soil impedance and soil matric potential. J Arid Environ. 40:43–52.
- SAS Institute Inc. 1999. SAS/STAT user’s guide, Version 6.12. Cary (NC): SAS Institute.
- Scambato AA, Echeverria M, Sansberro P, Ruiz OA, Menéndez AB. 2011. Glomus intraradices improved salt tolerance in Prosopis alba seedlings by improving water use efficiency and shoot water content. Braz J Plant Physiol. 22(4):285–289.
- Schubert S, Schubert E, Mengel K. 1990. Effect of low pH of the root medium on proton release, growth, and nutrient uptake of field beans (Vicia faba). Plant Soil. 124:239–244.
- Singh G, Singh H, Bhoivaid PP. 1998. Amelioration of sodic soils by trees for wheat and oat production. Land Degrad Dev. 9:453–462.
- Tang C, Unkovich MJ, Bowden JW. 1999. Factors affecting soil acidification under legumes III. Effects of nitrate supply. New Phytol. 143:513–521.
- Tejada M, Garcia C, Gonzalez JL, Hernandez MT. 2006. Use of organic amendment as a strategy for saline soil remediation: influence on the physical, chemical and biological properties of soil. Soil Biol Biochem. 38:1413–1421.
- USDA. 1954. Diagnosis and improvement of saline and alkali soils (agriculture handbook no. 60). Washington (DC): US Government Printing Office.
- Yazdanpanah N. 2016. Spatiotemporal mapping of groundwater quality for irrigation using geostatistical analysis combined with a linear regression method. Model Earth Syst Environ. 2(18):1–13.
- Yazdanpanah N, Mahmoodabadi M. 2013. Reclamation of calcareous saline- sodic soil using different amendments: time changes of soluble cations in leachate. Arab J Geosci. 6:2519–2528.
- Yazdanpanah N, Mahmoodabadi M, Cerdà A. 2016. The impact of organic amendments on soil hydrology, structure and microbial respiration in semiarid lands. Geoderma. 266:58–65.
- Yazdanpanah N, Pazira E, Neshat A, Mahmoodabadi M, Sinobas LR. 2013. Reclamation of calcareous saline sodic soil with different amendments (II): impact on nitrogen, phosphorous and potassium redistribution and on microbial respiration. Agric Water Manag. 120:39–45.
- Yazdanpanah N, Pazira E, Neshat A, Naghavi H, Moezi AA, Mahmoodabadi M. 2011. Effect of some amendments on leachate properties of a calcareous saline-sodic soil. Int Agrophys. 25(3):307–310.
- Yensen, NP, Biel KY. 2006. Soil remediation via salt-conduction and the hypotheses of halosynthesis and photoprotection. In: Khan MA, Weber DJ, editors. Ecophysiology of High Salinity Tolerant Plants, Tasks for Vegetation Science 40. Springer, Dordrecht; p. 313–344.
- Zhang JF, Zhang XD, Zhou JX, Liu GH. 2005. World resources of saline soil and main amelioration measures. Res Soil Water Conserv. 12:28–30.