ABSTRACT
Experimental System: Fermented dried fruit of wild cucumber (Cucumis myriocarpus Naude.) Had been successfully used as Nemarioc-AL phytonematicide in managing plant-parasitic nematodes in various cropping systems. However, the specialised oven-drying equipment are inaccessible to most resource-poor smallholder farmers in marginalised communities. Procedures: The objective of this study was to determine the efficacy of fermented crudes extracts of C. myriocarpus fruit when used in fresh form as Nemarioc-AL phytonematicide on management of population densities of root-knot (Meloidogyne species) nematodes and growth of tomato (Solanum lycorpersicum L.) plants. Seedlings at five leaf-stage were transplanted into pots containing 2700 ml steam-pasteurised river sand and Hygromix at 3:1 (v/v) ratio. Each seedling was inoculated with approximately 3000 eggs and second-stage juveniles (J2) at transplanting. The phytonematicide was applied at 0%, 2%, 4%, 8%, 16%, 32% and 64%, arranged in a randomised complete block design, with five replications and validated in time. Results: At 56 days after treatment, nematode variables and phytonematicide concentrations exhibited negative quadratic relations, with coeffients of determination (COD) ranging from 93% to 97%. Similarly, plant variables and phytonematicide concentrations exhibited positive quadratic relations, with COD from 96% to 98%. Conclusions: In conclusion, when prepared from fresh fruit of C. myriocarpus, Nemarioc-AL phytonematicide was suitable for use in managing nematode population densities of Meloidogyne species in tomato production.
Introduction
Worldwide, root-knot (Meloidogyne species) nematodes induce widespread crop yield losses, with plant genotypes that lack nematode resistance suffering from as high as 50% yield losses to complete crop failure (Thies and Levi Citation2007). The genus Meloidogyne is among the most damaging soil-borne pathogens of tomato ( Solanum lycorpersicum L.) plants, with estimated yield losses ranging from 28% to 68% (Adesiyan et al. Citation1990). In tomato production, the economic damage threshold level ascribed to Meloidogyne species is 0.1–1.0juveniles/cm3 soil at transplanting (Sikora and Fernández Citation2005). In tropical Meloidogyne species, namely, M. incognita and M. javanica, the temperature-dependent life cycles are approximately 30 days (Sikora and Fernández Citation2005). Generally, with predicted higher temperatures ascribed to global warming (Steyn et al. Citation2014), the life cycles of Meloidogyne species would probably be much shorter than the current life cycles. Due to the withdrawal of methyl bromide and related fumigant nematicides from the agrochemical markets, much focus had since been shifted to research and development of alternative nematode management strategies in various cropping systems.
The cucurbitacin-containing phytonematicides are being researched and developed in South Africa as alternatives for managing the tropical Meloidogyne species (Mashela et al. Citation2016). Primarily, the products are prepared from dried fruits of wild cucumber (Cucumis myriocarpus Naude.) and wild watermelon (Cucumis africanus L.F.) using the concept of green biotechnologies. Fruits were oven-dried at 52°C for 72 h, powdered using Thomas Wiley Mill (Thomas Scientific) and then fermented (Mashela et al. Citation2016) using locally-available strains of effective microorganisms (EM).
Sun- or shade-drying methods were (Shadung et al. Citation2016) not effective due to cucurbitacin-losses through volatilisation. On the other hand, freeze-drying of fresh fruits resulted in reduced cucurbitacin concentrations (Shadung et al. Citation2016), for reasons which had not been clearly understood. Oven-drying at 52°C for 72 h was, among the listed methods, the most suitable for the preparation of fruits from any of the two Cucumis species in production of phytonematicides. At lower and higher temperatures proliferation of rotting microorganisms and high cucrbitacin-losses occurred, respectively (Shadung et al. Citation2016). However, air-forced ovens used in the drying method at 52°C would not be accessible to resource-poor smallholder farmers, who had since shown much interest in the use of phytonematicides. Fresh fruits could not be stored due to high incidents of post-harvest rots induced Penicillium simplicissimum as an acid-loving fungus. (Mphahlele et al. Citation2012). Effective microorganisms used in fermentation contain actinomycetes, which eliminate most disease-inducing pathogens. The objective of this study was to investigate the efficacy of fermented fresh fruit from C. myriocarpus plants in the production of Nemarioc-AL phytonematicide for suppression of Meloidogyne species and growth of tomato plants.
Materials and methods
Growing and fermentation conditions
A greenhouse experiment was conducted at the Green Biotechnologies Research Centre of Excellence, University of Limpopo, South Africa (23°53′10″S, 29°44′15″E) in spring (July-September) 2016 and repeated in summer (October-December) 2016. Ambient day/night greenhouse temperatures averaged 25/16°C, with maximum temperatures controlled using thermostatically-activated fans. Mature fresh fruit of C. myriocarpus were harvested from a cultivated field, washed and cut into pieces, with 411 g material (≈ 80 g dried fruit) in a 20 L plastic container fermented using effective microorganism (EM) comprising local strains of yeast, lactic acid, phytosynthetic and actinomycete bacteria in 16 L chlorine-free tapwater for 14 days to allow pH to decline to 3.7 (Mashela et al. Citation2016).
Raising of seedlings and inoculation
Tomato cv. ‘Floradade’ seedlings were raised in 200-cone seedling trays, containing Hygromix-T (Hygrotech, Pretoria, South Africa) growing mixture. After hardening-off using intermittent withdrawal of irrigation water for two weeks outside the greenhouse, seedlings at five-leaf stage were transplanted into 20-cm-diameter plastic pots, each filled with 2700 ml steam-pasteurised (300°C for 1 h) river sand and Hygromix-T at 3:1 (v/v) ratio. Pots were arranged on green house benches at 0.25 m inter row and 0.25 m intra row spacing and irrigated to field capacity. Uniform five-week-old tomato cv. ‘Floradade’ seedlings were transplanted a day after irrigation to field capacity. Nematode inocula were prepared by extracting eggs and second-stage juveniles (J2) of M. incognita race 2 from roots of greenhouse-raised nematode-susceptible kenaf (Hibiscus cannabinus, L.) in 1% NaOCl solution (Hussey and Barker Citation1973). Each tomato seedling was inoculated with 3000 eggs and J2 of M. incognita race 2 using a 20 ml syringe to place inocula in 5 cm deep holes on cardinal sides at 5 cm away from the stem, with holes covered with the growing mixture and slightly irrigated with 300 ml chlorine-free water.
Experimental design and cultural practices
Due to the heterogeneous conditions that were created by the heat-extracting fans within the greenhouse, the treatments, namely, 0%, 2%, 4%, 8%, 16%, 32% and 64% Nemarioc-AL phytonematicide, were arranged on benches in a randomised complete block design, with five replicates. Three days after transplanting, each plant was fertilised with 3 g 2:3:2 (22) + 0.5% Zn + 5% S + 5% Ca to provide 186 N, 126 K and 156 P mg/ml water, with 2 g 2:1:2 (43) Multifeed (Nulandis, Witfield, South Africa) providing 0.35 N, 0.32 K and 0.32 P, 0.9 Mg, 0.75 Fe, 0.075 Cu, 0.35 Zn, 1.0 B, 3.0 Mn and 0.07 Mo mg/ml water. Four sets of iTuin Metre (Institute of Opto-Electronic Technology, Beijing) were inserted to 15 cm depths in randomly selected pots to monitor soil moisture tension, with plants irrigated to full capacity using chlorine-free tapwater at 50% moisture depletion. Scouting for the greenhouse whitefly (Trialeurodes vaporariorum West.) was performed weekly with plants sprayed using 1.33 ml fenthion 50%/L water when population densities increased above 10 whiteflies per five randomly selected plants.
Data collection
At 56 days after initiating the treatments, flowers were counted and fruit collected. Plant height was measured from the soil surface to the tip of the flag leaf. Stems were severed at the soil surface and stem diameters measured at 5-cm above the severed ends using a digital vernier calliper. Root systems were removed from pots, immersed in water to remove soil particles, blotted dry and weighed, whereas eggs and J2 were extracted from randomly sampled 10 g root/plant using maceration and blending for 60s in 1% NaOCl solution (Hussey and Barker Citation1973). The aliquot was passed through nested 75- and 25-μm-pore sieves, with contents from the latter collected for separation of nematodes from debris using the sugar-floatation and centrifugation method (Jenkins Citation1964). Soil in each pot was mixed and a 250-ml soil sample collected for nematode extraction using the sugar-floatation and centrifugation method (Jenkins Citation1964). Eggs and J2 from roots and J2 from soil were separately counted under a stereomicroscope and recorded as reproductive potential (RP = eggs and J2/g root) and J2/250 ml soil, respectively. Shoots and roots were dried in air-forced ovens at 70°C for 72 h.
Data analysis
Data were subjected to analysis of variance through the 2008 SAS software (SAS Institute INC Citation2008). Prior to analysis, flower and nematode numbers were each transformed through log10(x + 1) to homogenise the variances (Gomez and Gomez Citation1984). Mean sum of squares were partitioned to determine the total treatment variation (TTV) in plant and nematode variables (Gomez and Gomez Citation1984). A geometric series, 0%, 2%, 4%, 8%, 16%, 32% and 64% phytonematicide was used to generate the density-dependent growth (DDG) patterns (Mashela et al. Citation2016). However, to avoid curve-fitting challenges with observations crowded in lower concentrations, equidistances between adjacent observations (Liu and An Citation2005), were generated by transforming concentrations to log22 thereby generating 0%, 1%, 2%, 3%, 4%, 5% and 6% phytonematicide for use on lines of the best fit (Causton Citation1977). In cases where the relations between the plant variables and concentrations exhibited quadratic relations, optimisation was achieved using x = −b1/2b2 relation derived from Y = b2 x2 + b1 x + C (Gomez and Gomez Citation1984). Unless otherwise stated, treatment means were discussed at the probability level of 5%.
Results
Seasonal interactions for nematode and plant variables were not significant. Consequently, data were pooled (n = 70) and re-analysed using SAS software.
Nematode variables
Treatments had highly significant effects on eggs and J2 in root and J2 in soil, contributing 68% and 87% in TTV of the respective variables. Eggs and J2 in roots and/ or J2 in soil versus phytonematicide concentrations each exhibited quadratic relations (). In root and soil the relations had COD of 96% and 93%, respectively. Using x = −b1/2b2 relation, the nematode population density in roots was optimised at 0.22% phytonematicide, whereas the minimum nematode population density of J2 in soil was achieved at 11.08% phytonematicide ().
Figure 1. Response of eggs and second-stage juveniles (J2) of Meloidogyne incognita in roots (A) in soil (B) to Nemarioc-AL phytonematicide concentrations.
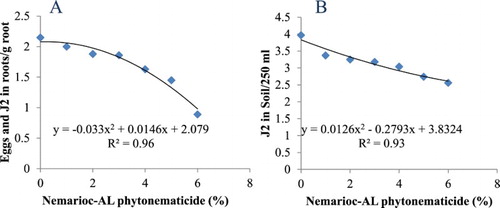
Table 1. Optimisation models for Meloidogyne incognita variables in root and soil to increasing concentrations of Nemarioc-AL phytonematicide at 56 days after treatment (n = 70).
Plant variables
Flowers number, fruit number, dry root mass and stem diameter over increasing phytonematicide concentrations each exhibited positive quadratic relations (). Models had 97%, 98%, 96% and 97% COD, respectively. The four plant variables were optimised at 2.18%, 2.23%, 2.14% and 1.67% phytonematicide, respectively ().
Figure 2. Response flower number (A), fruit number (B), dry root mass (C) and stem diameter (D) in tomato cv. ‘Floradade’ to Nemarioc-AL phytonematicide concentrations.
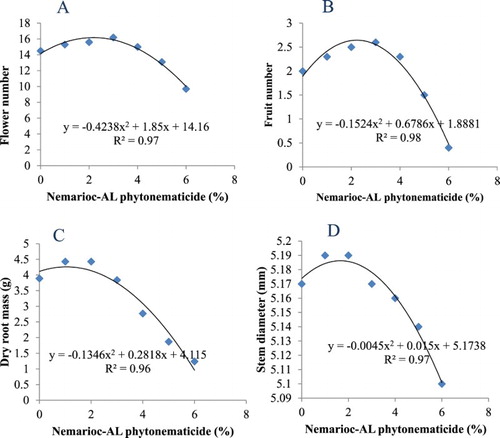
Table 2. Optimisation models for tomato plant variables to increasing concentrations of Nemarioc-AL phytonematicide at 56 days after treatments (n = 70).
Discussion
Nematode variables
Nematodes in roots and soil with increasing concentrations of Nemarioc-AL phytonematicide exhibited positive and negative quadratic relations, respectively (), thereby confirming the existence of DDG patterns (Liu and An Citation2005; Mashela et al. Citation2016). In root samples, with the optimum nematode population density achieved at 0.22% Nemarioc-AL phytonematicide (), suggested the stimulation of eggs and J2 in roots. As observed under in vitro trials, at low concentration of cucurbitacin-containing phytonematicides (Dube Citation2016), Nemarioc-AL phytonematicide had slight stimulation effects on eggs and J2 in roots (), as corroborated by optimum growth of the variables ().
The negative quadratic relation observed on J2 in soil over increasing concentration of Nemarioc-AL phytonematicide agreed with the inhibition effects of cucurbitacin-containing phytonematicides on Meloidogyne species (Mashela et al. Citation2016). In the current study, the minimum nematode population density in soil was achieved at a relatively higher phytonematicide concentration, namely 11.08% (). Generally, gradual exposure of eggs and J2 to stressful conditions does not wipe out nematodes since various nematode stages have the ability to enter the survival phases referred to dauer and cryptobiosis, respectively (Perry Citation1999). These abilities could further cast some light in the existence of cyclic growth patterns of nematodes that had been observed in plant-parasitic nematodes under intermittent and/or gradual stressful conditions such as salinity (Mashela et al. Citation1992). In context of DDG patterns, cucurbitacin-containing phytonematicides can either stimulate, have no effect or inhibit nematode population densities, as observed under in vitro (Dube and Mashela Citation2017) and ex vitro of C. myriocarpus. Also, the observed DDG patterns had since settled the misconception that phytonematicides had ‘inconsistent results’ on suppression of nematode population densities (Mashela et al. Citation2016). Almost always, the product, regardless of whether it was prepared from dried or fresh fruits, reduced root-knot nematode population densities with considerably high margins.
Plant variables
Responses of plant variables over increasing concentration of Nemarioc-AL phytonematicide each suggested the existence of DDG patterns (), thereby confirming the view that biological entities respond to allelochemicals in context of DDG patterns (Liu and An Citation2005; Salisbury and Ross Citation2005). In plant variables, the stimulation and inhibition phases in context of DDG patterns were clearly defined. The advantage of DDG patterns was, among other things, that the generated quadratic equations provided an opportunity to optimise (x = −b1/2b2) concentrations for the test variables (). Beyond the optimisation concentration the plant variables were invariably inhibited, which could be essential in decision-making when using Nemarioc-AL phytonematicide.
The findings in the current study provided evidence that Nemarioc-AL phytonematicide prepared from fresh fruit of C. myriocarpus also contains bioactivities with nematicidal properties against M. incognita eggs and J2 in roots and in soil. Also, at low concentrations the product had stimulation effects on growth of tomato plants, whereas at high concentrations plant growth was invariably inhibited. In conclusion, Nemarioc-AL phytonematicide from fresh C. myriocarpus fruit would be suitable for use in management of nematode population densities in tomato production.
Disclosure statement
No potential conflict of interest was reported by the authors.
Notes on contributors
P. E. Tseke and P. W. Mashela are research assistant and senior professor of nematology, respectively, at the Green Biotechnologies Research Centre of Excellence, University of Limpopo.
Additional information
Funding
References
- Adesiyan SO, Caveness FE, Adenyi MO, Fawole B. 1990. Nematode pests of tropical crops. Ibadan (Nigeria): Heinemann Educational Books PLC.
- Causton DR. 1977. Contemporary biology: a biologist’s mathematics (23). (Ed.). Barrington EJW, Willis J. 41 Bedford Square, London WCIB 3DO.
- Dube ZP. 2016. Nemarioc-AL and Nemafric-BL phytonematicides: bioactivities in Meloidogyne incognita, tomato crop, soil type and organic matter [PhD thesis]. Sovenga (South Africa): University of Limpopo.
- Dube ZP, Mashela PW. 2017. In vitro characterization of Meloidogyne incognita juvenile egress and mortality under Nemarioc-AL phytonematicide. Res Crops. 18:379–383. doi: 10.5958/2348-7542.2017.00066.3
- Gomez KA, Gomez AA. 1984. Statistical procedures for agricultural research. Wiley: New York.
- Hussey RS, Barker KR. 1973. A comparison of methods of collecting inoculants of Meloidogyne species including a new technique. Plant Dis Rep. 57:1025–1028.
- Jenkins WR. 1964. A rapid centrifugal-floatation technique for separating nematodes from soil. Plant Dis Rep. 48:692.
- Liu DL, An M. 2005. Implementation of card: curve-fitting allelochemical response data response. Nonlinearity Biol Toxicol Med. 3:235–244.
- Mashela PW, De Waele D, Dube ZP, Khosa MC, Pofu KM, Tefu G, Daneel MS, Fourie H. 2016. Alternative nematode management strategies. In: Fourie H, Spaull VW, Jones RK, Daneel MS, De Waele D, editors. Nematology in South Africa: a view from the 21st century. Heidelberg (Switzerland): Springer International Publishing; p. 151–182.
- Mashela PW, Duncan L, Graham JM, McSorley R. 1992. Leaching soluble salts increases population densities of Tylenchulus semipenetrans. J Nematol. 24:103–108.
- Mphahlele RR, Mashela PW, Pofu KM. 2012. Post-harvest fruit decay-inducing pathogen in medicinally important Cucumis species indigenous to South Africa. Afr J Agric Res. 6:3786–3379.
- Perry RN. 1999. Desiccation survival of parasitic nematodes. Parasitology. 119:19–30. doi: 10.1017/S0031182099004436
- Salisbury FB, Ross CW. 2005. Plant physiology. Wadsworth: Belmont California.
- SAS Institute INC. 2008. SAS/STAT® 9.2 qualification tools user’s guide. Cary (NC): SAS Institute.
- Shadung KG, Mashela PW, Mphosi SM. 2016. Suitable drying temperature for preserving cucurbitacins in fruit of wild cucumber and wild watermelon. Hort Technol. 26:816–819.
- Sikora RA, Fernández E. 2005. Nematode parasites of vegetables. In: Luc M, Sikora RA, J. Bridge Cambridge, editors. Plant parasitic nematodes in subtropical and tropical agriculture. Wallingford (UK): CABI Publishing; p. 319–392.
- Steyn JM, Haverkort AJ, Franke AC, Engelbrecht FA, van der Waals JE, Kruger K. 2014. Impact of different climate change scenarios on future potato production in South Africa: eastern Free State, Limpopo and Sandveld. Potato South Africa, Final Report: Pretoria.
- Thies JA, Levi A. 2007. Characterisation of watermelon (Citrullus lanatus var. citroides) germplasm for resistance to root-knot nematodes. Hort Sci. 42:1530–1533.