ABSTRACT
Bacillus pumilus WP8 is a plant growth-promoting rhizobacterium (PGPR) with good biocontrol efficacy against tomato bacterial wilt caused by Ralstonia solanacearum Rs1115. Biocontrol, however, is not due to antagonism of the pathogenic bacterium. Thus, we hypothesised that the biocontrol efficacy of WP8 was achieved by attenuation of Rs1115 virulence. Here, pot experiments for comparison of Rs1115 in different plant parts were conducted to investigate the ability of WP8 to prevent entry of Rs1115 into the regions of the plant above ground. Primary and secondary metabolite contents of WP8 and their inhibitory effects on twitching and swarming motilities of Rs1115 were determined by microscopic examination and crystal violet staining. The effects of WP8 metabolites on the expression of typical virulence genes in Rs1115 were established by quantitative PCR. Rs1115 abundance in the rhizosphere increased with time after inoculation. However, the shoots treated with WP8 were pathogen-free on days 3 and 6 after inoculation. In the WP8 + Rs1115 treatment group, the abundance of Rs1115 in shoots was 1.5 lg units higher on day 9 post-inoculation than that in the Rs1115 treatment group, while less Rs1115 was observed in the leaves. This indicated that WP8 prevented Rs1115 from spreading to the regions of the plant above ground. Furthermore, some heat-resistant secondary metabolites of WP8 (e.g. lipopeptides) inhibited the twitching and swarming motility of Rs1115. Moreover, the metabolites decreased the expression of typical virulence genes in Rs1115. Therefore, WP8 was shown to attenuate Rs1115 virulence, possibly through pumilacidin secretion.
Introduction
Solanum lycopersicum L., known as tomato, is one of the most popular fruits all over the world. In China, an area of over one million hectares is now used for tomato cultivation, and has become the world's main tomato exporting country. During the life cycle of the tomato, the plant may suffer from bacterial and fungal pathogens that cause significant economic losses. Ralstonia solanacearum, a soil-borne pathogen that causes tomato bacterial wilt (TBW), has attracted widespread concern due to its massive destruction of tomato plants (Hayward Citation1991). TBW is more severe in the tropical and warm-temperate regions that are the main tomato-producing areas, such as Sinkiang and Gansu Provinces in China. Therefore, effective strategies to control this pathogen are urgently required.
Plant growth-promoting rhizobacteria (PGPR) have been isolated and some have been successfully developed as commercial bio-agents to control this disease (Mahanty et al. Citation2017). Based on their mechanisms of action, these isolates can be divided into four groups: (i) those that exhibit antagonistic effects by secreting antibiotics and cell wall-degrading enzymes (Guo et al. Citation2004; Ramesh et al. Citation2009); (ii) those that attenuate virulence and pathogenicity genes of the pathogen (commonly Gram-negative bacteria) (Schell Citation2000) by quenching of quorum sensing (QS)-regulated production (Dong et al. Citation2000; Faure et al. Citation2009); (iii) non-pathogenic strains of R. solanacearum that can compete for space and niches (Frey et al. Citation1994; Etchebar et al. Citation1998); and (iv) those that induce systemic resistance against the pathogen (Deslandes et al. Citation2002). The diversity of PGPR with multiple mechanisms of action offers possibilities for the biological control of TBW. Among the group II PGPRs, very little information is available on the genes that may be associated with the attenuation process.
Bacillus pumilus WP8, a PGPR strain, was isolated from wheat rhizospheres (Kang et al. Citation2010). The growth-promoting and biocontrol effects of WP8 on multiple plants have been demonstrated (Shen et al. Citation2012; Kang et al. Citation2013; Kang et al. Citation2014). In a previous study, WP8 exhibited good biocontrol efficacy against TBW, although this effect was not achieved by antagonism of the pathogenic bacterium (Kang et al. Citation2012). We hypothesised that the biocontrol efficacy of WP8 was achieved by attenuation of Rs1115 virulence. In this study, a set of experiments were conducted to evaluate the efficacy of WP8 in attenuating the Rs1115 virulence. Also, the secondary metabolites that may be responsible for this process were investigated.
Materials and methods
Bacterial strains and culture conditions
B. pumilus WP8 was cultured in Luria–Bertani (LB) broth at 28°C for 12 h or 48 h for the subsequent extraction of primary or secondary metabolites. R. solanacearum 1115 (race 1, biovar 3), a pathogenic strain of TBW donated by Nanjing Agricultural University (China), was cultured in casamino acids/peptone/glucose (CPG) medium (0.1% casamino acids, 1% peptone, and 0.5% glucose) at 28°C for molecular experiments (Addy et al. Citation2012).
For preparation of inoculation solutions, WP8 and Rs1115 were cultured separately in LB broth for 24 h, and the culture solutions were centrifuged at 5000 rpm for 10 min. The resulting pellets were resuspended in sterile distilled water and adjusted to 109 cfu/mL.
In vitro antagonism assay for B. pumilus WP8 and R. solanacearum 1115
The dual culture method (Fokkema Citation2010) was employed to test the antagonistic effects of B. pumilus WP8 against R. solanacearum 1115. Briefly, R. solanacearum 1115 was cultured in LB broth for 18 h before centrifugation at 5000 rpm for 10 min. The resulting pellet was resuspended in sterile distilled water and 100 μL was then spread onto another LB agar plate containing a loopful of B. pumilus WP8 in the centre. After 48 h of culture at 28°C, the plate was examined for the presence of an inhibition zone surrounding the bacterial lawn of WP8. This experiment replicated on three independent occasions.
Primary or secondary metabolites of WP8 were prepared according to the previously described method. These metabolites were then sterilised using two different methods; filtration through 0.22 μm filters (referred to as WP8-12F or WP8-48F) and autoclaving at 121°C for 15 min (referred to as WP8-12A or WP8-48A), respectively. The resulting solutions were added separately to conical flasks containing 50 mL of LB broth at final concentrations of 20%. Each flask was then inoculated with 0.5 mL of R. solanacearum 1115 suspension. From 0 h to 28 h, absorbance at 600 nm (OD600) of each culture was recorded. Treatment without metabolite supplementation was conducted simultaneously as a control.
Biocontrol of tomato bacterial wilt (TWB) by B. pumilus WP8
In total, 60 young tomato seedlings (Fendu 110, Meiyamei Seeds, China) at the 4–5 leaf stage were transplanted into pots (26 cm height × 25 cm internal diameter) containing 8.5 kg of sandy loam soil. The soil was previously autoclaved at 121°C for 1 h and was repeated two times. The characteristics of the soil were pH 7.6, organic matter 3.83 g/kg, total N 1.49 g/kg, available N 19.44 mg/kg, P 7.66 mg/kg, and K 2.25 mg/kg. Before transplanting, the roots of seedlings were soaked for 30 min to give three treatment groups: blank control (no inoculation), Rs1115 only, and Rs1115+WP8. In the Rs1115 + WP8 group, seedlings were soaked for 30 min in WP8 suspension at a final concentration of 109 cfu/mL, while the remaining seedlings were simultaneously soaked for 30 min in sterile water as a control. After 3 days of adaptation, soil near the roots of each pot in the Rs115 only and Rs1115 + WP group was drenched with 100 mL of Rs1115 suspension. After 20 days of growth in a greenhouse at 25°C day/20°C night, plant growth indicators (plant height, root length, aboveground and root dry weight) were measured using routine methods. Healthy plants were also evaluated and biocontrol efficiency (%) was calculated as follows: (numbers of diseased plants in the Rs1115 treatment group – numbers of diseased plants in Rs1115 plus WP8 treatment group)/numbers of diseased plants in Rs1115 only treatment group × 100%.
Effects of B. pumilus WP8 on the distribution of R. solanacearum 1115 in tomato plants
Based on the previously described experiment, five plants in each group were sampled on days 3, 6, and 9 post-inoculation with pathogenic bacteria. For each treatment group, samples of the shoot (5 cm away from soil), leaf (a mixture of three leaves from the top, middle, and bottom of the plant) and rhizosphere soil were used for comparison of the colonisation of Rs1115.
The population of Rs1115 in the different parts of plants at each time-point was determined using the 8-spot plating method with some modifications (Harris and Sommers Citation1968). Briefly, before determination, plates with quartering markers (labelled 1–4) on the bottom were prepared with a dilution of Kelman's TTC (2,3,5 triphenyl tetrazolium chloride) (Kelman Citation1954) and stored at 4°C before use. For each plant, 1 g of tissue was mixed with 5 mL of phosphate buffer in a sterile sample bag. The samples were then macerated on ice with a wooden dowel to obtain a bright green solution, from which serial dilutions were prepared. Subsequently, each quarter of the agar plate was inoculated with equal amounts of eight bacterial solutions (10 μL each) using an automatic repeater pipet. After 2–3 days of incubation at 28°C, plates were removed for counting and calculation of the population.
Effects of B. pumilus WP8 metabolites on motility of R. solanacearum 1115
Three methods were adopted to assess the effects of metabolites of B. pumilus WP8 on twitching and swarming motility of R. solanacearum 1115. Twitching motility was determined according to Gallant's method with slight modifications (Gallant et al. Citation2005). Briefly, four bacterial suspensions of Rs1115 (20 μL each) were stab-inoculated into 1% LB agar plates (15 mL) with or without (blank control) metabolites of WP8 to a final concentration of 20%, followed by incubation at 28°C for 24 h. The agar was carefully removed and zones of bacteria spread were visualised by staining with 1% crystal violet for 10 min followed by gentle washing with tap water to remove unbound dye. To further confirm the twitching motility, 5 μL of Rs1115 suspension was spot-inoculated onto minimal medium (MM) plates with or without metabolites of WP8. MM medium contained (per litre) 10.0 g of glucose, 6.56 g of KH2PO4, 30.96 g of Na2HPO4, 0.41 g of MgSO4, 0.088 g of ferric citrate, 0.1 g of L-leucine, 0.1 g of L-isoleucine, 0.1 g of L-valine, 0.1 g of L-methionine, 0.1 g of L-cysteine, 0.6 g of L-glutamine, and 0.5 mg of riboflavin. After incubation for 48 h at 28°C, expansion of the colony perimeter was observed with a microscope (Addy et al. Citation2012). Swarming motility was evaluated by spot-inoculation of 10 μL of Rs1115 suspensions onto 0.5% MM semi-solid medium with or without metabolites of WP8. Plates were removed for observation at 24 h intervals during the period of incubation at 28°C (Kelman and Hruschka Citation1973).
Effects of B. pumilus WP8 metabolites on virulence gene expression in R. solanacearum 1115
Rs1115 was cultured in CPG medium with or without metabolites of WP8 at a final concentration of 20% at 28°C for 24 h on a rotary shaker at 200 rpm (Addy et al. Citation2012). Total bacterial RNA was isolated using an RNAprotect Bacteria Reagent kit (Qiagen, Germany) according to the manufacturer's protocol. Possible genomic DNA contaminants were removed by incubation with 10 U RNase-free DNase (Tiangen, Beijing, China) for 30 min at 37°C, followed by inactivation with phenol/chloroform extraction. A standard PCR was first performed with 30 cycles to generate cDNA in a T100TM thermal cycler (Bio-Rad Laboratories, Hercules, CA, USA) and quantitative real-time reverse transcriptase PCR (qRT-PCR) was then carried out in a CFX Connect TM Real-Time PCR System (Bio-Rad) with the cDNA derived from Rs1115. PCR reaction mixtures were prepared using the 10 µL of the SYBR® Premix Ex TaqTM II kit (Perfect Real-Time, Takara, Dalian, China), 2 μL of diluted cDNA, and 0.5 μM final concentration of each primer (Addy et al. Citation2012) and ddH2O to give a final reaction volume of 20 µL. The sequences of the primers for each target gene were as follows: transcriptional regulator coding gene for extracellular polysaccharide phcA (5’-GGACATGATCTTCACGGTCAACT-3’; 5’-GACTCATCCTCCTTTTCTGCATC-3’), QS signal synthase gene phcB (5’-CTACCAGATCGTCGTCAATGAA-3’; 5’-GTCGAGGTAGTGCTTGATCTTG-3’), endoglucanase coding gene egl (5’-CAGCGCGACCTACTACAAGA-3’; 5’-TCATCAGCCCGAAGATGAC-3’), polygalacturonases coding gene pehC (5’-GTTGTTCGGATTGCTGTACG-3’; 5’-AGTCAAACGATTGCCTGAACTA-3’), and pili-forming-related gene pilT (5’-AAGAACAAAGCGTCTGATCTGC-3’; 5’-CTTCCAGGTTTTCTTCGTAATGCT-3’). The thermocycling conditions were as follows: 30 s at 95°C followed by 50 cycles at 95°C for 5 s, and 60°C for 31 s. The specificity of each primer set was confirmed by melting curve analysis (65°C to 95°C with a heating gradient of 0.5°C/min). Relative expression levels were calculated as the ratio of expression of each gene versus that of the 16S rRNA gene (primer: 5’-CTAGAGTGTGTCAGAGGGAGGTAGA-3’; 5’-ATGTCAAGGGTAGGTAAGGTTTTTC-3’) in R. solanacearum. Cycle threshold (Ct) values were converted to relative gene expression levels using the 2 −ΔΔCT method.
Effects of B. pumilus WP8 on plant defense-related enzyme activity
On days 1, 3, and 5 post-inoculation with Rs1115, plant leaves were collected randomly from each treatment group, and peroxidase (POD) and phenylalanine ammonia-lyase (PAL) activities were determined (Qin and Tian Citation2005). One gram of each sample was homogenised at 4°C in 10 mL of 0.1 M sodium phosphate buffer (pH 6.4) containing 0.2 g of polyvinyl pyrrolidone (PVP). Subsequently, the homogenate was centrifuged at 4000 rpm for 25 min at 4°C, and the supernatant was used as a crude enzyme preparation for activity assays. For the POD activity assay, the reaction mixture consisted of 2 mL of crude enzyme, 1 mL of guaiacol as a substrate, and 1 mL of 50 mM sodium acetate buffer solution (pH 5.0) (Ippolito et al. Citation2000). One unit (U) of POD activity was defined as the change in absorbance at 460 nm (OD460) per minute per gram of protein.
For PAL crude enzyme extraction, 0.5 g of leaf was mixed with 2 mL of 0.1 M Tris-H2SO4 buffer (pH 8.8) containing 0.05% final concentration of β-mercaptoethanol, 0.03% EDTA, and 5% glycerol. After homogenisation on ice, the homogenate was centrifuged at 4000 rpm for 25 min at 4°C, and the supernatant was used as a crude enzyme preparation for activity assays. The reaction system consisted of 0.1 mL of crude extract, 1 mL of 0.01 M borate buffer (pH 8.8), 0.5 mL of 0.02 M phenylalanine, and 0.5 mL of ddH2O. The reaction system was incubated at 30°C for 30 min and the reaction was terminated by the addition of 0.1 mL of 6 M HCl (Cheng and Breen Citation1991). One unit (U) of PAL activity was defined as the change in absorbance at 290 nm (A290) per hour per gram of protein.
Data analysis
Raw data were imported into SPSS Statistics for Windows Version 24.0 (SPSS, IBM, Somers, NY, USA) for calculating means and standard errors (SE). Differences between treatment groups were analyzed with Duncan's multiple range test. P < 0.05 was considered to indicate statistical significance. All the figures presented in this article were obtained using Sigma Plot for Windows Version 10.0 (Systat Sofware, San Jose, CA, USA).
Results and Discussion
Antagonistic effects of B. pumilus WP8 and its metabolites on R. solanacearum 1115
Using the dual culture method, we demonstrated that WP8 was compatible with Rs1115 (). In contrast to our findings, it was reported that the growth and reproduction of R. solanacearum could be suppressed by Bacillus subtilis, Paenibacillus macerans, Pseudomonas fluorescens, Enterobacter sp., as well as B. pumilus (Naser et al. Citation2008; Ramesh et al. Citation2009). This discrepancy may be due to the differences in the isolates investigated. Furthermore, the primary and secondary metabolites also have no antagonistic effects against Rs1115 ().
Figure 1. Dual culture of Bacillus pumilus WP8 (referred to as WP8) and Ralstonia solanacearum Rs1115 (referred to as RS) showed that these two strains were compatible.
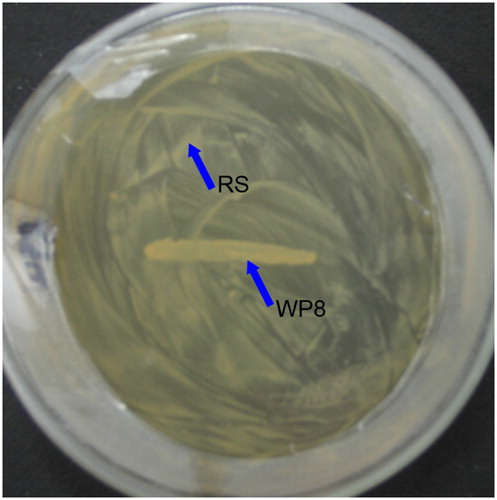
Figure 2. Growth curves of Ralstonia solanacearum Rs1115 in LB broth containing metabolites of Bacillus pumilus WP8 at final concentrations of 20%. 12A and 48A represent primary and secondary metabolites with autoclaving treatment; 12F and 48F represent primary and secondary metabolites with filtration treatment using 0.22 μm filters.
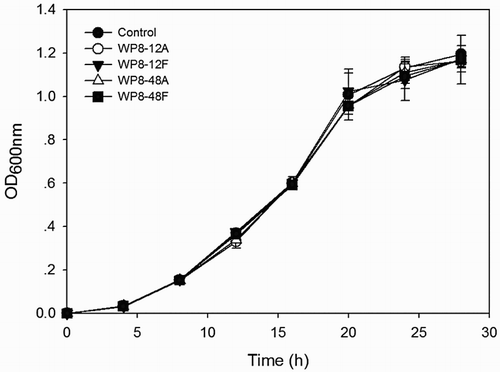
Effects of B. pumilus WP8 on distribution of Rs1115 in tomato plants
The pot experiment showed that WP8 exhibited both biocontrol and growth-promotion efficacy (), which was manifested as significant enhancement (P < 0.05) of root length and plant biomass rather than in plant height. This is in accordance with the results of our previous study (Shen et al. Citation2012). There were significantly fewer surviving plants treated with Rs1115 (n = 6) than in the control (n = 20) and WP8+Rs1115 (n = 18) treatment groups; however, there were no significant differences in the dry biomasses between the Rs1115 and control treatments. This suggested that the negative effect of the pathogen was reflected mainly in the lethality against tomato plants, but not in growth suppression of surviving plants, which may be due to the positive effects of pathogen-derived auxins, such as indoleacetic acid, (Valls et al. Citation2006) on plant growth. Nevertheless, these effects cannot be sustained long-term, with most studies showing that tomato yield decreases were accompanied by an increase in TBW incidence (Berga et al. Citation2001; Nguyen and Ranamukhaarachchi Citation2005). In the rhizosphere, the abundance of Rs1115 increased with time (), which indicated that Rs1115 is well adapted to the rhizoshpere environment. The similarity of the size of the Rs1115 populations of in the Rs1115 only and Rs1115+WP8 treatment groups confirmed that WP8 did not exhibit antagonistic effects against Rs1115 in the rhizosphere. However, there were no pathogens in the shoots of plants in the WP8+Rs1115 treatment group on days 3 and 6 post-inoculation with the pathogen. On day 9, the abundance of Rs1115 in shoots was 1.5 lg lower than that in the WP8+Rs1115 treatment group. Furthermore, Rs1115 was not detected in the leaves of plants in the WP8+Rs1115 treatment group. These data clearly indicate that B. pumilus WP8 prevents the transfer of Rs1115 into the regions of the plant above ground. This is in contrast to the study by Zhu and Yao (Citation2004), which showed that R. solanacearum could be suppressed by an arbuscular mycorrhizal (AM) fungus, an effect that was reflected in the decreases in abundance of pathogens in both the rhizosphere and in the xylem. Many studies have revealed that swimming motility and twitching motility contribute to bacterial wilt virulence in the early stages of host plant invasion and colonisation (Liu et al. Citation2001; Tans-Kersten et al. Citation2001). On reaching the plant xylem, the pathogen becomes non-motile (Tans-Kersten et al. Citation2004; Yoshimochi et al. Citation2009). Our results confirmed that B. pumilus WP8 exhibited biocontrol efficacy against TBW, probably by preventing the movement of Rs1115 into the regions of the plant above ground.
Figure 3. Effects of Bacillus pumilus WP8 on the population of Ralstonia solanacearum Rs1115 in tomato plants. Rs1115: soils were treated with a cell suspension of Rs1115 at a final concentration of 1010 cfu/kg of soil. Rs1115+WP8: plant roots were previously soaked for 30 min in WP8 suspension at a final concentration of 109 cfu/mL.
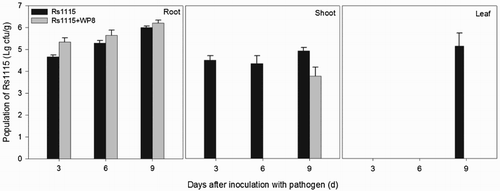
Table 1. Biocontrol of tomato bacterial wilt and growth-promoting effects mediated by Bacillus pumilus WP8.
Effects of B. pumilus WP8 metabolites on motility and virulence gene expression in Rs1115
To determine which of the metabolites secreted by WP8 were responsible for the inhibition process, primary and secondary metabolites of WP8 were prepared for evaluation of their effects on Rs1115 motility. Two types of metabolites exhibited inhibitory effects on Rs1115 swimming motility (, ). In addition, the inhibitory effects were more obvious in the 48F treatment group than those in the 12F treatment group, suggesting that the inhibitory effect was more likely to be mediated by secondary metabolites than by primary metabolites. Twitching experiments also showed that Rs1115 motility was inhibited by WP8 metabolite(s) ( and ; and ), which were probably heat-resistant (). Both WP8-48F and WP8-48A significantly decreased the expression of some typical virulence genes at the mRNA level (). These data indicate that some heat-resistant secondary metabolites of B. pumilus WP8 inhibit motility and the expression of certain typical Rs1115 virulence genes.
Figure 4. Time courses of swarming motility of Ralstonia solanacearum Rs1115 on 0.5% MM semi-solid medium with or without metabolites of WP8. 12F and 48F represent primary and secondary metabolites with filtration treatment using 0.22 μm filters.
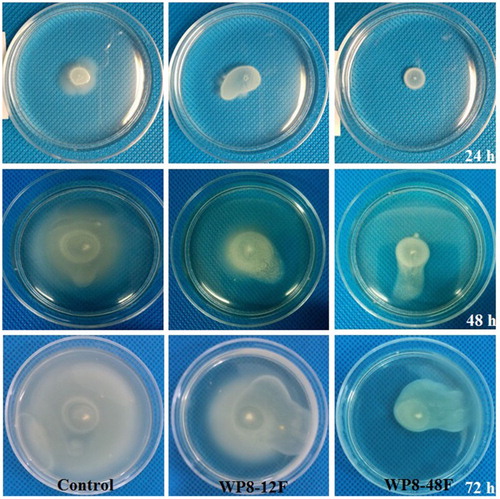
Figure 5. Effects of metabolites of Bacillus pumilus WP8 on twitching motility of Rs1115. 12A and 48A represent primary and secondary metabolites with autoclaving treatment; 12F and 48F represent primary and secondary metabolites with filtration treatment using 0.22 μm filters.
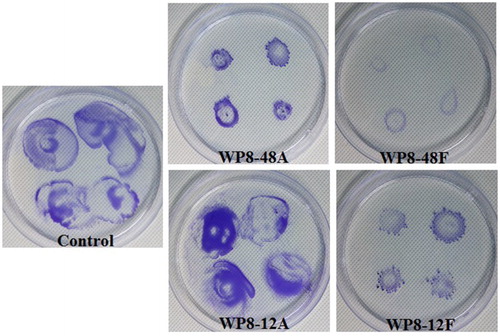
Figure 6. Time course of Ralstonia solanacearum Rs1115 spreading formed on MM plates. Different lengths of edges of colonies indicate distinct abilities to exhibit twitching motility.
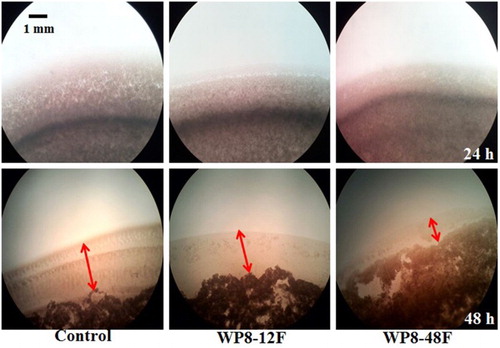
Figure 7. Expression analysis of Ralstonia solanacearum Rs1115 genes involved in virulence. Data represent the mean expression ± standard deviation of three independent experiments. Single asterisk (*) and double asterisks (**) indicate significant differential expression of genes versus controls at P < 0.05 and P < 0.01 respectively, according to the Duncan test.
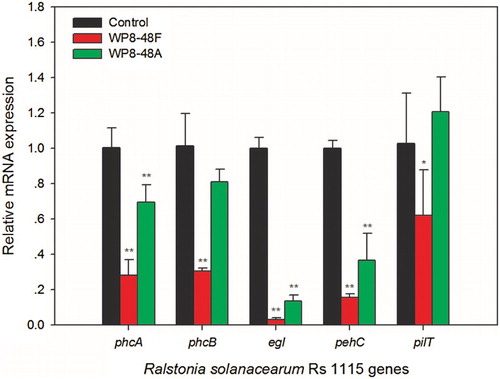
Table 2. Effects of metabolites of Bacillus pumilus WP8 on swimming motility of Ralstonia solanacearum Rs1115.
Table 3. Effects of metabolites of Bacillus pumilus WP8 on twitching motility of Ralstonia solanacearum Rs1115.
Table 4. Effects of metabolites of Bacillus pumilus WP8 on twitching motility of Ralstonia solanacearum Rs1115.
Similarly, Liu et al. reported that strains of B. cereus and B. sphaercus attenuated the pathogenicity of R. solanacearum (Liu et al. Citation2004). The difference is that WP8 inhibits the motility of Rs1115 by preventing the transport of Rs1115 into the regions of the plant above ground, especially the xylem (). However, the triggers of this process remain to be clarified, and there is little information on the mechanism by which B. pumilus mediates biocontrol of TBW (Hardoim et al. Citation2008). In the first study, we found that WP8 produced a siderophore. However, this may not be the factor that causes the attenuation effect because siderophores often play a role in growth inhibition in eukaryotic pathogens by competition for iron (Pal et al. Citation2001). It can be speculated that pumilacidin, a heat-resistant metabolite of P. pumilus, is responsible for the attenuation effect for two reasons: i) the biocontrol effect of pumilacidin has been reported, and its homolog maintained activity after treatment for 2 h at 100°C (Pathak and Keharia Citation2014); ii) pumilacidin exhibits inhibitory effects on some viruses, but not on many bacteria or fungi (Karuse et al. Citation1990). Apart from the attenuation process, WP8 could also increases in the activity of POD and PAL (), which are enzymes are involved in defense reactions against pathogens in plants, and typical indicators of induced systemic resistance (ISR) mediated by PGPRs (Hsa et al. Citation2004). Our data indicate that the biocontrol of TBW by WP8 is achieved via integration of multiple pathways rather than a single mechanism. We also showed that Rs1115 colonies grown on the culture media containing metabolites of WP8 were not non-virulent strains (data not shown). This suggested that treatment of soil with WP8 may allow the recovery of the pathogenicity of a non-virulent pathogen; however, this hypothesis requires more detailed investigation.
Figure 8. Time course of two typical defense enzymes activities [peroxidase (POD) and phenylalanine ammonia-lyase (PAL)].
![Figure 8. Time course of two typical defense enzymes activities [peroxidase (POD) and phenylalanine ammonia-lyase (PAL)].](/cms/asset/ad72e773-68b3-4dea-8a14-ae414e9fa6c6/sagb_a_1416663_f0008_b.gif)
In conclusion, we found that the biocontrol efficacy of WP8 against Rs1115 was not achieved by growth suppression, but by attenuation of pathogenic virulence. Furthermore, our findings indicate that WP8 attenuates Rs1115 virulence through pumilacidin secretion.
Disclosure statement
No potential conflict of interest was reported by the authors.
Notes on contributors
Min Shen is a lecturer at College of Marine and Bio-engineering,Yancheng Teachers University, Yancheng, Jiangsu, PR China,and her major is Environmental Microbiology.
Dan Xia is a postgraduate student for master degree at College of Marine and Bio-engineering,Yancheng Teachers University, Yancheng, Jiangsu, PR China, and her is Environmental Microbiology.
Zhifeng Yin is a postgraduate student for master degree at Environmental Science & Engineering, Yangzhou University, Yangzhou,Jiangsu, PR China, and her major is Soil Microbiology.
Qingxin Zhao is a Professor at College of Marine and Bio-engineering, Yancheng Teachers University, Yancheng, Jiangsu, PR China, and his major is Agricultural/Environmental Microbiology.
Yijun Kang is a associate Professor at College of Marine and Bio-engineering, Yancheng Teachers University, Yancheng,Jiangsu, PR China, whose research interest focus on Agricultural/Environmental Microbiology.
Additional information
Funding
References
- Addy HS, Askora A, Kawasaki T, Fujie M, Yamada T. 2012. The filamentous phage φRSS1 enhances virulence of phytopathogenic Ralstonia solanacearum on tomato. Phytopathology. 102:244–251. doi: 10.1094/PHYTO-10-11-0277
- Berga L, Siriri D, Ebanyat P. 2001. Effect of soil amendments on bacterial wilt incidence and yield of potatoes in southwestern Uganda. Afr Crop Sci J. 9:267–278.
- Cheng GW, Breen PJ. 1991. Activity of phenylalanine ammonia-lyase (PAL) and concentrations of anthocyanins and phenolics in developing strawberry fruit. J Am Soc Hortic Sci. 116:865–869.
- Deslandes L, Olivier J, Theulieres F, Hirsch J, Feng DX, Bittner-Eddy P, Beynon J, Marco Y. 2002. Resistance to Ralstonia solanacearum in Arabidopsis thaliana is conferred by the recessive RRS1-R gene, a member of a novel family of resistance genes. P Natl Acad SCI USA. 99:2404–2409. doi: 10.1073/pnas.032485099
- Dong YH, Xu JL, Li XZ, Zhang LH. 2000. Aiia, an enzyme that inactivates the acylhomoserine lactone quorum-sensing signal and attenuates the virulence of Erwinia carotovora. Proc Nat Acad Sci USA. 97:3526–3531. doi: 10.1073/pnas.97.7.3526
- Etchebar C, Trigalet-Demery D, Gijsegem F, Vasse J, Trigalet A. 1998. Xylem colonization by an HrcV– Mutant of Ralstonia solanacearum is a key factor for the efficient biological control of tomato bacterial wilt. Mol Plant Microbe In. 11:869–877. doi: 10.1094/MPMI.1998.11.9.869
- Faure D, Vereecke D, Leveau JHJ. 2009. Molecular communication in the rhizosphere. Plant Soil. 321:279–303. doi: 10.1007/s11104-008-9839-2
- Fokkema NJ. 2010. Fungal antagonisms in the phyllosphere. Ann Appl Biol. 89:115–119. doi: 10.1111/j.1744-7348.1978.tb02582.x
- Frey P, Prior P, Marie C, Kotoujansky A, Trigalet-Demery D, Trigalet A. 1994. Hrp- mutants of Pseudomonas solanacearum as potential biocontrol agents of tomato bacterial wilt. Appl Environ Microbiol. 60:3175–3181.
- Gallant CV, Daniels C, Leung JM, Ghosh AS, Young KD, Kotra LP, Burrows LL. 2005. Common beta-lactamases inhibit bacterial biofilm formation. Mol Microbiol. 58:1012–1024. doi: 10.1111/j.1365-2958.2005.04892.x
- Guo J-H, Qi H-Y, Guo Y-H, Ge H-L, Gong L-Y, Zhang L-X, Sun P-H. 2004. Biocontrol of tomato wilt by plant growth-promoting rhizobacteria. Biol Control. 29:66–72. doi: 10.1016/S1049-9644(03)00124-5
- Hardoim PR, van Overbeek LS, van Elsas JD. 2008. Properties of bacterial endophytes and their proposed role in plant growth. Trends Microbiol. 16:463–471. doi: 10.1016/j.tim.2008.07.008
- Harris RF, Sommers LE. 1968. Plate-dilution frequency technique for assay of microbial ecology. Appl Microbiol. 16:330–334.
- Hayward AC. 1991. Biology and epidemiology of bacterial wilt caused by Pseudomonas solanacearum. Annu Rev Phytopathol. 29:65–87. doi: 10.1146/annurev.py.29.090191.000433
- Hsa S, Romeiro RD, Halfeld-Vieira BD, Mcb P. 2004. Rhizobacterial induction of systemic resistance in tomato plants: non-specific protection and increase in enzyme activities. Biol Control. 29:288–295. doi: 10.1016/S1049-9644(03)00163-4
- Ippolito A, Elghaouth A, Wilson CL, Wisniewski M. 2000. Control of postharvest decay of apple fruit by Aureobasidium pullulans and induction of defense responses. Postharvest Biol Tec. 19:265–272. doi: 10.1016/S0925-5214(00)00104-6
- Kang Y, Cheng J, Mei L, Yin S. 2010. Screening and identification of plant growth-promoting rhizobacteria. Acta Microbiol Sin. 50:853–861.
- Kang Y, Shen M, Wang H, Zhao Q. 2013. A possible mechanism of action of plant growth-promoting rhizobacteria (PGPR) strain Bacillus pumilus WP8 via regulation of soil bacterial community structure. J Gen Appl Microbiol. 59:267–277. doi: 10.2323/jgam.59.267
- Kang Y, Shen M, Wang H, Zhao Q, Yin S. 2012. Biological control of tomato bacterial wilt caused by Ralstonia solanacearum with Erwinia persicinus RA2 and Bacillus pumilus WP8. Chin J Biol Control. 28:255–261.
- Kang Y, Shen M, Yang X, Cheng D, Zhao Q. 2014. A plant growth-promoting rhizobacteria (PGPR) mixture does not display synergistic effects, likely by biofilm but not growth inhibition. Microbiology. 83:666–673. doi: 10.1134/S0026261714050166
- Karuse N, Tenyo O, Kobaru S, Kamei H, Miyaki T, Konishi M, Oki T. 1990. Pumilacidin, a complex of new antiviral antibiotics. Production, isolation, chemical properties, structure and biological activity. J Antibiot. 43:267–280. doi: 10.7164/antibiotics.43.267
- Kelman A. 1954. The relationship of pathogenicity of Pseudomonas solanacearum to colony appearance in a tetrazolium medium. Phytopathology. 64:693–695.
- Kelman A, Hruschka J. 1973. The role of motility and aerotaxis in the selective increase of avirulent bacteria in still broth cultures of Pseudomonas solanacearum. J Gen Microbiol. 76:177–188. doi: 10.1099/00221287-76-1-177
- Liu H, Kang Y, Genin S, Schell MA, Denny TP. 2001. Twitching motility of Ralstonia solanacearum requires a type IV pilus system. Microbiology. 147:3215–3229 doi: 10.1099/00221287-147-12-3215
- Liu B, Lin Y, Zhu Y, Ge C, Cao Y. 2004. Attenuation characteristics of bacterial-wilt-disease biocontrol strain anti-8098A (Bacillus cereus) to Ralstonia solanacearum. J Agric Biotechnol. 12:322–329.
- Mahanty T, Bhattacharjee S, Goswami M, Bhattacharyya P, Das B, Ghosh A, Tribedi P. 2017. Biofertilizers: a potential approach for sustainable agriculture development. Environ Sci Pollut Res. 24:3315–3335. doi: 10.1007/s11356-016-8104-0
- Naser A, Chemeda F, Yaynu H. 2008. Evaluation of rhizosphere bacterial antagonists for their potential to bioprotect potato (Solanum tuberosum) against bacterial wilt (Ralstonia solanacearum). Biol Control. 47:282–288. doi: 10.1016/j.biocontrol.2008.09.003
- Nguyen MT, Ranamukhaarachchi SL. 2005. Soil-borne antagonists for biological control of bacterial wilt disease caused by Ralstonia solanacearum in tomato and pepper. J Plant Pathol. 92:395–406.
- Pal KK, Tilak KV, Saxena AK, Dey R, Singh CS. 2001. Suppression of maize root diseases caused by Macrophomina phaseolina, Fusarium moniliforme and Fusarium graminearum by plant growth promoting rhizobacteria. Microbiol Res. 156:209–223. doi: 10.1078/0944-5013-00103
- Pathak KV, Keharia H. 2014. Application of extracellular lipopeptide biosurfactant produced by endophytic Bacillus subtilis K1 isolated from aerial roots of banyan (Ficus benghalensis) in microbially enhanced oil recovery (MEOR). 3 Biotech. 4:41–48. doi: 10.1007/s13205-013-0119-3
- Qin GZ, Tian SP. 2005. Enhancement of biocontrol activity of Cryptococcus laurentii by silicon and the possible mechanisms involved. Phytopathology. 95:69–75. doi: 10.1094/PHYTO-95-0069
- Ramesh R, Joshi AA, Ghanekar MP. 2009. Pseudomonads: major antagonistic endophytic bacteria to suppress bacterial wilt pathogen, Ralstonia solanacearum in the eggplant (Solanum melongena L.). World J Microbiol Biotechnol. 25:47–55. doi: 10.1007/s11274-008-9859-3
- Schell MA. 2000. Control of virulence and pathogenicity genes of Ralstonia solanacearum by an elaborate sensory network. Ann Rev Phytopathol. 38:263–292. doi: 10.1146/annurev.phyto.38.1.263
- Shen M, Kang Y, Wang H, Zhang X, Zhao Q. 2012. Effect of plant growth-promoting rhizobacteria (PGPRs) on plant growth, yield, and quality of tomato (Lycopersicon esculentum Mill.) under simulated seawater irrigation. J Gen Appl Microbiol. 58:253–262. doi: 10.2323/jgam.58.253
- Tans-Kersten J, Brown D, Allen C. 2004. Swimming motility, a virulence trait of Ralstonia solanacearum, is regulated by FlhDC and the plant host environment. Mol Plant Microbe In. 17:686–695. doi: 10.1094/MPMI.2004.17.6.686
- Tans-Kersten J, Huang H, Allen C. 2001. Ralstonia solanacearum needs motility for invasive virulence on tomato. J Bacteriol. 183:3597–3605. doi: 10.1128/JB.183.12.3597-3605.2001
- Valls M, Genin S, Boucher C. 2006. Integrated regulation of the type III secretion system and other virulence determinants in Ralstonia solanacearum. PLoS Pathog. 2:e82. doi: 10.1371/journal.ppat.0020082
- Yoshimochi T, Hikichi Y, Kiba A, Ohnishi K. 2009. The global virulence regulator PhcA negatively controls the Ralstonia solanacearum hrp regulatory cascade by repressing expression of the PrhIR signaling proteins. J Bacteriol. 191:3424–3428. doi: 10.1128/JB.01113-08
- Zhu HH, Yao Q. 2004. Localized and systemic increase of phenols in tomato roots induced by Glomus versiforme inhibits Ralstonia solanacearum. J Phytopathol. 152:537–542. doi: 10.1111/j.1439-0434.2004.00892.x